Approximately 40,000 children are born in North America each year with congenital heart disease (CHD). Approximately half of these children require no therapy, because the defect is relatively minor (eg, a bicuspid aortic valve) or spontaneously heals (eg, a small ventricular septal defect). However, in the other half of these children, surgical or catheter intervention is necessary. For some of the children in this group, the CHD does not cause hypoxemia, circulatory insufficiency, or symptoms; these children typically undergo repair on an elective basis in childhood (eg, atrial septal defects or progressive valvar disease). In this group of school-age children, it has been shown that there is little to no impact on the central nervous system from either the unrepaired defect or from the effects of anesthesia, surgery, or postoperative care following the repair.1
In contrast, it is now increasingly recognized that the group of children with CHD severe enough to require surgery shortly after birth (eg, transposition of the great arteries [TGA], hypoplastic left heart syndrome [HLHS], total anomalous pulmonary venous return) or in early infancy (eg, tetralogy of Fallot, atrioventricular canal, large ventricular septal defects) are at increased risk for neurologic, behavioral, and psychological abnormalities as they mature. For the purposes of this chapter, we will discuss the findings on the group of children who require surgery in early infancy—those considered to have “complex congenital heart disease” (cCHD).
With improvements in diagnosis, surgery, and perioperative care, it is estimated that 95% of children born in 2010 with cCHD will survive into childhood and beyond. Current research discussed below suggests that approximately half will have neurologic, behavioral, psychosocial, or cognitive abnormalities. Unless there is a decrease in the prevalence of these problems, over the next 2 decades it is estimated that an additional 130,000 children will enter the U.S. school system at significant risk for educational, interpersonal, and, ultimately, occupational impairment (Figure 11-1).
Initially, it was presumed that hypoxemia, intraoperative factors, or both were the likely causes of adverse developmental outcome, but current data suggest that the factors are multiple and probably interactive. Children with cCHD have an increased risk for congenital structural central nervous system (CNS) abnormalities and presurgical white matter injury, stroke, hemorrhage, and microcephaly. About 50% have neurologic or neurobehavioral abnormalities before their first surgery, including hyper- or hypotonia, motor asymmetry, micro- or macrocephaly, jitteriness, poor state regulation, and/or a weak suck reflex. The specific cardiac defect also plays a role in subsequent developmental risk. It appears that differences in fetal blood flow and substrate delivery that occur in the presence of cCHD lead to immaturity of brain development and render term infants with cCHD vulnerable to the types of brain injury seen in premature infants.2,3
Multiple factors can contribute to brain injury during and after surgical repair (Table 11-1). The type of support during surgery (deep hypothermic circulatory arrest versus continuous cardiopulmonary bypass) has been studied extensively, and although prolonged use of either is clearly disadvantageous, the nature of the defect that leads to the prolonged surgical time may be critical rather than the technique itself. Other factors include the degree of cooling, use of hemodilution, and type of blood gas and pH management. Postoperative factors have been studied less frequently but appear to include hemodynamic lability leading to reduced cerebral blood flow and oxygen delivery, hyperthermia, and the length of the intensive care unit stay itself.2,3
Fetal | Preoperative | Intraoperative | Postoperative | Other |
---|---|---|---|---|
Abnormal cerebral resistance | Decreased cerebral blood flow | Hypothermia | Hypoxemia | Parental stress |
Decreased cerebral blood flow | Decreased oxygen delivery | Anesthetic agents | Hypotension | Socioeconomic status |
Decreased oxygen delivery | Cardiac arrest | Cardiopulmonary bypass | Cardiac arrest | Genetic syndromes |
Low birth weight | Paradoxical embolus | Circulatory arrest | Decreased cerebral blood flow | Genetic polymorphisms |
Prematurity | Regional cerebral perfusion | Embolism | ||
Glucose management | Hypothermia |
Early brain and heart organogenesis occurs simultaneously in the human fetus. Similar developmental programs are invoked for both systems including stem and progenitor cell proliferation, cell fate commitment, migration, and left/right and dorsal/ventral patterning.4-6 The cardiovascular system is the first organ system to function in the embryo and is complete at about 8 to 10 weeks of gestational age. This precocious development is necessary because the nutritional and oxygen demands of the growing fetus can no longer be satisfied by diffusion alone. The development of the brain begins concurrent with cardiovascular development but continues throughout the pregnancy with a dramatic increase in brain size in the third trimester of gestation (Figure 11-2A) due to elaboration of neuronal microstructure (eg, dendrites, axons, synapses) and the onset of myelination, which continues for many years postnatally. The formation and refinement of connections in the brain require neuronal activity and glial maturation. These both lead to an increase in brain metabolism with dependence on heart function for oxygen and substrate delivery.
Figure 11-2

A. Expected in utero brain growth as a percentage of full-term brain weight. There is a rapid rise in brain growth during the third trimester. B. A comparison of measured total brain volumes (TBV) from fetal brain magnetic resonance imaging in fetuses with congenital heart disease (CHD) (open circles) and fetuses without CHD (filled squares). (Adapted with permission from Limperopoulos C, Tworetzky W, McElhinney DB, et al. Brain volume and metabolism in fetuses with congenital heart disease: evaluation with quantitative magnetic resonance imaging and spectroscopy. Circulation. 2010;121:26-33.).
Down (trisomy 21), Williams (chromosome 7q11 deletion), Noonan (several genes), and DiGeorge (chromosome 22q microdeletion) syndromes are examples of known genetic causes of both cardiac malformations and neurodevelopmental deficits. There are likely additional, as yet unidentified, genetic effects on both heart and brain because similar morphogenetic events in brain and heart development engage many of the same genes such as sonic hedgehog4 (progenitor proliferation and outflow tract development) and notch/jagged7 (cardiac and neural progenitor cell fate, cardiac ventricle formation, and angiogenesis).
It has long been observed that the head circumferences in infants with cCHD are smaller than those of infants of similar gestational ages without cCHD3,8 (Figure 11-2B). Not only are the head circumferences smaller, but infants with CHD are prone to a hypoxic-ischemic white matter injury that is not distinguishable from periventricular leukomalacia, an injury seen primarily in premature infants (Figure 11-3). Importantly, in mixed populations of infants with cCHD, this injury is seen in about 20% of patients before surgery and in over 50% after surgery.2
Figure 11-3

Typical distribution of periventricular leukomalacia (PVL) lesions from 9 presurgical patients with either hypoplastic left heart syndrome (HLHS) or transposition of the great arteries (TGA). The tracings are overlaid on a brain atlas composed of a composite of 42 T2 volumetric brain magnetic resonance images (33 with HLHS and 19 with TGA).
Relative to the normal control newborns, newborns with cCHD had 10% lower N-acetylaspartate/choline ratios and 4.5% higher diffusivity on nuclear magnetic resonance spectroscopy.9 Comparing these data to values obtained from normal fetuses suggests that term newborns with cCHD have a delay in brain development of approximately 1 month, equivalent to an infant born prematurely at 34 to 36 weeks. Licht et al.8 evaluated brain maturation in 42 full-term (average gestational age, 38.9 ± 1.1 weeks) infants born with CHD prior to surgery. Based on (1) degree of myelination, (2) degree of cortical folding, (3) the radiographic presence or absence of germinal matrix in the anterior and posterior horns of the lateral ventricles, and (4) the presence and number of migrating bands of glial cells, these infants had symmetric delays in brain development across all 4 areas assessed, appropriate for infants born at 35 weeks of gestation. Similarly, using fetal magnetic resonance images, Limperopoulos et al.3 performed a cross-sectional study comparing brain volumes in fetuses with and without CHD. They concluded that there was divergence of brain growth starting at the beginning of the third trimester3 (Figure 11-2B). Thus, the finding of a delay of 4 to 5 weeks is consistent across institutions and studies.
The areas of the affected white matter (Figure 11-3) are homologous to the periventricular areas rich in premyelinating oligodendrocytes in animal models. Failure of nutrient and oxygen delivery during late gestation, transition from fetal to neonatal blood flow, or postnatal/presurgical care results in white matter ischemia in the areas of brain (periventricular white matter) where premyelinating oligodendrocytes reside. Delayed brain development, at the biochemical, cellular, and structural levels, may underlie the preponderance of injury to periventricular white matter in term newborns with cCHD as well as the increased risk for an open operculum.2,3,8,9
More recently, it has been shown that the abnormal circulation in cCHD will cause significant abnormalities of cerebral blood flow in the fetus. In the fetus without CHD, gas exchange occurs in the placenta. Oxygenated blood returns through the umbilical vein and ductus venosus to the portal vein, inferior vena cava, and right atrium and deoxygenated blood returns to the placenta via the umbilical artery (Figure 11-4A). Prior to birth, blood flow to the lungs is very low due to elevated pulmonary vascular resistance and relatively low lung volumes. Two connections exist between the systemic and pulmonary circulations: the foramen ovale connecting the right and left atria, and the ductus arteriosus between the pulmonary trunk and descending aorta. Oxygenated umbilical venous blood is preferentially directed through the ductus venosus into the left lobe of the liver. As a result, the oxygen saturation is higher in the left hepatic veins as they join the inferior vena cava, resulting in streams of blood with different saturations. The higher saturated stream containing blood from the ductus venosus is preferentially directed across the foramen ovale to the left atrium. This blood mixes with the limited amount of pulmonary venous blood returning from the lungs. The resulting oxygen saturation in the fetal left ventricle, and thus to the brain, is approximately 65%. Blood ejected by the right ventricle consists of venous blood from the superior vena cava, as well as the relatively desaturated streams from the inferior vena cava and coronary sinus. The resulting oxygen saturation in the fetal right ventricle is approximately 55%.
Figure 11-4

A. Normal fetal circulation: Red (oxygenated) blood from the placenta is preferentially streamed from the ductus venosus through the right atrium to the left side of the heart and then pumped via the ascending aorta to the brain and body. B. Fetal circulation with transposition of the great arteries: Red blood circulates normally to the left heart structures but is then pumped via the common pulmonary artery to the ductus arteriosus and the descending aorta. Blue (low oxygen–containing) blood returns to the right atrium from the body and the brain and is pumped via the ascending aorta to the brain. C. Fetal circulation with hypoplastic left heart syndrome: Complete mixing of red and blue blood occurs at the level of the right atrium. Blood is pumped to the common pulmonary artery and the ductus arteriosus. Antegrade flow delivers blood to the lower body, and retrograde flow in the transverse arch delivers blood to the head and brain. (Adapted with permission from Johnson, BA and Ades, A. Delivery room and early postnatal management of neonates who have prenatally diagnosed congenital heart disease. Clinics in Perinatology. 2005;32:921-946.).
In most forms of cCHD, the abnormal anatomy will lead to abnormalities of fetal oxygen content, differences in intravascular streaming, and/or changes in cerebral vascular resistance, all of which may lead to abnormalities of cerebral oxygen delivery. For example, in TGA and HLHS, specific alterations in fetal blood flow may lead to decreased brain oxygen delivery. In TGA, the aorta arises from the right ventricle and thus receives the relatively desaturated blood from the superior vena cava and lower saturation stream of blood in the inferior vena cava (Figure 11-4B). The higher saturated stream from the left hepatic veins is directed normally across the foramen ovale to the morphologic left ventricle. The left ventricle, however, is connected to the pulmonary trunk, and this higher saturated blood (approximately 65%) is delivered to the lungs and lower body. In HLHS, the fetal circulation is characterized by obstructed antegrade flow at the level of the left atrium. This results in reversal of flow across the foramen ovale and reduced or absent flow through the left ventricle and into the ascending aorta. All systemic and placental venous return mixes in the right ventricle and is ejected into the pulmonary trunk and ductus arteriosus, the beneficial effects of streaming seen in the fetus without cCHD are absent. In addition, flow to the head and neck vessels primarily occurs by retrograde flow into the transverse aorta (Figure 11-4C). Fetuses with HLHS have been shown to have lower cerebral vascular resistance than normal fetuses, and in particular, neonates with HLHS and aortic atresia (with obligate retrograde flow to the brain) are the most likely to have microcephaly at birth.10 In both TGA and HLHS, as well as most other forms of cCHD, disordered fetal circulation places the brain at risk for disrupted growth and development, particularly during the third trimester.2,3,8-10
Developmental scores discussed throughout this chapter refer to standard scores with a population mean of 100 and standard deviations (SD) of 15. Thus, a score of 85 is 1 SD below the mean, and approximately 16% of a normally distributed population would be expected to score below this. A score of 70 is 2 SD below the mean, and only about 2.5% of the population would be expected to score below this. Depending on the test, 1, 1.5, or 2 SD below the mean is considered delayed, or there may be a distinction between mild-moderate (1-1.5 SD) and severe delay (>2 SD below the mean). (See Appendix 11-1 for additional information about some commonly used developmental assessment tools.)
Cognitive abilities in infants and toddlers with cCHD are typically within the average or “normal” range but lower than the corresponding population mean. On the Mental Development Index (MDI) of the Bayley Scales of Infant Development, infants and toddlers with cCHD have an average MDI of 89 to 95 ± 9 to 17.2,11-15 Approximately 5% to 11% of infants and toddlers have an MDI of less than 70.14,16,17
Motor delay is the most common and prominent finding in this age group. The same cohorts described earlier have Psychomotor Development Indices (PDI) from the Bayley Scales of 73 to 91 ± 13 to 23.2,11-16 Approximately 14% to 48% have PDI scores less than 70. On a specific test of motor function, the Peabody Developmental Motor Scales, a similar percentage (42%) of children with cCHD showed delays (>1.5 SD below the mean) in gross and fine motor skills 12 to 18 months after surgery in the first 2 years of life.17
The single most important factor in predicting a lower MDI or PDI is the presence of a known genetic condition, such as trisomy 21 or chromosome 22q11 microdeletion.12 When scores are separated based on the presence of a known genetic condition, these infants and toddlers have a mean MDI of 74 ± 14 and PDI of 60 ± 12, whereas subjects without a known genetic condition have a mean MDI of 93 ± 13 and PDI of 82 ± 16.11
Specific measures of speech and language demonstrate high rates of receptive and expressive language as well as phonologic (articulation) delays. Thirty-four percent of children with cCHD show language delays (primarily expressive) that are greater than 1.5 SD below the mean for age.17 As early as 1 year of age, it can be observed that children are excessively quiet with rare use of recognizable words. At 2.5 years of age, delays in the development of vocabulary, word usage, word endings, and combining words are evident.18 Approximately 25% of children are diagnosed with apraxia of speech,19 a condition in which speech is limited by oral–motor planning and integration deficits.
Behavior and temperament are atypical prior to surgery in over one third of infants. They are described as being more irritable or lethargic.20 On the Early Infancy Temperament Questionnaire, infants with a single-ventricle form of cCHD have more negative mood and are more difficult to soothe compared with controls.21
The Functional Independence Measure for Children (WeeFIM), the Vineland Adaptive Behavior Scale, and the Adaptive Behavioral Assessment System-II are commonly used, parent-report measures of children’s function in a variety of domains of daily life. Children at 18 to 24 months of age who had a Norwood or arterial switch procedure at less than 6 weeks of age are 4 times as likely to have a delay on the General Adaptive Composite of the Adaptive Behavioral Assessment System-II compared with the norm group.22 In a cohort specifically excluding children with HLHS, assessment at 12 to 18 months after the first open-heart surgery shows lower than average developmental quotients (77-92 ± 24-30) for self-care, mobility, and cognition on the WeeFIM, with only 21% functioning at age level. Standard scores for daily living skills and socialization are also in this range on the Vineland Adaptive Behavior Scale, with greater than half of the children exhibiting poor socialization skills.20 These results suggest that social impairment may become apparent in toddlers and young children when a greater variety and complexity of social interaction is expected.
The presence of a life-threatening chronic condition can have a substantial impact on family function, which can impact parent–child interaction and neurodevelopmental outcome. At 3 months of age, infant negative mood and difficulty to soothe are predictive of higher parenting stress and are more common in infants with single-ventricle physiology.21
At the age of school entry, full-scale intelligence quotient (IQ) scores typically remain in the average range (89-97 ± 7-21) on the Wechsler Preschool and Primary Scale of Intelligence–Third Edition and other intelligence tests.23,24 Children with tetralogy of Fallot tend to have a higher IQ than those with HLHS, although both are within the average range (111 versus 97, respectively; McCarthy Scales of Children’s Abilities).25 Despite mean IQs in the average range, approximately 20% to 33% of children have IQs greater than 1 SD below the mean, and approximately 11% have IQs greater than 2 SD below the mean.24,26
Approximately 25% of 4 to 5 year olds score greater than 1 SD below the mean and 10% score greater than 2 SD below the mean on the total language score of the Preschool Language Scale 4, indicating moderate and severe language impairment, respectively.26 Mean scores on tests of single-word receptive and expressive vocabulary tend to be similar to population means,24,25 with better scores in TGA compared with HLHS.25 However, more complex language productions, such as narratives, which require memory and organization as well as language skills, are deficient compared with control norms in greater than 50% of 4 year olds.19 The narratives of preschoolers with cCHD are characterized by shorter length and descriptive depth. They contain less information and fewer language devices related to evaluation (eg, “he wanted to”), qualification (eg, “he almost fell”), and style (eg, repetition for emphasis). Narrative competency may be especially relevant because it can be deficient even when earlier language morphology and syntax were not and because it may be particularly important for socializing, comprehension, and literacy.18,19
At 4 to 5 years of age, the relatively high rate of neurologic abnormalities and gross and fine motor delays in children with cCHD persists. Mean Peabody gross motor quotients are 83 ± 12, with 49% scoring below 78 (1.5 SD below the mean), indicating significant delay. The same group has a mean fine motor quotient of 86 ± 16, with 39% scoring less than 78.27 Motor findings at 12 to 18 months are strongly predictive of findings at 4 to 5 years of age,17,27 suggesting that motor delays do not “catch up” over this time period.
Fine motor, visual–spatial, and visual–motor integration skills take on increased significance as children are expected to participate in more complex play activities; to increase independence in eating, grooming, and dressing; and to develop writing skills. On the Beery-Buktenica Developmental Test of Visual Motor Integration (Beery VMI) and other tests of visual–motor ability, several cohorts of preschoolers with cCHD have mean fine motor and visual–motor scores in the mid-80s to high 90s (range, 69-135). Between 12% and 30% scored greater than 1 SD below the population mean (moderate impairment), whereas 9% to 12% scored greater than 2 SD below the population mean (severe impairment).23,25
Executive functions are the collection of metacognitive abilities that allow humans to persist to accomplish goal-directed behavior. They include working memory (the ability to hold information in mind while analyzing it or completing a related task); behavioral inhibition (the ability to avoid distraction or impulsive reacting that interferes with accomplishing a task); the regulation of attention and cognitive effort (both the ability to persist when appropriate and to shift when appropriate); the abilities to organize, plan, and strategize (use previous experience to guide future behavior); and the ability to monitor one’s performance of all of these skills to adapt to changing demands in “real time.” Executive functions appear to be based in the frontal lobe, particularly the prefrontal cortex, and its connections to other brain regions. They continue to develop throughout childhood and into young adulthood, as does the frontal lobe. This uniquely protracted period of brain development makes the prefrontal cortex and executive functions uniquely vulnerable to a wide variety of conditions that adversely affect brain function. On the “statue” subtest of the NEPSY (Developmental Neuropsychological Assessment), which measures motor control and behavioral inhibition, 33% of children with cCHD are moderately impaired and 11% are severely impaired.26
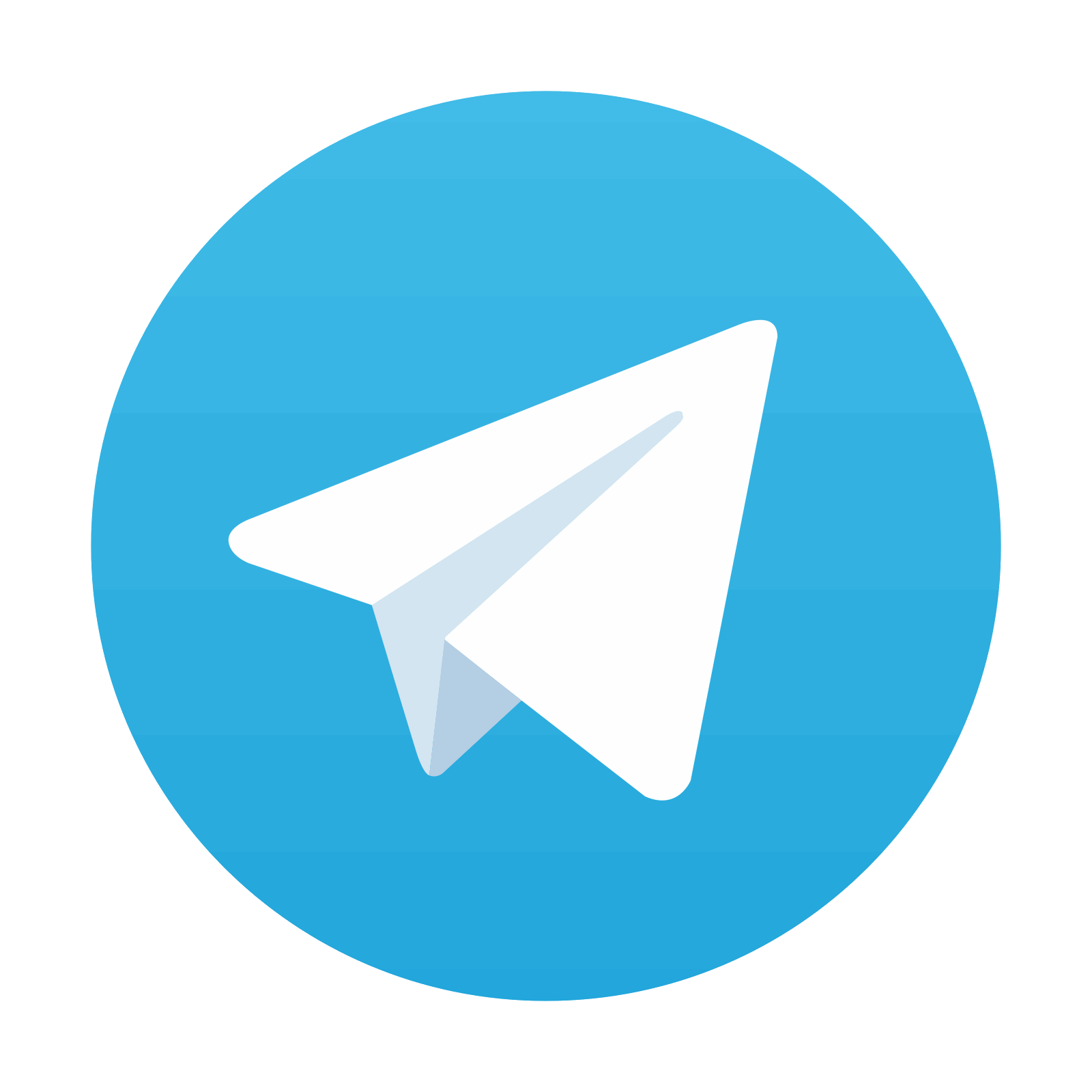
Stay updated, free articles. Join our Telegram channel

Full access? Get Clinical Tree
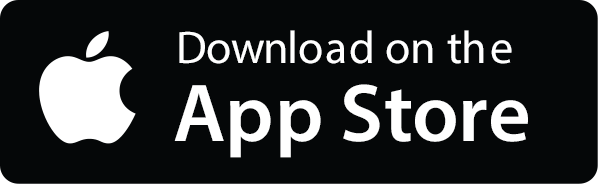
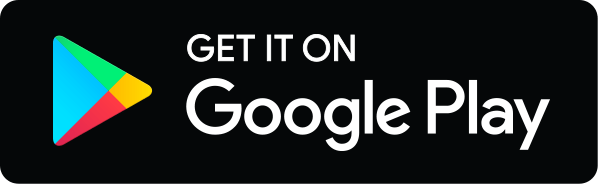