Dramatic advances in noninvasive imaging, cardiopulmonary bypass, surgical technique, and intensive care now allow most patients with congenital cardiovascular defects to undergo surgery during the neonatal period or in later infancy. With these advances, mortality has declined, but many patients are at risk for neurodevelopmental impairment. A natural assumption was that adverse neurologic outcome was directly related to brain injury sustained during neonatal surgical intervention, leading to a seminal study in the late 1980s. The Boston Circulatory Arrest Trial compared two methods of vital organ support in infants undergoing open-heart surgery to repair d-transposition of the great arteries. Much of what is known about the relationship between early intervention for complex cardiovascular disease and neurodevelopmental outcome has been gleaned from this study. However, it is now apparent that injury to the brain may occur during fetal life, at birth, preoperatively, intraoperatively, and postoperatively. The interplay between brain development and the circulation is complex and occurs at many levels. This chapter reviews mechanisms influencing neurologic outcome, including (1) shared genetic and developmental pathways, (2) physiologic effects of congenital cardiovascular lesions on brain blood flow, and (3) the timing, appearance, and mechanism of acquired brain injuries. We summarize how these pathogenic mechanisms result in a neurodevelopmental “signature” of congenital cardiovascular disease. Finally, we will speculate on how these mechanisms suggest strategies of neuroprotection, repair, and recovery that may improve outcome.
Certain aspects of heart and brain development occur simultaneously in the human fetus (summarized in Chapter 1 for heart and below for brain). Many vertebrate organs undergo related developmental events (eg, cell fate determination, cell migration, dorsal/ventral patterning, left/right asymmetry, area specification, etc.). Thus, it is not surprising that similar genes share important and similar developmental roles in both organs (Table 14-1). This includes genes such as those in the Ras-MAPK pathway, members of the transforming growth factor β family, fibroblast growth factor family members, notch and notch ligands, and vascular endothelial growth factor. Disruption of shared fundamental genetic pathways that result in cardiac defects will affect brain development as well.
Gene | Function in cardiac development | Function in brain development | Syndrome or isolated CHD |
---|---|---|---|
Nkx2.5 | Cardioblast cell fate commitment, chamber septation | Neural cell fate commitment | Holt-Oram, ASD, VSD, TOF |
TBX5 | Left ventricular specification | Cortical area specification, axon guidance | Holt-Oram |
Lefty1 | Left/right asymmetry | Neural cell fate commitment, left/right asymmetry | Heterotaxy |
ZIC1 | Left/right asymmetry | Neural progenitors proliferation, neural crest and roof plate specification, holoprosencephaly, cerebellar development | Heterotaxy |
GATA4 | Heart tube formation | Astrocyte proliferation | ASD, VSD |
NF-1 | Myocardial growth | Glial differentiation | |
TFAP2B | Neural crest | Regulates monoaminergic gene expression in neural crest cells in midbrain, hindbrain, and spinal cord | Charge |
Notch/Jagged | Cardiac progenitor cell fate determination | Neural cell fate commitment | Alagille, aortic stenosis/bicuspid aortic valve |
TBX1 | Outflow tract, pharyngeal arch development, aortic arch patterning | Learning and memory (prepulse inhibition) | DiGeorge |
Ras-MAPK | Pulmonary valve development, cardiomyopathy | Pleomorphic: learning memory, neuronal survival/death, plasticity | Noonan cardio-facio-cutaneous, Costello |
Elastin/Limk1, Cyln2, Fzd9; GtfII | Elastin: valve formation | Limk1, Cyln2: regulation of neuronal cytoskeleton—growth cone motility, dendrite formation, synaptogenesis; Fxd9: hippocampal development; Gtf2i: visuospatial processing | Williams-Beuren |
PROSIT240 | Ventricular septation | Cerebellar development | D-TGA |
Congenital cardiovascular disease frequently occurs within the context of a genetic syndrome. Often but not exclusively, these syndromes also include abnormal neurodevelopment. Notable examples of genetic syndromes with both congenital cardiovascular disease and neurodevelopmental impairment include trisomy 21 (Down syndrome), 22q11 deletion (DiGeorge and velocardiofacial syndrome), monosomy X (Turner syndrome), and Jacobsen and Williams syndromes. Despite well-characterized chromosomal microdeletions, identifying a specific gene or limited set of genes that accounts for any one component (eg, cardiac or brain) of the syndrome is challenging. The 22q11 deletion syndrome is a good example. The causative microdeletion encompasses three megabases of DNA, representing 30 to 40 genes. The syndrome is notably highly heterogeneous with regard to all potential components of the syndrome (eg, parathyroid, immune deficiency, conotruncal cardiac defects, and neurodevelopmental outcome). Even when known genetic or malformation syndromes are excluded, a large prospective study of the determinants of 1-year neurodevelopmental outcome identified genetic syndromes, unsuspected at birth, as among the most potent predictors of adverse outcome. The list of single-gene defects contributing to congenital cardiovascular disease and/or neurodevelopmental impairment is likely to grow quickly with the advent of large-scale genomics projects.
Beyond the direct effects of single genes or shared genetic pathways in genetic syndromes, patient genotype can influence neurodevelopmental outcome as “risk modifiers” of the response to brain injury. This is perhaps best described for alleles of apolipoprotein E, in which the Apo ε4 allele is associated with adverse outcome in many conditions in adults (eg, Alzheimer’s disease, traumatic brain injury, stroke, and subarachnoid hemorrhage). In infants with congenital cardiovascular disease, however, the Apo ε2 allele is associated with worse outcome. Interestingly, polymorphisms of inflammatory cytokines, including interleukin-6, have been associated with cerebral palsy in term newborns. This issue has not been examined in patients with congenital cardiovascular disease despite observations that inflammatory cytokines, including interleukin-6, are increased after surgery and are associated with postoperative cardiovascular morbidity.
Human cardiac development begins during the first postconceptual days with rhythmic contractions of a primitive heart tube beginning by embryonic day 23 and a morphologically mature heart formed by day 50, or gestational week 7 (reviewed in Chapter 1). In contrast, brain development extends over a much longer time period, with morphologic events (cell proliferation, migration, axon pathfinding, and target selection) occurring in the first two trimesters, followed by a prolonged period of refinement of connections that occurs in both the third trimester and the early postnatal period.
A primitive neural tube forms by gestational week 5 with identifiable radial glia, the neural stem cell. Corticogenesis, the process of production and migration of neurons from regions of proliferation to their targets in specific neocortical layers, occurs from week 7 through week 18, although a mature six-layered cortex does not appear until week 26. Axon outgrowth, pathfinding, target selection, and innervation all take place after production and migration of neurons. Formation of connections between the thalamus and cortex requires a transient population of early born neurons, referred to as subplate neurons because of their location as a discrete layer below the cortical plate. In humans, thalamocortical pathfinding takes place between the histological emergence of the subplate at the end of the first trimester and the appearance of cholinesterase positive fibers in the subplate at weeks 17 to 20 (earlier for somatosensory vs. visual cortex). Thalamocortical fibers accumulate during a “waiting period” from week 17 through weeks 22 to 26 before innervating the cortical plate.
Most morphologic events of brain development are completed by the end of the second trimester. The third trimester involves a period of dramatic brain growth and refinement of connections that are dependent on endogenous and spontaneous neuronal activity arising at multiple levels. In the visual system, this endogenous activity takes the form of spontaneous waves of neuronal activation that sweep across and tile the retina and are then transmitted to the thalamus and cortex. This patterned activity sculpts developing neural circuits into mature, precise patterns. Ocular dominance columns in the visual system, representing nonoverlapping eye-specific innervation, are a representative example of such a patterned circuit and form the basis of binocular vision. In higher primates, ocular dominance columns have fully formed by birth before onset of visually driven activity. At a gross morphologic level, the third trimester is characterized by development of secondary and tertiary gyri. At a cellular level, brain growth involves elaboration of dendritic arbors and formation of corticocortical connections. Myelination of fiber tracts also begins during fetal life, with a characteristic caudal-to-cranial pattern beginning with deep structures, such as the tegmentum and cerebellar peduncles. By the end of the third trimester, myelination extends to the posterior limb of the internal capsule and involves the motor fibers of the pyramidal tract. In neocortex, myelination begins in the optic radiations and occipital white matter after birth before extending to the frontal lobes by 9 months of age.
Brain development continues after birth, with sensory driven activity now influencing the refinement of connections. For a brief period of time (critical period), soon after the onset of vision, deprivation of visually evoked activity leads to permanent loss of visual acuity in the deprived eye, a process referred to in humans as amblyopia, which represents a form of neural plasticity. In humans, amblyopia is usually observed only after 6 months of age. Weeks of deprivation can lead to substantial loss of visual acuity between 6 and 18 months, and months of deprivation can have an effect until 8 years of age.
The fetal circulation is unique in a number of respects that impact cerebral blood flow. As reviewed in detail in Chapter 3, vascular and cardiac blood flow patterns in the normal fetus direct the most highly saturated blood from the ductus venosus and left hepatic veins via the foramen ovale to the left heart and, subsequently, to the cerebral circulation. In contrast, in d-transposition of the great arteries, the aorta arises from the right ventricle and thus receives the relatively desaturated blood from the superior vena cava, lower body, and coronary sinus. In hypoplastic left heart syndrome, the fetal circulation is characterized by admixture of all venous streams in the right atrium and ventricle. The ascending aorta is a very small vessel, delivering blood in a retrograde direction to the coronary arteries. The aortic arch is also hypoplastic and shows flow reversal to supply the brain and upper body (see Chapters 3 and 8). The effects of these abnormal flow patterns on brain development are uncertain but may be very different despite the fact that both decrease the oxygen content of the blood delivered to the brain. In d-transposition of the great arteries, the pulsatility and perfusion pressure of the cerebral arterial circulation are normal. On the other hand, in hypoplastic left heart syndrome, the hypoplastic isthmus and aortic arch may function as resistors, decreasing the pulsatility and perfusion pressure to the cerebral circulation. This cannot easily be overcome by the cerebral circulation, particularly early in gestation, and may explain the finding of a recent study showing that ascending aortic diameter predicts the degree of microcephaly in newborns with hypoplastic left heart syndrome.
Blood flow to the fetal brain is estimated to be almost one-quarter of the combined ventricular output in the third trimester. Local regulation of fetal cerebral blood flow is thought to redistribute blood flow to the brain in the setting of placental insufficiency, a phenomenon referred to as “brain sparing,” a pattern of in which overall somatic growth is restricted but head growth is relatively preserved. In the setting of congenital cardiovascular disease with decreased oxygen content of the cerebral arterial blood, similar mechanisms may be invoked; for example, the ratio of cerebral to placental resistance can decrease so that cerebral oxygen delivery can be preserved. However, low cerebral blood flow has been measured in newborns with hypoplastic left heart syndrome and d-transposition of the great arteries using magnetic resonance imaging, and newborns with certain forms of congenital cardiovascular disease have smaller head circumferences, which may be an indicator of impaired brain growth. The issue is complex, however. The different patterns of alterations in cerebral blood flow are associated with different patterns of growth disturbance. Newborns with d-transposition of the great arteries tend to have small head circumference with normal birth weight, whereas those with hypoplastic left heart syndrome are smaller in all dimensions but head volume is disproportionately decreased. Interestingly, infants with isolated aortic coarctation have a greater head volume relative to birth weight.
Advanced magnetic resonance imaging (MRI) provides the highest-resolution conventional images of brain anatomy and acquired brain lesions. These techniques can also be used to measure brain development and to investigate brain metabolism and microstructure. Proton MR spectroscopic imaging measures resonance from N-acetyl groups (predominantly N-acetylaspartate), lactate, creatine, and tetramethylamines (predominantly choline-containing compounds). N-acetylaspartate is found predominantly in neurons (cell body and axon) so that changes in N-acetylaspartate reflect neuronal metabolic integrity. Particularly relevant to studies of brain development is the observation that N-acetylaspartate increases consistently with advancing cerebral maturity, providing a developmental brain “growth chart.”
Another advanced technique is diffusion tensor imaging, which provides a sensitive measure of regional brain microstructural development. Three-dimensional spatial distribution of water diffusion is characterized in each voxel of the MR image. With increasing brain maturation, brain water content diminishes, and developing neuronal and glial cell membranes increasingly restrict proton diffusion, resulting in a consistent decrease in average diffusivity over time in gray and white matter regions (Figure 14-1).
FIGURE 14-1.
Fetal MRI and diffusion imaging detects delayed brain development in congenital cardiovascular disease. A–C. Sagittal, axial, and coronal T2 images at 31 weeks in a fetus with prenatal diagnosis of hypoplastic left heart syndrome. The brain is morphologically normal. D and E. Average diffusivity coefficient (ADC) is plotted against gestational age for periatrial white matter (D) or thalamus (E). Values for three fetuses with hypoplastic left heart syndrome (orange) are compared with a control cohort without congenital cardiovascular disease (green). The regression line was fit to the control population. Average diffusion is higher in fetuses with hypoplastic left heart syndrome, indicating a relative delay in development.
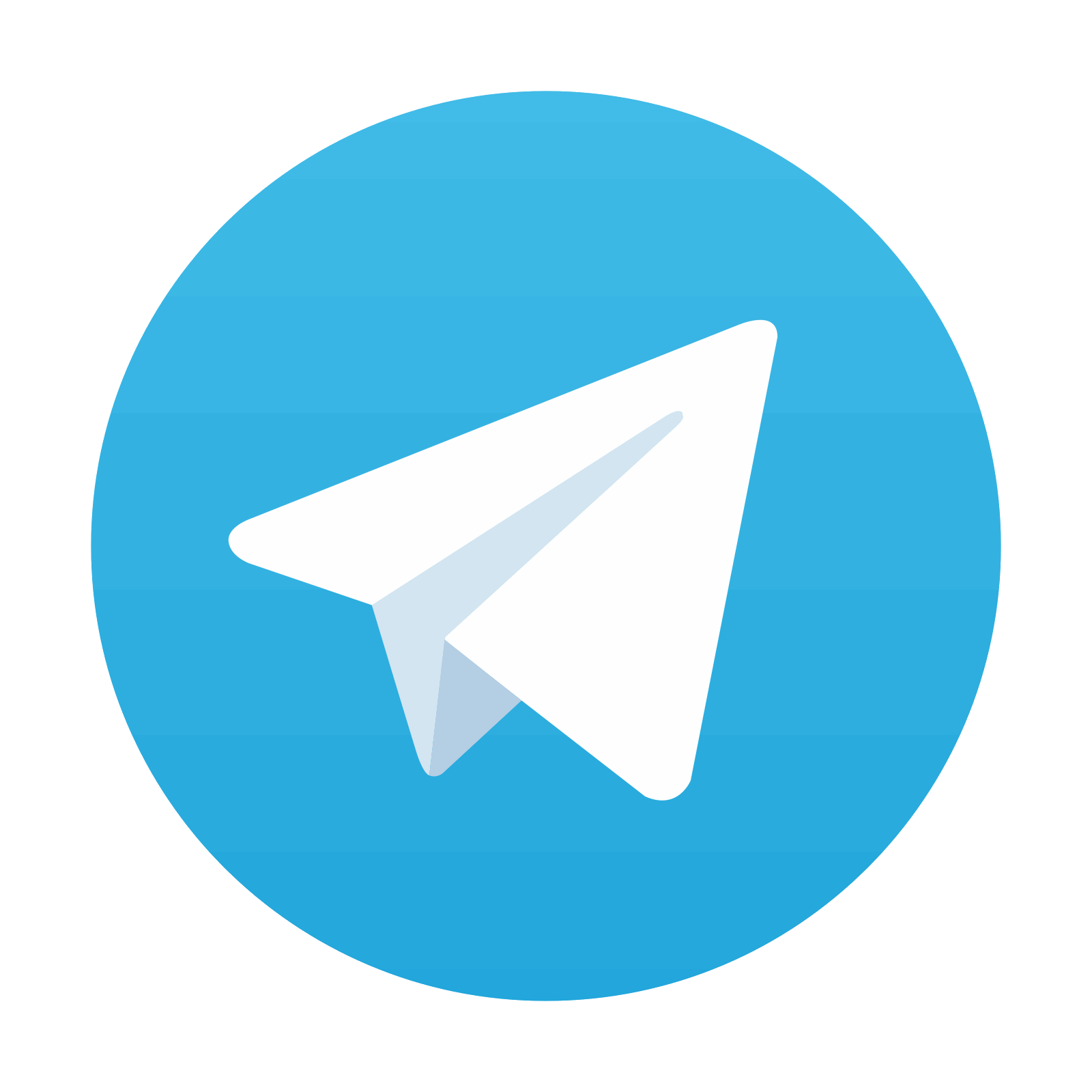
Stay updated, free articles. Join our Telegram channel

Full access? Get Clinical Tree
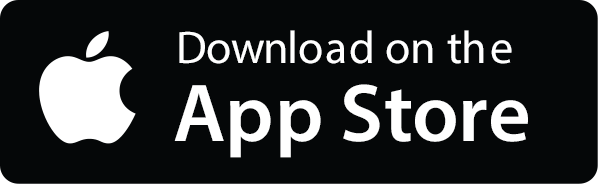
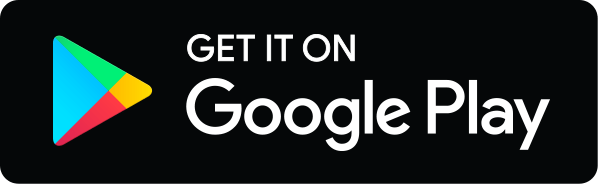
