Summary of Key Points
- •
Radiation-induced brachial plexopathy (RIBP) occurs with treatment of apical tumors and is frequently complicated by tumor-related brachial plexopathy (TRBP). Stereotactic ablative radiotherapy (SABR) of apical tumors, which employs a higher dose per fraction, can cause RIBP as well.
- •
RIBP symptoms include upper extremity paresthesias, motor weakness, muscle atrophy, and neuropathic pain. The peak incidence is 1–2 years, and the onset is often insidious over months to years.
- •
The most common side effect of radiation to the spinal cord is Lhermitte sign, which is caused by reversible demyelination of the ascending sensory neurons. Lhermitte sign is a shock-like sensation in the spine and extremities exacerbated by neck flexion, almost always symmetrical, and not associated with a dermatomal distribution. Radiation-induced Lhermitte sign begins 3 months and subsides within 6 months of the completion of radiotherapy.
- •
Radiation myelopathy can be devastating, and the clinical presentation depends on the level of the spinal cord affected. In general it begins with paresthesia and muscle weakness, and as the syndrome progresses gait disturbance and paraparesis appear. Radiation myelopathy is a diagnosis of exclusion, and patients must be evaluated for tumor progression and paraneoplastic syndromes with magnetic resonance imaging (MRI) of the cord.
- •
Assessment of the neurotoxic effects of radiation therapy can be confounded by the impact of brain metastases on neurologic function. Long-term outcome data are limited as a result of the short survival, and pretreatment and posttreatment neurologic testing has not been routine.
- •
Chemotherapy induced peripheral neuropathy (CIPN) is associated with taxanes (e.g., paclitaxel, docetaxel, nanoparticle albumin-bound paclitaxel), platinum agents (e.g., cisplatin and carboplatin), and vinca alkaloids (vinorelbine). Neuropathy associated with microtubule-targeting agents (vinca alkaloids and taxanes) is dependent on the length of the nerves, and patients frequently present with numbness and paresthesias of the feet and fingertips.
- •
The rate and severity of CIPN depends on the dose, duration and combination of chemotherapy agents used. Patients with a history of nerve damage from diabetes, alcohol use, and inherited neuropathy are at increased risk for the development of CIPN.
Chemotherapy and radiotherapy are routinely used for the treatment of nonsmall cell lung cancer (NSCLC) and small cell lung cancer (SCLC). Both types of treatment can cause acute and chronic neurotoxicities, which may affect the health-related quality of life of the patient and his or her ability to tolerate therapy. Management of the toxicities varies depending on the patient’s prognosis. In the palliative setting, acute toxicities may result in dose reduction, treatment delay, or treatment discontinuation, thus offsetting the potential benefits of palliative treatment. In the potentially curative setting, chronic treatment-related toxicities may be more clinically relevant. Unfortunately, the assessment of acute neurotoxicities has been variable, and the prospective collection of data on chronic neurotoxicities has been limited. Selected neurotoxicities are addressed in the National Cancer Institute (NCI) Common Terminology Criteria for Adverse Events (CTCAE) version 4.0 ( Table 43.1 ); however, many of these toxicities are based on physician assessment, and the determination of a grade 2 or grade 3 toxicity can be patient- and/or physician-dependent. When neurotoxicities do develop, management is often based on the patient’s symptoms. Current research is investigating methods of identifying patients who are at increased risk for neurotoxicity, as well as prevention strategies and improved treatment options.
Adverse Event | Grade 1 | Grade 2 | Grade 3 | Grade 4 |
---|---|---|---|---|
Brachial plexopathy | Asymptomatic; clinical or diagnostic observations only; intervention not indicated | Moderate symptoms, limiting instrumental ADL | Severe symptoms; limiting self-care ADL | NA |
Cognitive disturbance | Mild cognitive disability; not interfering with work, school, life performance; specialized educational services, devices not indicated | Moderate cognitive disability; interfering with work, school, life performance but capable of independent living; specialized resources on part-time basis indicated | Severe cognitive disability; significant impairment of work, school, life performance | NA |
Concentration impairment | Mild inattention or decreased level of concentration | Moderate impairment in attention or decreased level of concentration; limiting instrumental ADL | Severe impairment in attention or decreased level of concentration; limiting self-care ADL | NA |
Memory impairment | Mild memory impairment | Moderate memory impairment; limiting instrumental ADL | Severe memory impairment; limiting self-care ADL | NA |
Neuralgia | Mild pain | Moderate pain; limiting instrumental ADL | Severe pain; limiting self-care | NA |
Paresthesia | Mild symptoms | Moderate symptoms; limiting instrumental ADL | Severe symptoms; limiting self-ADL | NA |
Peripheral motor neuropathy | Asymptomatic; clinical or diagnostic observations only; intervention not indicated | Moderate symptoms; limiting instrumental ADL | Severe symptoms; limiting self-care ADL; assistive device indicated | Life-threatening consequences; urgent intervention indicated |
Peripheral sensory neuropathy | Asymptomatic; loss of deep tendon reflexes or paresthesia | Asymptomatic; loss of deep tendon reflexes or paresthesia | Severe symptoms; limiting self-care ADL | Life-threatening consequences; urgent intervention indicated |
Neurotoxicity from Radiotherapy
Neurotoxic effects of radiotherapy for lung cancer, predominantly to the brachial plexus, spinal cord, and brain, are important in the curative and palliative setting. Understanding the neurotoxic effects of radiotherapy is increasingly important, as patients with lung cancer are living longer and radiotherapy techniques are evolving. Intensity-modulated radiotherapy (IMRT) and image-guided radiotherapy (IGRT) allow for delivery of increasing total doses of radiation to the tumor while potentially resulting in a high total dose of radiation to small volumes of normal tissues and inhomogeneous doses across large volumes. SABR is a special consideration because it can potentially result in a very high dose per fraction to small volumes of the lung. As patients are living longer with more aggressive local treatment and more effective systemic therapy, the effects of late toxicity are more likely. It is imperative that current and future studies and clinical practice include long-term follow-up with appropriate documentation of dose, grading, and resulting toxicity.
Brachial Plexus
Data regarding RIBP in patients with lung cancer are limited because of the perceived lack of clinical significance. High-dose radiation to the brachial plexus is limited to cases of apical tumors and is frequently complicated by TRBP. However, RIBP may increase in incidence because of improved therapy for advanced lung cancer and treatment of earlier-stage lung cancer, with SABR resulting in longer survival. The risk of RIBP is associated with increased radiation dose and higher dose per fraction, as well as with the volume of the brachial plexus treated and the concomitant use of chemotherapy. Appropriate radiation dose to the brachial plexus and acceptable risk of RIBP varies depending on the stage of disease and intent of therapy. In many cases, avoiding high radiation dose to the brachial plexus results in undertreating the tumor. When disease is potentially curable or long-term survival is anticipated, the high risk of RIBP may be unavoidable and an understanding of the risks is important for counseling patients.
The diagnosis of RIBP is often complicated by tumor involvement, surgery, and/or unrelated trauma or injury. Symptoms include upper extremity paresthesias, motor weakness, muscle atrophy, and neuropathic pain. The latency period for the onset of symptoms can be a few months to as many as 20 years; the peak incidence is around 1 to 2 years. The onset of RIBP is often insidious, occurring over 6 months to as long as 5 years and progressing in intensity, eventually resulting in paralysis of the upper extremity. RIBP is almost always chronic and progressive, although there are rare reports of early transient RIBP. Symptoms reported and attributed to early transient RIBP include pain, paresthesias, and weakness occurring in 2 to 14 months following therapy with regression and often complete resolution of symptoms. MRI and/or computed tomography (CT) are important diagnostic tools to rule out progressive or metastatic disease. Electromyography can be used to support a diagnosis of RIBP.
Most information regarding the effects of radiation on the brachial plexus is from the literature on breast cancer. The brachial plexus is at least partially treated in almost all cases of breast or chest wall radiotherapy and is frequently involved in regions of matching fields, leading to high doses as a result of unintended field overlap. In addition, patients who receive radiotherapy for breast cancer tend to have relatively long follow-up, increasing the likelihood that late reactions will manifest. Over the past 50 years, different radiation techniques have been used to treat breast cancer, resulting in varying incidences of RIBP. In the 1950s and 1960s, RIBP was diagnosed in more than 50% of patients treated with 50 Gy to 60 Gy at 5 Gy per fraction; currently, RIBP develops in less than 1% to 2% of patients treated with less than 55 Gy at 1.8 Gy to 2 Gy per fraction.
RIBP in patients with head and neck cancer is an increasing area of interest because of the use of IMRT for treatment of the disease. In an attempt to restrict radiation dose to organs at risk while maximizing dose to treatment target volumes with the use of IMRT, there is a relatively inhomogeneous dose distribution, and the implications may not be completely understood. If organs at risk are not appropriately contoured, these hot spots could be inadvertently in a high-risk area. Proper anatomic definition of the brachial plexus is necessary for understanding potential side effects and complying with dose–volume constraints. The development of a brachial plexus contouring atlas by the Radiation Therapy Oncology Group (RTOG) has facilitated and encouraged the consistent and routine evaluation and reporting of radiation effects to the brachial plexus in the treatment of head and neck cancers. RTOG guidelines recommend a maximum dose of 60 Gy to 66 Gy or less to the brachial plexus. Truong et al. retrospectively contoured and reviewed doses to the brachial plexus in 114 patients treated with IMRT for head and neck cancer with 69.3 Gy in 33 fractions. There were no reports of RIBP, despite a maximum dose of more than 66 Gy to the brachial plexus in 20% of patients, with a median follow-up of 16.2 months. Longer follow-up is needed to assess the true incidence of RIBP in patients with head and neck cancer. Chen et al. prospectively evaluated the incidence of clinically significant peripheral neuropathies in patients undergoing radiotherapy for head and neck malignancies and reported an incidence of 12% for all patients and 22% for patients who were followed for more than 5 years. The investigators also suggested that RIBP symptoms in patients with head and neck cancer are underreported. Their data suggested a threshold dose of more than 70 Gy to the brachial plexus, although RIBP was also reported in some patients treated with doses less than 60 Gy, suggesting other contributing factors. Prior neck dissection and higher maximum dose of radiotherapy were associated with an increased risk of RIBP.
Eblan et al. evaluated RIBP in 80 patients treated to 50 Gy or more of conventionally fractionated radiotherapy for apical NSCLC; the median follow-up was 17.2 months. RIBP developed in five patients, which was more common among patients who had prior TRBP. The 3-year competing risk-adjusted rate of RIBP was 12%, whereas the 3-year estimated rate of TRBP as a result of treatment failure was 13%. The median onset of TRBP was 4 months compared with 11 months for RIBP, and symptom severity was greater for patients in whom TRBP developed. RIBP did not develop in any of the patients who received less than a maximum dose of 78 Gy to the brachial plexus, and for patients in whom RIBP did develop, considerable volumes were irradiated to doses above 66 Gy. Amini et al. identified 90 patients treated with definitive radiotherapy and concurrent chemotherapy and more than 55 Gy to the brachial plexus. The median dose to the brachial plexus was 70 Gy and the median follow-up was only 14 months; grade 1 to grade 3 RIBP developed in 16% of patients. The median time to symptoms was 6.5 months. Independent predictors of RIBP were a median dose to the brachial plexus of more than 69 Gy, a maximum dose of more than 75 Gy to 2 cm, and the presence of plexopathy before radiation.
The brachial plexus receives a considerably higher dose per fraction with SABR and therefore is susceptible to greater risk of late complications. Maximum dose of SABR, as well as dose–volume tolerance and clinical presentation with high dose per fraction, is uncertain. Forquer et al. evaluated the risk of brachial plexopathy in 37 lesions treated with SABR for apical lung tumors; RIBP developed in 7 of 37 patients treated. Five patients had neuropathic pain alone, one patient had pain and weakness, and one patient had pain, numbness, and paralysis of the hand and wrist. At a median follow-up of 7 months, the absolute risk of RIBP was 32% with a dose to the brachial plexus of more than 26 Gy and 6% with a maximum dose of 26 Gy or less in three to four fractions. The median time to development of RIBP was 13 months. In contrast to RIBP reported in other series, symptoms improved in six of seven patients over 3 to 10 months, including improvement of neuropathic pain. One patient who had received a maximum dose of 76 Gy to the brachial plexus had onset of pain and tingling at 9 months of follow-up, with progression to muscle wasting and weakness at 42 months.
Consistent contouring and dose–volume analyses in symptom reporting in the literature will continue to improve the clinical understanding of radiation tolerance of the brachial plexus. Currently, for apical lung tumors adjacent to or contiguous with the brachial plexus, restricting the dose to the brachial plexus may not be possible without compromising tumor control. A better understanding of dose–volume constraints and symptoms will assist in determining the risk of RIBP and proper patient counseling.
Spinal Cord
Radiation myelitis is a rare complication of radiotherapy for lung cancer because, in most cases, the spinal cord can be avoided without compromising disease coverage. This avoidance is particularly true for lung cancer in an era of smaller treatment fields directed at gross disease, three-dimensional conformal radiotherapy planning, IMRT, and IGRT. IMRT plans can shape the high-dose lines around the cord, and with IGRT, high doses adjacent to the spinal cord can be delivered with relative confidence that the set-up is reproducible and accurate.
The most common side effect of radiation to the spinal cord is Lhermitte sign, which is caused by reversible demyelination of the ascending sensory neurons as a result of inhibition of oligodendrocyte proliferation. Lhermitte sign was first described in relation to injury to the cervical spinal cord; is associated with other demyelinating disorders, including multiple sclerosis; and can be induced by radiotherapy or chemotherapy. Lhermitte sign is a shock-like sensation in the spine and extremities exacerbated by neck flexion; it is almost always symmetrical, is not associated with a finite dermatomal distribution, and is transient, subsiding with oligodendrocyte recovery and remyelination. Radiation-induced Lhermitte sign begins at about 3 months and subsides within 6 months of the completion of radiotherapy. The incidence of Lhermitte sign is reported to be between 3.6% and 13% in large patient groups receiving radiotherapy for head and neck and thoracic malignancies. Risk factors associated with the development of Lhermitte sign are total radiation doses above 50 Gy to the cervical spinal cord and daily radiation fraction doses above 2 Gy. Pak et al. found a relatively high incidence of Lhermitte sign (21%) with IMRT for head and neck cancer and concurrent chemotherapy. The strongest predictors of Lhermitte sign were higher percentage and cord volumes receiving 40 Gy or more. The investigators suggested that the higher incidence of Lhermitte sign in their series might have been related to higher reporting in a prospective setting and the chemotherapeutic agents. Lhermitte sign appearing in the context of transient radiation myelopathy is not associated with chronic progressive myelitis; however, delayed radiation myelopathy, which is irreversible and results in paralysis, may be preceded by Lhermitte sign. Lhermitte sign that predates delayed radiation myelopathy is found later in onset than the usual latency period of Lhermitte sign found in transient radiation myelopathy.
Because delayed radiation myelopathy can be a devastating side effect, radiation oncologists take every precaution to avoid it. Although glial cells and vascular endothelium are proposed to be the main targets for radiation and play a role in the pathogenesis of radiation myelopathy, experimental data support that radiation-induced vascular damage resulting in vascular hyperpermeability and venous exudation is a basic process. The clinical presentation of radiation myelopathy depends on the area of the affected spinal cord and the extent of the lesion. Generally, paresthesia and muscle weakness, which begins in the legs, are the main early symptoms. As the lesion progresses, various symptoms present, such as gait disturbance and paraparesis. Schultheiss and Stephens emphasized that radiation myelopathy is a diagnosis of exclusion, and patients should be evaluated for tumor progression and paraneoplasia. In almost all cases of radiation myelopathy, the latency period is longer than 6 months, and MRI may show tumor swelling or atrophy, and the level of protein in cerebrospinal fluid may be slightly elevated, with lymphocytes present. Radiation myelopathy is irreversible, although some interventions, including corticosteroids, heparin or warfarin, and hyperbaric oxygen, have been suggested to have benefit.
The maximum dose considered safe for spinal cord tolerance and for the prevention of delayed radiation myelopathy is 45 Gy to 50 Gy delivered with conventional fractionation (1.8–2 Gy daily). Schultheiss combined reported data from the literature to establish the parameters of the dose–response function for clinical radiation myelopathy. He used data from 18 reported series that included the number of patients treated with a consistent dose regimen, dose, number of fractions, number of myelopathy cases resulting from the dose regimen, and information about the survival experience of patients at risk. At a 45-Gy dose, the probability of myelopathy is 0.03%; at 50 Gy, the probability is 0.2%. The dose for a 5% myelopathy rate is 59.3 Gy. Quantitative Analyses of Normal Tissue Effects in the Clinic (QUANTEC) analysis demonstrated that, when conventional fractionation of 1.8–2 Gy/fraction is delivered to the full-thickness cord, the estimated risk of myelopathy is less than 1% at 54 Gy and less than 10% at 61 Gy.
Data are limited regarding the risk of radiation myelopathy and repeat radiation to the spinal cord. Data on repeat radiation in animals and humans suggest partial repair of radiotherapy-induced subclinical damage becoming evident about 6 months after radiotherapy and improving over the next 2 years. Follow-up data for spinal cord injury after repeat radiation for recurrent disease is limited and few cases of radiation myelopathy are reported. In general, attempts should be made to avoid the spinal cord if repeat treatment is indicated.
The understanding of spinal cord tolerance with SABR is evolving. The acceptable maximum dose is dependent on dose per fraction. Gibs et al. reported six cases of radiation myelopathy among 1075 patients treated for benign and malignant spinal cord tumors. They recommended limiting the volume of spinal cord treated above an 8-Gy equivalent dose in one fraction. Delayed radiation myelopathy developed at a mean of 6.2 months (range, 2 to 9 months). Saghal et al. evaluated five cases of radiation myelopathy following spine SABR and compared dosimetric data with those in a larger series of patients treated with spine SABR in which no radiation myelopathy occurred. The investigators concluded that the maximum point dose to the thecal sac should be respected for spine SABR. For single-fraction SABR, 10 Gy to a maximum point is safe, and up to five fractions and biologic estimated dose of 30 Gy to 35 Gy secondary to the thecal sac also poses a low risk of radiation myelopathy. This finding was supported by data reported by Macbeth et al., showing no radiation myelopathy at 10 Gy in a single fraction. Based on extensive literature review, QUANTEC for spine radiosurgery demonstrated that a maximum cord dose of 13 Gy in a single fraction or 20 Gy in three fractions appeared to be associated with a less than 1% risk of injury.
Brain
Neurotoxic effects of radiation to the brain are variably assessed in the setting of lung cancer. Data are available, primarily in the absence of controls, for patients with inoperable brain metastases treated palliatively with whole-brain radiation therapy (WBRT), local therapy with surgery or stereotactic radiosurgery for patients treated with and without WBRT, and with prophylactic cranial radiation for either SCLC or NSCLC. The neurotoxic effects of radiation therapy to the brain are difficult to assess because of several factors: most patients with lung cancer who are treated with WBRT have neurologic deficits from brain metastases, long-term follow-up is limited as a result of short survival, and neurologic testing has not been routine.
Series that evaluated neurotoxic effects of radiation for brain metastases have consistently shown that the risk of neurocognitive deficits as a result of WBRT is outweighed by the benefits of treatment. In 1989, De Angelis et al. evaluated 12 patients with neurologic complications attributed to WBRT for brain metastases and reported an incidence of 1.9% to 5.1% for WBRT-induced dementia. All 12 patients, who were treated with total doses of 25 Gy to 39 Gy at 3 Gy to 6 Gy per fraction, had cortical atrophy and hypodense white matter on CT images. The authors concluded that more protracted schedules should be used for the safe and efficacious treatment of good-risk patients with brain metastases.
In RTOG 91-04, a phase III trial designed to assess overall survival of patients with unresectable brain metastases treated with 54.4 Gy/1.6 Gy twice daily or 30 Gy/3 Gy once daily, no difference in overall survival was found between radiation doses; the median survival in both arms was only 4.5 months. A secondary analysis of this study was conducted to evaluate the importance of a Mini-Mental Status Exam (MMSE) before treatment on long-term survival and neurologic function of patients treated with 30 Gy/3 Gy once daily. Both pretreatment MMSE ( p = 0.0002) and Karnofsky performance status ( p = 0.02) were significant factors for survival. WBRT appeared to be associated with an improvement in MMSE score and a lack of decline to below 23 on the MMSE in long-term survivors. Additional analysis of both arms of this trial showed that the use of 30 Gy/3 Gy once daily as compared with 54.4 Gy/1.6 Gy twice daily was not associated with a significant difference in neurocognitive function as measured by MMSE. Control of brain metastases had a noticeable effect on the MMSE score.
Neurocognitive function with a neuropsychometric battery before and after WBRT (30 Gy/3 Gy once daily) was assessed prospectively in a phase III trial of WBRT with or without motexafin gadolinium. Impairment was found in more than 90% of patients at baseline, and the results suggested that only tumor control correlated with neurocognitive function. Li et al. evaluated 135 of 208 patients in the control arm of the study who were available for evaluation at 2 months. The authors found that WBRT-induced tumor shrinkage correlated with better survival and preservation of neurocognitive function. Neurocognitive function was stable or improved in long-term survivors, and tumor progression adversely affected neurocognitive function more than WBRT.
Studies in which patients treated with and without WBRT after local therapy for a limited number of brain metastases are evaluated have also routinely included assessment of neurotoxic effects. This setting provides an opportunity to review neurocognitive effects of radiotherapy in a patient population with a relatively good performance status and less extensive systemic disease. In general, these studies have shown that WBRT can be delivered safely without substantial changes in neurocognitive function and that it improves local control but not overall survival. Chang et al. conducted a phase III trial comparing stereotactic radiosurgery with and without WBRT for patients with one to three brain metastases, with the primary end point being a change in neurocognitive function at 4 months as measured by the Hopkins Verbal Learning Test (HVLT). The investigators found that patients treated with stereotactic surgery plus WBRT had noticeable impairment in learning and memory function by HVLT compared with the patients who were treated with stereotactic radiosurgery alone. This study, however, has been controversial because of unexpected survival differences favoring the stereotactic radiosurgery arm and for the timing of the neurocognitive assessment to one time point.
The European Organisation for Research and Treatment of Cancer (EORTC) conducted a phase III trial assessing whether adjuvant WBRT (30 Gy/3 Gy once daily) increases the duration of functional independence after surgery or stereotactic radiosurgery for brain metastases. Adjuvant WBRT reduced intracranial relapses (surgery: 59% to 27%, p = 0.001; stereotactic radiosurgery: 31% to 19%; p = 0.040) and neurologic deaths. WBRT did not affect the rate of decline in performance status. The median time to World Health Organization performance status higher than 2 was 10.0 months after observation and 9.5 months after WBRT ( p = 0.71).
Aoyama et al. prospectively evaluated WBRT after local therapy for brain metastases and did not find a difference in survival or in neurocognitive function. Intracranial relapse occurred considerably more frequently among patients who did not receive WBRT and, consequently, as demonstrated in other studies, salvage treatment was frequently needed when upfront WBRT was not used. Neurocognitive function was scored 0 to 4 based on the degree of functional impairment and level of assistance required. Neurocognitive function assessment using the MMSE was optional. MMSE data for at least one time point were available for 28 of 44 patients who lived 12 months or longer (16 patients in the WBRT plus stereotactic radiosurgery group and 12 in the stereotactic radiosurgery-alone group) at a median follow-up of 30.5 months (range, 13.7 to 58.7 months). The median MMSE scores before and after treatment were 28 and 27, respectively, in the WBRT plus stereotactic radiosurgery group and 27 and 28 in the stereotactic radiosurgery-alone group. The investigators also evaluated MRI for leukoencephalopathy, and radiographic findings consistent with leukoencephalopathy were found in seven patients in the WBRT plus stereotactic radiosurgery group and in two patients in the stereotactic radiosurgery-alone group ( p = 0.09). Three of these nine patients also had symptomatic leukoencephalopathy; the other six patients were asymptomatic.
Prophylactic Cranial Radiation
Prophylactic cranial radiation is a superior setting to assess the effects of radiotherapy on the whole brain, although it comes with some challenges. Even with the use of prophylactic cranial radiation, survival is limited for patients with lung cancer, routine use of neuropsychologic testing in this patient population is limited, and frequently, patients have baseline neuropsychologic deficits before prophylactic cranial radiation, partially as a result of prior chemotherapy and possibly also because of paraneoplastic effects from the underlying malignant process.
Historically, high rates of toxicity with prophylactic cranial radiation were reported when it was given concurrently with chemotherapy or when it was given at high dose per fraction to patients with SCLC. After low-dose concurrent chemotherapy and prophylactic cranial radiation, 44% of patients with SCLC had abnormal neuropsychologic tests at a median follow-up of 6.2 years. Unexpected neurocognitive deficits have been detected in patients with SCLC after combination chemotherapy, with no noticeable change in those deficits after prophylactic cranial radiation. The authors suggest that neuropsychologic abnormalities associated with SCLC may be secondary to the disease itself (paraneoplasia) and systemic therapy.
Le Péchoux et al. published the results of an international phase III study (PCI99-01, EORTC 22003-08004, RTOG 0212, and IFCT 99-01) comparing 25-Gy and 36-Gy prophylactic cranial radiation for patients with limited-disease SCLC. Over 3 years, the authors found no significant difference between the two groups in any of the 17 selected items assessing quality of life and neurologic and cognitive functions. However, in both groups, there was mild deterioration in communication, memory, intellectual capacity, and leg strength ( p < 0.005 for all).
RTOG 0212 was a randomized phase II trial designed to evaluate the incidence of chronic neurotoxicity and changes in quality of life among patients who received prophylactic cranial radiation for limited-disease SCLC; some patients from this study were also involved in the international phase III prophylactic cranial radiation trial. Patients in RTOG 0212 were treated to 25 Gy/2.5 Gy once daily, 36 Gy/2 Gy once daily, or 36 Gy/1.2 Gy twice daily. There were no significant baseline differences among the treatment groups in terms of quality-of-life measures, and one of the neuropsychologic tests, namely the HVLT. However, at 12 months after prophylactic cranial radiation, there was a significant increase in the occurrence of chronic neurotoxity in the 36-Gy cohort ( p = 0.02). According to logistic regression analysis, increasing age was found to be the most significant predictor of chronic neurotoxicity ( p = 0.005).
RTOG 0214 evaluated the use of prophylactic cranial radiation for patients with locally advanced NSCLC. Prophylactic cranial radiation was shown to considerably decrease the risk of brain metastasis from 18% to 7.7% at 1 year. However, there was no significant difference in overall survival or disease-free survival. A secondary end point of this study was to evaluate the neuropsychologic impact of prophylactic cranial radiation. There were no significant differences at 1 year between the two arms in any component of the EORTC Quality of Life Questionnaire (EORTC-QLQ) C30 or EORTC-QLQ BN20 studies, although a trend for greater decline in patient-reported cognitive functioning was noted with prophylactic cranial radiation. There were no significant differences in MMSE score or activities of daily living. However, for HVLT, there was a significantly greater decline in immediate recall ( p = 0.03) and delayed recall ( p = 0.008) in the prophylactic cranial radiation arm at 1 year.
Gondi et al. reported a pooled secondary analysis of tested and self-reported cognitive functioning of patients treated with prophylactic cranial radiation in RTOG 0212 and RTOG 0214. Among patients with lung cancer in whom brain relapse did not develop, prophylactic cranial radiation was associated with decline in HVLT-tested and self-reported cognitive functioning; however, decline in HVLT and self-reported cognitive functioning were not closely correlated, suggesting that they may represent distinct elements of the cognitive spectrum.
Radiographic Imaging Studies
WBRT is one of the most effective modalities for the treatment and prevention of brain metastases, although it can result in neurocognitive deficits. WBRT is associated with the development of delayed white matter abnormalities or leukoencephalopathy and has been correlated with cognitive dysfunction. The effects of WBRT have been studied in the setting of treatment for intracranial disease and prophylactic cranial irradiation. Prophylactic cranial irradiation is ideal for studying the effect of WBRT, as patients do not have baseline neurologic effects from metastatic or primary tumors in the brain.
Stuschke et al. studied neuropsychologic function and MRI of the brain in patients with locally advanced NSCLC after prophylactic cranial radiation. T2-weighted MR images demonstrated white matter abnormalities of higher grade in patients who received prophylactic cranial radiation than in those patients who did not. Two of nine patients treated with prophylactic cranial radiation and none of four patients not treated with prophylactic cranial radiation had grade 4 white matter abnormalities. A trend toward impaired neuropsychologic functioning was also found in patients with white matter abnormalities of higher degree. Impairments in attention and visual memory in long-term survivors were found among patients in both prophylactic cranial radiation and nonprophylactic cranial radiation groups.
In prophylactic cranial radiation studies, MR images have not been prospectively evaluated before and after therapy for radiation effects and correlation with clinical toxicity. Johnson et al. evaluated CT and MR images of patients 6 to 13 years after receiving prophylactic cranial radiation for SCLC. Findings on CT were abnormal (i.e., demonstrated ventricular dilation, cerebral atrophy, and/or cerebral calcification) in 12 of 15 patients, and white matter abnormalities were present on MR images for seven of 15 patients. Anatomic abnormalities documented by CT and MRI were more frequent among patients with abnormal neuropsychologic function.
Little is known about the factors that predispose patients to white matter changes that occur with WBRT. Sabsevitz et al. used MRI volumetrics to prospectively evaluate the effect of white matter health before treatment on the development of white matter changes after WBRT. Age at the time of treatment and volume of abnormal fluid-attenuated inversion recovery before treatment were significantly associated with white matter changes following WBRT; however, pretreatment fluid-attenuated inversion recovery volume was the strongest predictor of white matter changes after treatment. No significant relationships were found between dose of WBRT, total glucose, blood pressure, or body mass index and development of white matter changes. Szerlip et al. retrospectively reviewed serial MR images and measured volumetric white matter changes over time for patients treated with WBRT and who survived more than 1 year. Following WBRT, white matter changes accumulated at an average rate of 0.07% of total brain volume per month. On multivariate analysis, greater rates of accumulation were associated with older age, poor levels of glycemic control, and the diagnosis of hypertension.
Routine use of MRI before and after therapy and correlation with neuropsychologic assessment are necessary to better understand the neurotoxic effects of brain radiation. Additionally, factors predicting neurologic change or faster rate of neurologic change are important to understand. Paying careful attention to mitigating risk, such as through control of hyperglycemia or hypertension or possibly avoiding or delaying WBRT for patients at high risk of complications, is vital for individualizing care.
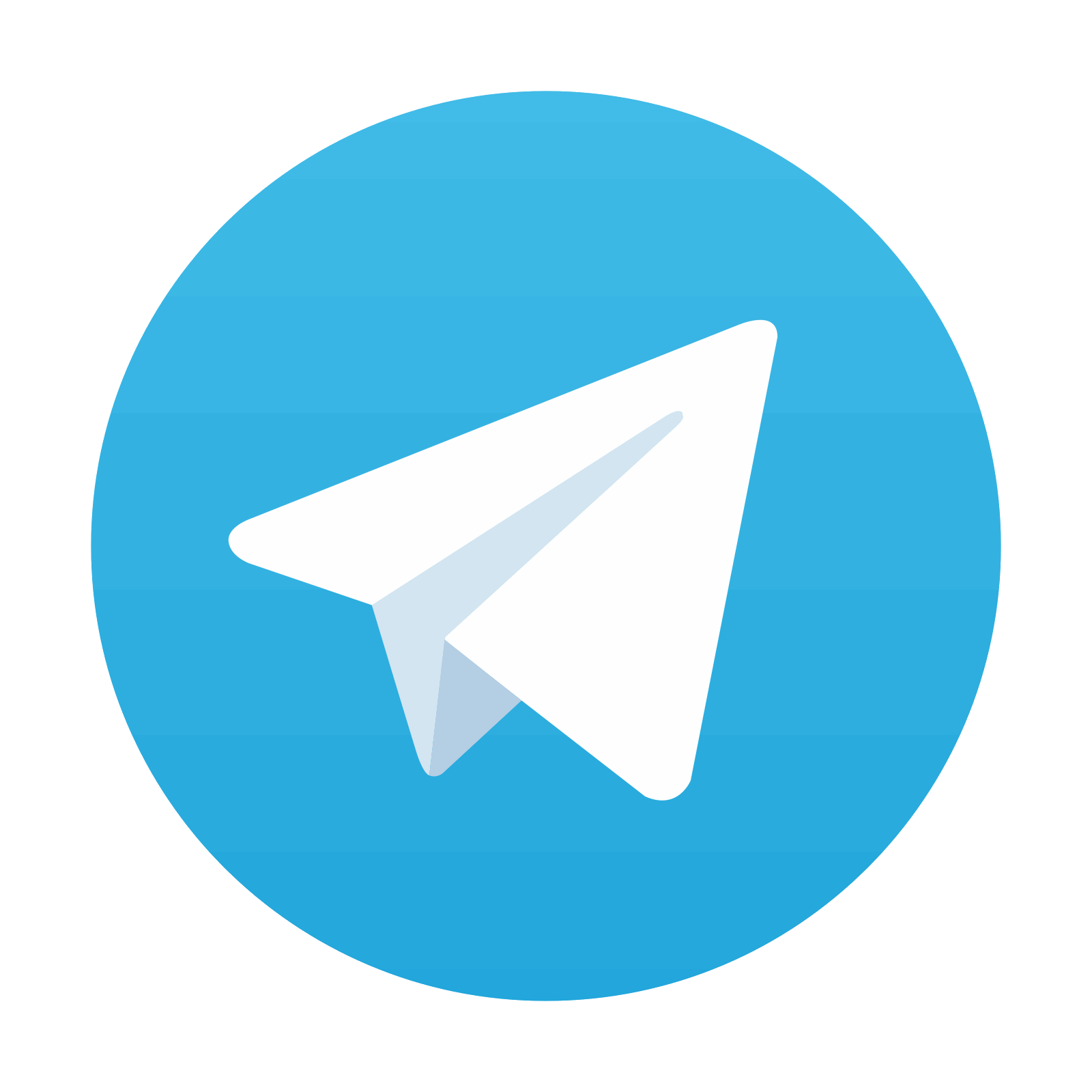
Stay updated, free articles. Join our Telegram channel

Full access? Get Clinical Tree
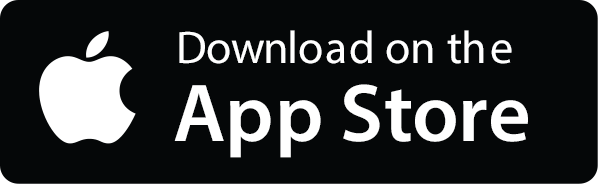
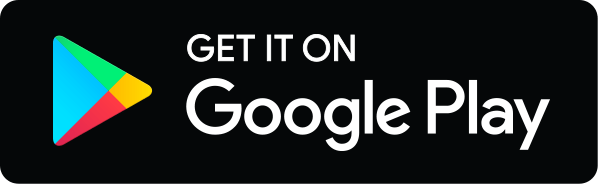