27
Natural sources – wildland fires and volcanoes
The distinction between natural sources of air pollution and other emission sources is not a clear one. We do not typically speak of ‘unnatural’ sources as distinct from ‘natural’ sources, but rather of anthropogenic (i.e. man-made) sources and natural sources. While most often ‘anthropogenic’ refers to pollution made up of products of combustion, crustal and other types of dust generated by human activities are also in a sense man-made and could be considered anthropogenic, although that is not common usage. It is important to understand that designating a source of air pollution as natural provides no assurance that these natural emissions are not harmful to health. Emissions from natural sources, as will be described in this chapter, contain components that are well known to be toxic.
The following specific types of pollution from natural sources will be considered in this chapter: smoke from wildfires, including forest, bush and grass fires; smoke from agricultural burning; and volcanic emissions. Smoke from agricultural burning, while not strictly natural, is included here to the extent that it provides insight into wildfire emissions and their effects. Biomass smoke will be used here to refer to smoke from wildland (forest and bush) fires and agricultural burning. Inhalation burn injuries or effects due to carbon monoxide intoxication will not be covered here.
Much is known about the health effects of individual components that make up biomass smoke and volcanic emissions. Much less is known about the effects of exposure to biomass smoke or volcanic emission in general, or about the contributions of the individual components in the respective pollutant mixes in producing these effects. Information from many sources will be used and integrated here in summarizing the health effects evidence. Some insight can be gained from knowing the individual components and concentrations that are present in these emissions. Toxicological studies employing exposures to emissions can provide insights into pathophysiological mechanisms and can serve to shore up plausibility of suspected effects. Ultimately, findings from experimental and epidemiological (observational) human studies are needed to understand the effects that actually result from these exposures. The study designs employed in the human studies will be briefly identified as they are encountered in this chapter, as will some of their strengths and shortcomings that influence how we interpret findings based on them.
This chapter begins with a review of biomass smoke exposure and its effects, followed by a review of volcanic emissions exposure and effects. Each review will conclude with a brief summary. A final section will address prevention of exposures and patient management. A small list of recommended readings is included at the end. Many of the studies referred to in this chapter review are referenced in those readings.
Exposure to smoke from wood and other biomass burning has been commonplace throughout human history. While smoke from forest wildfires is the most dramatic example of biomass smoke, bush and grass fires and controlled agricultural burns are also important outdoor sources of smoke exposure. The most prevalent source of biomass smoke exposure is indoor burning, with exposures resulting from personal wood burning for heating or biomass burning for cooking, or from neighborhood smoke resulting from residential burning. Effects of these more prevalent exposures will not be reviewed here, apart from brief mention in the context of extreme exposures below.
27.2.1 Extreme biomass smoke exposures
Health effects resulting from extreme exposures can serve to suggest effects that might potentially occur with more commonplace exposures. It should be kept in mind, however, that more commonplace exposures may never in fact have such severe effects.
Case reports involving intense exposures to products of wood combustion indoors have strongly indicated that these exposures have the potential to cause interstitial lung disease and asthma. ‘Hut lung’ has been used to describe cases of interstitial lung disease caused by chronic exposure to high levels of biomass smoke. Case-control studies of women in developing countries have provided good evidence that long-term exposure to the high concentrations of pollutants generated from burning biomass for cooking can produce chronic obstructive pulmonary disease (COPD) and associated pulmonary hypertension that can be as severe as those due to cigarette smoking. There is also evidence that these exposures in developing countries can cause lung cancer and increase the risk of active tuberculosis.
Forest wildfires are either caused naturally, most often from lightning strikes, or are started by people either accidentally or intentionally. In the USA, from 15,000 to 20,000 km2 (or 0.2% of the total land area) are burned each year. In Indonesia in 1997 and 1998, approximately 100,000 km2 burned. Smoke from forest fires often does not drift to areas where people will be exposed. When exposure does occur, it is most often on a small scale in small rural communities involving relatively few people. On occasion, especially with very large fires, smoke can drift over large urban areas exposing a large number of people to varying degrees and over varying periods of time. While the health impacts of smoke exposure on an individual are not obviously influenced by the size of the community affected, except perhaps through the potentially modifying effects of background urban air pollution, the ability to adequately study these impacts, and in particular the most adverse impacts such as mortality, is only possible when large numbers of people are exposed. Opportunities for studying these more severe effects are consequently limited.
Population density is increasing in areas prone to forest fires, such as in southern California, which is increasing the likelihood of significant population exposures. The apparent increased occurrence of large forest fires, possibly related to global climate change, and certainly related to the practice of using burning to clear large areas of forest, is also enhancing prospects for increased exposure.
Wood smoke constituents and exposures
Forest fire smoke, as is typical of smoke from any combustion process, especially an inefficient one, is a complex mixture of particles and hundreds of chemical compounds. Most of the particles are in the inhalable size range (PM10), by both number and by mass, and most of those are in the fine inhalable (PM2.5) size fraction. By far the largest numbers of particles are in the submicrometer size range. Particles this small settle poorly and can be transported in the air over several hundreds of kilometers before depositing. The particles are carbonaceous and include both elemental carbon (essentially soot) and organic carbon compounds.
Gaseous compounds in wood smoke include carbon monoxide (CO), nitrogen oxides and a host of hydrocarbons. Many of the hydrocarbons are potent respiratory irritants, such as acrolein, and many, including formaldehyde, benzene, and polycyclic aromatic hydrocarbons (PAHs), are either known or strongly suspected to be carcinogens. The relative proportions of the various compounds present in smoke both in the particulate and vapor phase is highly dependent on the type of wood burned and its water content, and the burning conditions such as whether a fire is smoldering or flaming. Less efficient burning, such as with a smoldering fire, produces less oxidized compounds.
Exposures to wildfire smoke are extremely variable, as would be expected, with the primary determinant being proximity to the fire. Particulate concentrations measured in areas where people are exposed can vary by more than two orders of magnitude. In close proximity, PM10 concentrations can reach several milligrams per cubic meter (i.e. thousands of micrograms per cubic meter), while dispersed smoke in urban areas may result in increases above background concentrations of only a few micrograms per cubic meter. CO can reach lethal concentrations immediately next to a fire and in smoke-filled spaces, but elevations in CO concentrations can also be barely detectable when smoke is dispersed.
Only a minority of forest fires result in significant nonoccupational (‘environ mental’) smoke exposure, and a much smaller number result in large populations being exposed. As a group, firefighters receive the highest concentrations and the longest durations of smoke exposure. Of the many constituents of wood smoke, personal concentrations of PM in firefighters when fighting fires are consistently the most elevated relative to occupational or ambient air standards. Personal peak concentra tions over 1 mg/m3 are regularly measured in firefighters. Because of the intense physical activity entailed in fighting fires, lung doses for a given air concentration would be expected to be considerably higher in these workers than in the relatively sedentary. See the section on wildland firefighters on the next page.
Wood smoke health effects
In Table 27.1, health effects are listed that might be expected on the basis of the constituents present in wood smoke. Later, in Table 27.2, a summary of the evidence that these expected effects actually result from exposure to wood smoke is presented, ordered from least to most adverse effects.
There is little controversy that exposure to wood smoke causes symptoms of irritation such as eye burning, throat soreness and cough. Anyone who has been exposed to wood smoke can personally relate experiencing these symptoms. It is reasonable to expect that individuals with underlying cardiopulmonary disorders, and even some without underlying illnesses, would be more sensitive to wood smoke effects and therefore experience more deleterious effects from wood smoke exposure than merely symptoms of irritation. Findings from properly conducted epidemiological and experimental health effects studies provide the basis for optimal recommendations for preventive measures and medical management.
The 2003 wildfires in Southern California provided an opportunity to study effects in a large population. Recently, the effects on children already enrolled in a large cohort study were reported. Children in this cohort experienced more respiratory symptoms with smoke exposure. Asthmatic children were not singled out as being more likely to experience worsened symptoms than children without asthma. Doctor visits prompted by symptoms were increased in those who reported more smoke exposure, but not in those living in areas with higher concentrations of PM mostly from wood smoke. Increased doctor visits for respiratory complaints were also seen during the 2003 wildfires in southern British Columbia. The increase in doctor visits was limited to the one of the two cities studied that experienced the highest PM concentrations, suggesting that a certain level of exposure may be required to trigger doctor visits.
Table 27.1 Constituents and potential health effects of wood smoke
Component | Health concerns |
Particulate matter (PM) | Wide ranging effects from respiratory symptoms to cardiopulmonary morbidity and mortality; effects of both short-and long-term exposure |
Carbon monoxide (CO) | Acute hypoxia; myocardial ischemia in coronary artery disease at low concentrations |
Nitrogen oxides | Pulmonary edema at high concentrations; possible airways effects at low concentrations |
Acrolein | Mucous membrane and respiratory irritant |
Benzene | Carcinogen (leukemia) |
Polycyclic aromatic hydrocarbons (PAHs) | Probable lung carcinogens (e.g. benzo[a]pyrene) |
Table 27.2 Evidence for health effects of biomass smoke exposure
Health outcome | State of evidence |
Irritant symptoms | Definite |
Impaired lung function | Suggestive in wildland firefighters |
Increased hospital visits | Best evidence for asthma |
Lung cancer | With indoor biomass burning in developing countries; possible from occupational exposure in the sugar cane industry |
Increased mortality | Possible, but evidence for an acute effect is mixed |
Earlier studies in California and Florida identified increases in emergency room visits for respiratory conditions in relation to wildfires. Increased hospitalizations were also reported during the Southeast Asia wildfires of 1997 and 1998.
Because of the wealth of evidence regarding short-term PM exposure effects on mortality, there would naturally be concern that wood smoke-related increases in PM have similar effects. Also, if hospitalizations are increased from smoke exposure, one might expect that some very susceptible persons would be even more severely affected. The evidence for mortality effects is mixed, however. Findings of studies of the Southeast Asia wildfires were inconsistent, but indicated that effects on mortality may have occurred. No effects on mortality were identified in a recent study of two episodes in which smoke from a wildfire drifted briefly over Denver, Colorado.
There is little information on acute cardiovascular effects of wood smoke exposure, effects expected in light of the cardiovascular effects of PM. No increase in cardiovascular doctor visits was seen in the 2003 southern British Columbia fires. One controlled, human exposure study using wood smoke generated from a wood stove has been performed to date. The most compelling finding was an exposure-related increase in serum amyloid A, an acute-phase reactant that reflects increased systemic inflammation and is a risk factor for atherosclerosis. There were no meaningful effects in this experimental study on coagulation factors, exhaled nitric oxide or an indicator of oxidative stress.
Wildland firefighters
The dramatically higher exposures to wildfire smoke experienced by firefighters would indicate that studies of smoke-related health effects in wildland firefighters might be ideal for identifying worst case scenarios. Unfortunately, some challenges encountered in studying firefighters have limited their usefulness in this regard. The physical demands of firefighting require a relatively healthy workforce. Studies in which no effects are identified can therefore be generalized to only a subset of the exposed population. Effects that are identified might be expected to underestimate effects experienced in a less healthy general population in settings of comparable exposure, but these settings would be unusual. Finally, the locations and rough terrain where firefighting activities are often performed limit the ability to assess exposure. Use of respiratory protection (respirators) by wildfire firefighters to reduce exposure is variable. The most effective protection is provided by atmosphere-supplying respirators, such as self-contained breathing apparatuses, but these are largely impractical. The least effective is the bandana, which provides only minimal protection against particles and essentially no protection against gases, but is nevertheless commonly used. Air filter masks that are more effective than bandanas against respirable particles are variably used. Powered air-purifying respirators are more effective than filter masks, but these are little used. Firefighter exposures to smoke occur both while fighting fires using some type of respiratory protection that is most often inadequate to protect against the many components of smoke, and while in the vicinity of fires when respiratory protection is not used. Study designs have therefore most often relied on indirect and crude measures of exposure such as a workshift in pre- and post-workshift studies, and a fire season in pre- and post-season studies.
Cross-season studies have shown increased symptom reporting, lower level of lung function and increased bronchial responsiveness after a firefighting season compared with those before the season. The rare cross-shift study has shown mean decreases in level of lung function over a workshift. One cross-sectional study of wildland firefighters done before the fire season was aimed at identifying effects of long-term smoke exposure. Levels in measures of lung airway function in this study were lower than those in control workers. Workers with more years of exposure, surprisingly, were not more severely affected than those with less exposure.
Assessing exposure-response relationships in cross-sectional studies such as this is hampered by the healthy worker effect in which the more healthy workers tend to preferentially continue working. This could make it appear that those who have worked the longest, and had the largest cumulative exposure, are actually healthier. In spite of the challenges in interpreting and applying findings from firefighter studies, evidence favors both short-term and long-term respiratory effects from the sometimes extreme exposures that this work entails.
Evidence from toxicology
Toxicological studies serve not only to investigate mechanisms underlying effects of smoke exposure, but to enhance the plausibility of epidemiological findings. Very high dose, acute inhalational studies, although relevant in indicating the types of effects that could occur with smoke inhalation, do not offer much insight into effects at the levels of exposure investigated in most epidemiological studies. Some lower dose studies have been done, however. Animal studies in which carboxyhemoglobin levels did not exceed 20% have demonstrated alterations in pulmonary immune defense mechanisms, specifically in macrophage function, and reduction in pulmonary clearance of bacteria. No pulmonary inflammation, as reflected by increased pulmonary inflammatory cell infiltration or increased lung cytokines, is typically observed at those levels of exposure. There is evidence for reduced lung antioxidant activity, however. There is also some evidence of smoke-induced exacerbation of allergen allergic responses, such as increased allergen-specific serum IgE and increased pulmonary eosinophils, which may be relevant to the epidemiological observations regarding smoke exposure and asthma. Forest fire smoke instilled in rat lungs produces little inflammation compared with other combustion sources.
Little evidence for cardiac effects or lung tumors has been found in relevant toxicological studies of wood smoke exposure. Wood smoke is mutagenic, however. Although somewhat dependent on burning conditions and type of wood burned, the potency of wood smoke in initiating skin tumors, a test of general tumor producing potential, is less than gasoline or diesel exhaust, but greater than cigarette smoke, which is typically less potent than other combustion sources.
27.2.3 Bush and grass fires and agricultural burning
Bush and grass fires, and agricultural fires, most of which involve burning of agricultural straw and stubble, can be reasonably discussed together. Increases in PM, CO and volatile organic compounds have been measured in relation to these types of burning, as they have for forest fires. Unlike for forest fires, it is not unusual for smoke from these fires to drift over large urban areas, thereby providing an opportunity to investigate effects in population studies.
A series of relatively small studies from Sydney, Australia largely showed no effects of bush fire smoke on respiratory emergency room visits or on level of lung function in asthmatic children. In contrast, in a larger time series study in Darwin, an association between PM concentration and asthma emergency visits was detected during a period of near-continuous bushfires. Time series studies, in which daily measures of smoke are related to daily counts of health events such as hospitalizations or deaths, are often used to study smoke effects. These studies are relatively easy to carry out as long as the relevant smoke data and counts of health endpoints are available. Interpretation of time series studies in assessing smoke effects relies on being able to identify burning-related pollutant concentrations or time periods. PM concentrations are often used as a measure of smoke exposure, which is reasonable if PM in the specific study setting is composed largely of wood smoke PM.
Number of acres burned has been used as a surrogate measure of agricultural burning in some time series studies. Emergency room visits for asthma have been associated with burn acreage in California and eastern Washington State. In Winnipeg, Canada, most of a cohort of subjects with mild to moderate airways obstruction reported increased respiratory symptoms with exposure to smoke from straw and stubble burning in surrounding fields; over one-third of this presumably susceptible cohort noted no increase in symptoms. Recently, relatively low concentrations of smoke from agricultural stubble burning in eastern Washington State did not affect either level of lung function or exhaled nitric oxide in asthmatics.
In rural Iran, however, rice stubble burning was associated with measures of worsened asthma control. Also, rice stubble burning in Japan has been associated with increased asthma emergency visits and hospitalization in children. One experimental human exposure study using controlled burning of rice stubble has been performed, and included only subjects with allergic rhinitis. No increase in inflammatory cells was seen in broncho-alveolar lavage fluid.
Effects of sugar cane burning have also been studied. Time series studies have suggested effects on intensity of hospital emergency nebulizer use in Brazil and, more recently, on asthma hospitalizations. Asthma hospital visits in Louisiana are higher in the sugar cane burning season.
There has been concern that occupational exposure in the sugar cane industry causes lung cancer and mesothelioma, specifically exposure to the amorphous silica fibers in sugar cane smoke. Evidence was determined to be inadequate for judging the carcinogenicity of sugar cane work in the IARC assessment on this in 1997, in spite of reports of increased lung cancer risk from several case-control studies. A subsequent case-control study from India also identified increased risk of lung cancer. Although anecdotal cases of mesothelioma have been reported in sugar cane workers, case-control studies have not supported this anecdotal impression.
27.2.4 Biomass burning summary
There are many challenges to rigorously studying health effects associated with exposure to smoke from forest fires, bush fires and agricultural burning. One of the greatest challenges is adequately estimating exposure to smoke. Exposure misclassifi cation is likely to be substantial when surrogate measures such as burning season and burn acreage are used. Even when concentrations of smoke pollutants such as PM are used, substantial spatial variability in concentrations is likely to be present, with attendant exposure misclassification. Also, when PM concentrations are not dramatically elevated during burning periods, PM may include significant contributions from nonburning sources. This limits the ability to attribute observed PM effects to burninggenerated PM specifically, especially in time series studies that attempt to relate shortterm concentration changes with changes in health. Use of PM measures that are specific for biomass burning would address this limitation, but specific measures have not been used in epidemiological studies.
In one commonly used study design, a measure of morbidity over a specified time period, hospital visits for asthma in September or October, for example, is compared across other time periods of the year. This design is particularly prone to bias due to seasonal trends in disease morbidity that may coincide with the burning season. The near-universal increase of asthma morbidity in the fall of each year, for example, often coincides with periods of burning. If these short-term temporal trends in the data cannot be accounted for, the more credible comparisons may be with morbidity measures at the same time period from other years when no burning occurred, such as was done in studying the wildfire smoke effects from the 2003 southern British Columbia fires described above.
In spite of these challenges in adequately estimating exposure and in using appropriate study designs, it is clear that exposure to smoke from biomass burning has health impacts. The types of impacts produced are known with varying degrees of certainty (Table 27.2). Some are known to occur only in specific exposure settings, but might be suspected in other settings as well. Regardless of the type of biomass burned, smoke produces symptoms of mucous membrane and airway irritation. There is good evidence that people exposed to smoke are more likely to seek medical attention manifested either as increased doctor visits, emergency room visits or hospitalizations; this evidence is particularly strong for asthmatics. It is not clear that effects on asthma are due to worsened airways inflammation; there is little suggestion from experimental studies that relevant exposures result in airways inflammation, although some data suggest enhancement of allergic responses.
Whether smoke exposure increases mortality is controversial at this point. Clearly intense acute smoke inhalation can be lethal. Also, arguing from the example of PM and its effects on mortality, unless PM in smoke is considerably less toxic than other sources of PM, smoke-induced mortality would be expected. Based on studies to date of large populations exposed to smoke, however, no firm conclusion regarding mortality is possible. Similarly, there is yet little evidence for cardiovascular effects of wood smoke exposure. However, based on the burgeoning amount of information on the cardiovascular effects of PM, cardiovascular effects of smoke would be anticipated. The human experimental finding of smoke-induced increase in serum amyloid A, an inflammatory marker and risk factor for atherosclerosis, supports the possibility of vascular effects.
Volcanic eruptions, exposures to volcanic emissions and their attendant health impacts have occurred repeatedly throughout human history. Reportedly, the most significant air pollution event related to a volcanic eruption in Europe in recent times was due to the eruption of Laki in Iceland in 1783. The resulting degradation in air quality in Europe during the summer months of 1783 was such that there was a distinct odor, visibility was reduced, effects on vegetation were apparent and human health impacts, consisting of irritation symptoms and respiratory illness, were reported. In North America, the Mt St Helens eruption in 1980 was important in focusing attention on the health effects of emissions from volcanic eruptions.
Rather than producing discrete air pollution episodes, volcanic emissions sometimes contribute to further degradation of already poor air quality, such as the contributions of emissions from Popocatepetl or the Miyake Island volcano on Mexico City’s and Tokyo’s air quality, respectively. In some cases, concern is focused more on gaseous volcanic emissions vented from a relatively quiescent volcano or due to soil gas in the vicinity of a volcano (‘degassing’), rather than eruptive emissions.
27.3.1 Composition of volcanic emissions
Emissions from volcanic eruptions contain pollutants in the particulate, vapor and gaseous phases. Emissions of air pollutants from volcanoes that occur between eruptions include similar pollutants. Gaseous pollutants include carbon monoxide, carbon dioxide, sulfur dioxide, hydrogen sulfide and radon. These can be present as gases in a volcanic eruption or produced in volcanic venting or degassing; degassing refers to the noneruptive emission of volatile compounds from volcanic magma in volcanic craters or through the ground. Acidic fumes (from condensed vapor) composed of hydrochloric acid, hydrofluoric acid and sulfuric acid can also be emitted primarily or formed secondarily from gaseous emissions.
Volcanic ash consists of particles of varying size, ranging from larger particles that settle quickly and are not inhalable, to fine inhalable particles. Inhalable particles (PM10) typically make up the largest number of particles but less than half of the particle (PM) mass. Fine inhalable PM (PM2.5) in volcanic ash, as distinct from typical urban ambient PM2.5, usually makes up only a small proportion of the PM mass. Silica is a prominent component of ash, especially in the inhalable fraction, but its contribution to total mass varies according to the type of volcanic activity. Crystalline silica, the form of concern in relation to silicosis, makes up much less of the mass. Volcanic PM also includes secondary compounds such as sulfate and various acid aerosols that form in the atmosphere after being emitted, although these can also be part of the primary emission mix. Heavy metals such as lead and mercury are also common particle components.
It is important to realize that not all volcanoes emit the same mix of pollutants in similar proportions, and that the same volcano may emit a different pollutant mix at different times. Health effects, to the extent that they are related to the composition of the emissions, would also be expected to be variable.
A large range of exposures to volcanic pollutants is expected based largely on marked differences in proximity of populations to volcanic emission plumes. It is estimated that at least 500 million people in the world today (~ 8%) live in areas where they could be exposed to emissions from volcanoes known to have been active in human times. Volcanic emissions from the often long periods between eruptions affect a much smaller subset of this population.
Health effects due to exposure to volcanic ash have been investigated using both observational and experimental approaches. There was a flurry of interest in the health effects of volcanic ash following the Mt St Helens eruption in southern Washington State in 1980. Short-term effects included increased emergency and hospital visits for respiratory symptoms and asthma. Pre-existing respiratory disease was a risk factor for adverse effects. Short-term ocular symptoms were also common. In vitro toxicology studies generally found toxicity similar to that of ‘low-toxicity’ minerals. Inhalational animal studies showed conflicting results, but those done using more realistic, although still high, concentrations of ash showed little toxicity. The ash was clearly less toxic than crystalline silica.
Other series of health studies have been carried out in relation to the Mt Sakurajima volcano in Japan and the Soufriere Hills volcano in Montserrat in the West Indies. Regular and frequent eruptions of the Mt Sakurajima volcano have chronically exposed the local population to volcanic emissions and ash since 1955. While no cases of silicosis have been detected, there is a slightly increased prevalence of respiratory symptoms related to exposure. Level of lung function in area loggers has not been associated with exposure. Eruptions of the Soufriere Hills volcano have resulted in frequent ash exposures of the resident population since 1995. Most of the crystalline silica in ash from this volcano is cristobalite, a particularly fibrogenic form. Prevalence of respiratory symptoms in children is higher in those from higher exposure areas of Montserrat. Inhalational studies using the Soufriere Hills ash in rats have shown more lung inflammation than with inert dust, but toxicity is considerably less than that of quartz. While no chest radiographic evidence of pneumoconiosis in island residents has been found, the latency period from onset of exposure is obviously short.
Exposure to volcanic gases also has the potential to be harmful. CO2 from volcanoes may be concentrated in high enough concentrations to cause asphyxiation. This can occur as a result of the sudden release of a CO2 cloud or from accumulation of CO2 in low-elevation areas where air circulation is limited, even at times when a volcano is quiescent. The evidence for deaths due to CO2 asphyxiation is largely indirect, being typically based on reports of large numbers of deaths in a defined area not due to other apparent causes, such as occurred around Lake Nyos in the Cameroon in 1986, and on some case reports. These reports are nevertheless compelling.
Hydrogen sulfide is another potential cause of asphyxiation from concentrated volcanic or geothermal releases. Like CO2, H2S can concentrate in low-elevation areas. If concentrations are high enough, the fraction of inspired oxygen could be critically reduced, leading to asphyxia. Unlike CO2, H2S in these situations probably causes its effects not by asphyxiation but by blocking the mitochondrial cytochrome chain, much like cyanide. Also, because H2S is lipid-soluble, effects are often localized to the central nervous system with manifestations of loss of consciousness or respiratory arrest. In those who survive exposure to high H2S concentrations, the clinical presentation could be one of pulmonary edema, adult respiratory distress syndrome or pneumonia. While the pneumonia is probably a chemical pneumonitis, bacterial pneumonia should be suspected if pneumonia develops several days after the incident. At low concentrations, H2S is a potent irritant to mucous membranes and the respiratory tract. The sulfurous rotten egg odor of H2S is apparent at much lower concentrations than produce irritant symptoms.
There has also been concern about effects of long-term exposure to much lower concentrations of H2S and other reduced sulfur compounds. The evidence is most convincing for neurological and psychological sequelae of long-term H2S exposure and for increased reported respiratory symptoms. As concerns other respiratory effects of H2S exposure, most evidence indicates no effect on level of lung function; evidence for increased respiratory hospitalizations and lung cancer is sporadic and inconsistent. An ecological study from Rotorua, New Zealand, a city affected by H2S emissions from a geothermal field, found evidence for neurological and respiratory hospitalization rates being increased in higher exposure areas, although there was also a suggestion that cardiovascular hospitalization rates were also increased. As in all ecological studies, because no data on individuals was available, there is concern that the observed associations could have other explanations than exposure to H2S.
Sulfur dioxide exposure can also be harmful, especially at high concentrations, where it is well known to cause bronchoconstriction in asthmatics. The evidence that high concentrations of volcanic SO2 specifically have been harmful is limited. As with other gases and fumes, it is has been difficult to implicate SO2 as the harmful component of what is often a mix of gases and fumes. However, episodes of degassing of SO2 have reportedly caused a small number of deaths in asthmatics. Volcanic acid fumes and acid aerosols, some generated from SO2, could potentially also contribute to adverse health effects, but evidence implicating them specifically is lacking.
27.3.3 Volcanic emissions summary
Both volcanic ash and gases from volcanic eruptions and degassing can cause effects on health. Exposure to ash most certainly causes ocular and respiratory symptoms and causes symptomatic individuals to seek medical care, especially those with pre-existing respiratory conditions such as asthma. Whether more severe effects of volcanic ash occur is controversial. Of particular concern is whether those chronically exposed to airborne ash are at risk of silicosis. While there have been no documented cases of silicosis caused by volcanic ash, silicosis is a theoretical possibility when risk is assessed on the basis of concentrations of crystalline silica and years of exposure. The health impacts of volcanic gases specifically appear to be due largely to the rare episodes in which very high concentrations cause death by asphyxiation, poisoning or severe exacerbation of asthma. While chronic exposure to gases is of concern, apart perhaps for neuropsychological sequelae of H2S exposure, the evidence for effects from long-term exposure to volcanic gases is weak (Table 27.3).
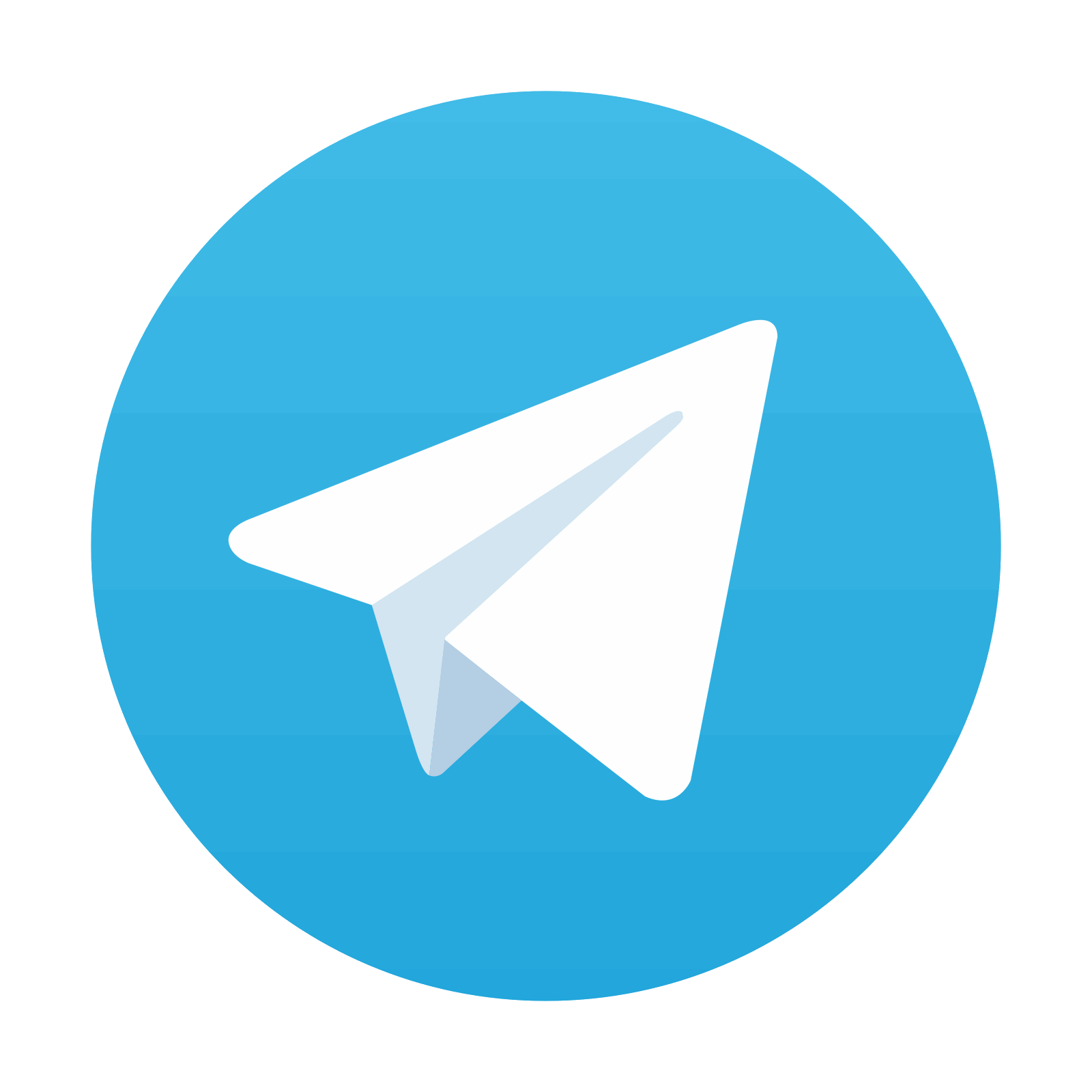
Stay updated, free articles. Join our Telegram channel

Full access? Get Clinical Tree
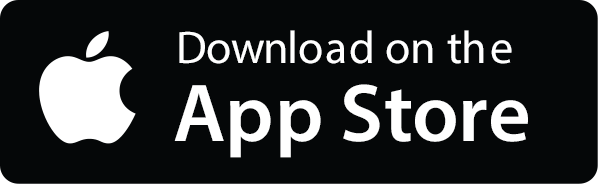
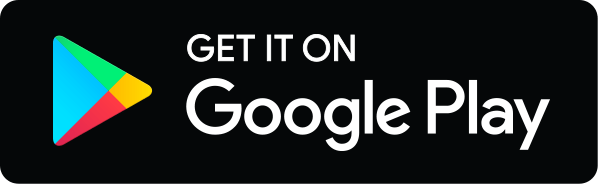