Fig. 4.1
(a) Derivatives of the cardiac neural crest cells (CNCC). CNCCs originate in the dorsal neural tube and migrate to pharyngeal arches 3, 4, and 6 and into outflow tract to form the aorticopulmonary septum (AP). The AP septum divides the single outflow tract into the aorta (AO) and pulmonary trunk (P). CNCCs form a mesenchymal sheath around the aortic arch arteries and the connective tissue of the pharyngeal glands. (b) Schematic of the development of the paired aortic arch arteries and the subsequent remodeling to form the mature pattern of the great vessels (a–d). (a and b) Diagram of the bilaterally symmetrical aortic arch arteries that carry the early cardiac output to the dorsal aorta which then distributes blood to the mouse or human embryo. (c and d) The early symmetry is lost when these vessels are remodeled to be the adult great arteries of the thorax. The 3rd aortic arch arteries (green) form the right and left common carotid arteries (RCC and LCC). The left 4th aortic arch artery (red) forms part of the transverse arch of the aorta. The right 4th forms the base of the right subclavian artery (RSC). The left 6th aortic arch artery (purple) forms the ductus arteriosus (DA). The 7th dorsal intersegmental arteries (pink) form most of the RSC and left subclavian (LSC) arteries. Abbreviations: BC brachiocephalic artery, LVA and RVA left and right vertebral arteries, A aorta, P pulmonary trunk, DOA dorsal aorta, DC ductus caroticus (Adapted from [50, 51])
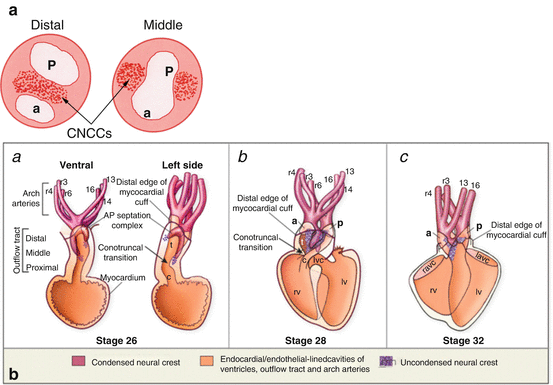
Fig. 4.2
(a) Cardiac neural crest cells (CNCCs) in the outflow cushions. Cross-section through the distal and middle outflow tract shows position of the condensed CNCC-derived mesenchyme within the cushions to form the future aorta (a) and pulmonary trunk (p). (b) Stages of outflow septation in the chick. (a) U-shaped septation complex straddles the aortic sac between the 4th and 6th aortic arch arteries. (b) The septation complex grows at the expense of the prongs. (c) Septation of the conus (Adapted from Hutson and Kirby [50])
4.3 Signaling Pathways Regulating Early CNCC Migration
CNCCs require a wide variety of environmental signals in order to be specified, migrate, proliferate, differentiate, and survive as they leave the neural tube and migrate into the pharynx and the outflow tact. The complex molecular cascade required to establish the migratory and multipotent nature of neural crest cells is not fully understood. Neural crest cells form at the border of the non-neural ectoderm and the neural plate. Secreted extracellular signaling molecules such as Wnts, bone morphogenetic proteins (BMPs), and fibroblast growth factors (Fgfs) separate the non-neural ectoderm from the neural plate during neural induction [13]. For example, downregulation of Bmp signaling in the neural plate accompanied by an increase in Bmp signaling in the surface ectoderm results in the activation of the transcription factor Slug in the cells at the border between the two tissues. These cells become specified as neural crest. Induction is heralded by the expression of Pax3 and Zic family member 1 (Zic1) transcription factors in the dorsal neural tube followed by activation of neural crest specification genes such as snail family zinc finger (Snail), Slug, transcription factor AP-2 (AP–2), SRY (sex determining region Y)-box 9 (Sox9), and forkhead box (Fox) D in the neural crest cells [13].
Once induced, neural crest cells require another set of overlapping signaling pathways to leave the dorsal neural tube in a process called epithelial-to-mesenchymal transition (EMT). During EMT the cells lose their cell-cell contacts, reorganize their cytoskeleton, and acquire a motile phenotype to leave the dorsal neural tube. One of the downstream effectors of BMP signaling is Smad-interacting protein 1 (Sip1). Sip1, an E-box binding zinc finger transcriptional repressor expressed by premigratory and migrating cranial neural crest cells, is known to specifically repress E-cadherin in epithelial cells and thus repress cell-cell adhesion [14]. Downregulation of cadherins is important for EMT and migration from the neural tube. Thus, one of the major roles of BMP in the CNCCs is to activate Sip1, which suppresses expression of E-cadherin, allowing the cells to detach from their neighbors.
Release from cell-cell contacts with adjacent cells allows the CNCCs to interact in three dimensions with extracellular matrix components. Migratory neural crest cells are now “mesenchymal” in that they express the intermediate filament protein vimentin and are flattened cells with filopodia and lamellipodia that facilitate movement. CNCCs express a complex collection of integrins, which are receptors that mediate attachment between cells and/or the extracellular matrix. Integrins are important for cell signaling and can influence cell shape and mobility and regulate the cell cycle. In addition to a role in induction, Wnt signaling also plays a role in CNCC migration. Wnt1 is expressed in early migrating neural crest cells and is turned off as the cells migrate away from the neural tube. Mutations in the mouse DISHEVELLED2 gene, another member of the Wnt signaling pathway, result in some of the same cardiac defects seen after cardiac neural crest ablation, including persistent truncus arteriosus [15].
Once the neural crest cells leave the neural tube, they travel along distinct migratory pathways to specific targets. In conjunction with FGF signaling, members of the ephrin family of ligands and receptors, semaphorins and connexin 43, define specific routes for the CNCC migration. Ephrin signaling is likely more important for directing migration into the pharyngeal arches rather than guidance away from the neural tube as seen with the semaphorins. Semaphorins, a group of secreted ligands, and their receptors are also important players in the targeted migration of the CNCCs to the outflow tract of the heart. The semaphorin receptors plexin-A2, plexin-D1, and neuropilin-1 (Nrp1) are expressed by the CNCCs. Sema6A and 6B ligands have been shown to repel neural crest cells, while Sema3C attracts them. Sema3C is expressed in the outflow tract, whereas Sema6A and 6B are expressed in the dorsal neural tube and lateral pharyngeal mesenchyme [16]. This suggests that CNCCs are driven from the neural tube by Sema6A and 6B and attracted to their target, the outflow tract, by Sema3C. Downregulation of each receptor in neural crest cells by RNAi knockdown results in the failure of the cells to migrate into the cardiac outflow tract [16].
4.4 CNCCs and Pharyngeal Arch Artery Remodeling
As described above, the neural crest cells migrate into pharyngeal arches 3, 4, and 6 to participate in the development of the pharyngeal arch arteries. Specifically the CNCCs migrate between the pharyngeal ectoderm and endoderm, proliferate, and envelope the endothelial strands of the nascent aortic arch arteries. The pharyngeal arch arteries initially form as a bilaterally symmetrical series of arteries that connect the aortic sac to the paired dorsal aortas (Fig. 4.1). Remodeling of the pharyngeal arteries requires programmed asymmetrical expansion, regression, persistence, and change of relative position of the different vascular segments (Fig. 4.1). Pharyngeal arch arteries 3–6 are remodeled into the asymmetric great arteries, including the common carotid, definitive aortic arch, and the ductus arteriosus. The right and left common carotid arteries are derived from the right and left 3rd arch artery. The arch of the aorta is derived from the left 4th arch artery. The left 6th arch artery gives rise to the ductus arteriosus. The CNCCs form the smooth muscle tunica media of these arteries [17]. Interestingly, CNCC ablations studies show that CNCCs are not required for aortic arch artery formation but are required for their repatterning [7]. Defects in 4th arch artery remodeling include interrupted aortic arch (IAA), double aortic arch, and aberrant (retroesophageal) subclavian artery. Patent ductus arteriosus and proximal pulmonary artery hypoplasia arise from defects in the 6th arch artery.
Several signaling pathways have been implicated in aortic arch artery patterning. Mutations in the endothelin 1 (ET1) pathway in mouse models result in patterning defects of the great arteries and outflow despite normal migration of the neural crest cells [18–22]. Haploinsufficiency for the transcriptional regulator T-box (Tbx) 1, by contrast, affects the initial growth and patterning of the arch arteries, the 4th arch artery in particular. Vessel growth failure, poor smooth muscle differentiation as well as abnormal CNCC migration have all been proposed as factors contributing to the defects [23]. The transcription factor encoding gene Tbx1 is not expressed by the CNCCs but rather by the pharyngeal endoderm, ectoderm, and second heart field (see below). Loss of Tbx1 indirectly affects the neural crest cells by impacting tissue-specific gene expression and altering the environment through which the neural crest cells migrate [21, 24, 25]. This in turn influences signaling between the crest cells and surrounding tissues, ultimately leading to altered pharyngeal arch patterning.
4.5 CNCCs and Septation of the Cardiac Outflow Tract and Valvulogenesis
After CNCCs migrate into the caudal pharynx, a subpopulation of cells continues to migrate into the cardiac outflow cushions (Fig. 4.2). The cushions are cardiac jelly-filled ridges that spiral within the outflow tract. The outflow cushions are largely populated by two distinct groups of mesenchymal cells, depending on the proximal-distal location within the outflow tract. In the proximal or conal outflow tract, the cushions are populated by endocardial cells that undergo an EMT induced by the conal myocardium. These mesenchymal cells will form the bulk of the semilunar valve leaflets. CNCCs move into the distal or truncal cushions and form two condensed columns of cells that are joined by a shelf of condensed CNCCs at the aortic sac (Figs. 4.1 and 4.2). The horseshoe-shaped aorticopulmonary septation complex is positioned between the origins of the 4th and 6th pairs of arch arteries where it will divide the pulmonary and systemic circulation [27]. Septation proceeds as the aorticopulmonary septation complex elongates into the distal outflow tract at the expense of the prongs [8, 28]. This splits the common arterial trunk into the aorta and pulmonary trunk (Fig. 4.2). Once the aortic sac and truncal portion of the outflow is divided, the proximal outflow septum in the conus closes zipper-like from distal to proximal toward the ventricles. This is accomplished by the invasion of myocardial cells into the cushions in a process called myocardialization [29]. After division of the outflow tract, cushion mesenchyme is remodeled to form the aortic and pulmonary semilunar valves. Each valve has three cusps or leaflets. The valve leaflets receive a major contribution from the endocardial-derived mesenchymal cells. Neural crest cells are observed at the tip of the semilunar valve leaflets and have been shown play a role in remodeling and maturation of the valves [4, 30, 31]. However, it is unclear to what extent CNCCs play a role in initial valve formation.
4.6 Role of Interaction of CNCCs and the Secondary Heart Field
An unexpected finding from the CNCC ablation experiments in chick was that crest cells are required not only for outflow septation but also for normal heart looping. Abnormal looping is one of the earliest defects seen after cardiac neural crest ablation and can be observed days before the crest cells reach the outflow. Defective looping is caused by a failure of addition of outflow myocardium to the heart tube from the secondary heart field, a subpopulation of the second heart field [32]. During looping stages, the secondary heart field is located in the ventral caudal pharynx, just behind the outflow attachment to the pharynx. The secondary heart field-derived cells add to the outflow and lengthen heart tube to ultimately form the myocardium and smooth muscle at the level of the semilunar valves. Lengthening of the outflow tract is required for the proper alignment of the aorta and pulmonary trunk over the left and right ventricles. An abnormally shortened heart loop results in arterial pole malalignment defects, such as double outlet right ventricle (DORV) and overriding aorta [32]. Thus the looping defects observed after CNCC ablation suggest that CNCCs [26] are required for normal deployment of the second heart field.
As discussed above, outflow alignment and outflow septation involve distinct cell populations, the second heart field derivatives and CNCCs. The ablation studies suggest that both these cell populations must act together to form a divided outflow tract. Indeed, after CNCC ablation, proliferation in the secondary heart field is increased due to elevated levels of FGF8 indicating that the CNCCs influence FGF signaling in the pharynx [33, 34]. Conversely, inhibition of Notch signaling within the second heart field results in abnormal migration of CNCCs into the outflow tract, suggesting that a Notch-dependent signal from the SHF can modulate CNCC behavior [35]. Thus, coordinated morphogenesis of the two cell populations is essential to form the myocardium and the septation complex to divide the outflow tract.
4.7 CNCCs in Cardiac Innervation and Conduction
CNCCs form the entire parasympathetic innervation of the heart [1]. While the components of the cardiac conduction system are largely innervated by neural crest-derived cardiac ganglia, the essential components of cardiac conduction system are myocardial in origin [36, 37]. Lineage analysis showed that some cells of His-Purkinje fibers lack mesoderm posterior 1 homolog (Mesp–1) implying that some of these cells may be of neural crest in origin, but this may just reflect variation in Cre expression [38]. Retroviral studies in chick and Cre-lineage analysis in mouse show a contribution of neural crest cells to the inflow region near the cardiac conduction system [39, 40], but a neural crest contribution to the inflow has not been demonstrated in quail chick chimeras [8]. Another lineage analysis using a Wnt1–Cre mouse suggested that the neural crest cells contribute to the central conduction system [41].
Whether or not CNCCs contribute directly to the His-Purkinje conduction system, they are required for normal development and maturation of the cardiac conduction system. A large number of fibroblasts derived from the epicardium, endocardium, and neural crest, are found within the mature conduction system and that insulate the ventricular pathways. Following ablation of CNCCs in chick embryos, there is a delayed maturation of conduction system function stemming from a failure of the conduction bundles to condense [12]. This finding is supported by mouse studies in which deletion of Sp4 transcription factor (Sp4/Hf1b) in neural crest cells resulted in atrial and atrioventricular conduction dysfunction due to deficiencies in the neurotrophin tyrosine kinase receptor 3 (Ntrk3/trkC) [42].
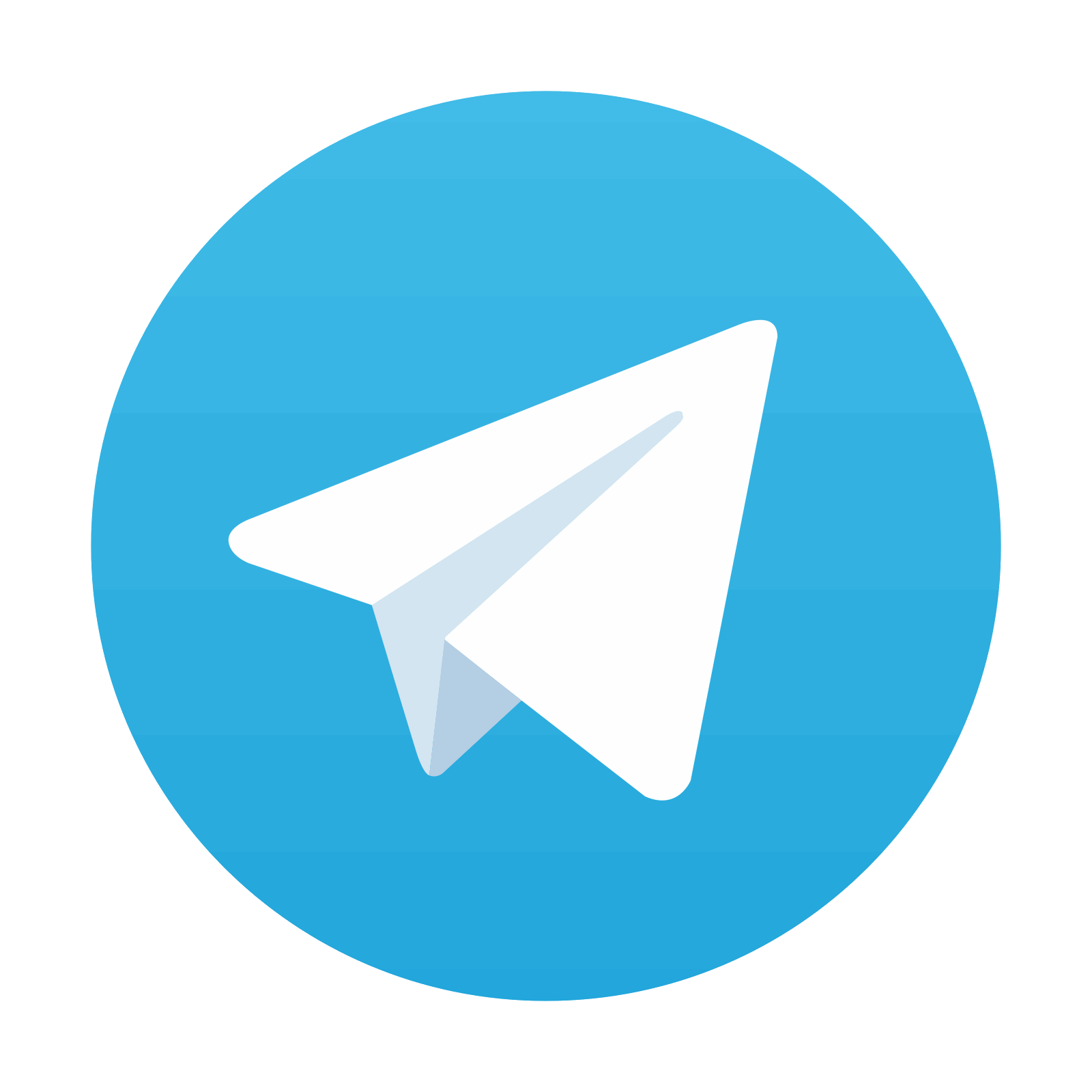
Stay updated, free articles. Join our Telegram channel

Full access? Get Clinical Tree
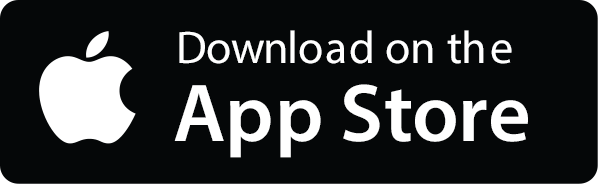
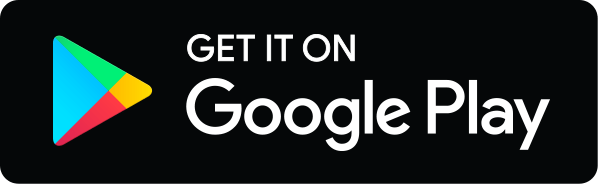