At its height, the success of surgical coronary revascularization spurred improvement in catheter-based technology—first for imaging quality, and later for attempted therapy. In 1974, Andreas Gruentzig completed the development of a double-lumen balloon catheter that was miniaturized for use in coronary arteries. Soon afterward, techniques for percutaneous transluminal coronary angioplasty (PTCA) expanded as technical breakthroughs were applied to subselective catheters, devices, guidewires, balloon materials, coronary stents, and circulatory support. Currently, trial evidence attests that percutaneous therapy is useful as a treatment in patients with poorly controlled angina whose anatomy does not imply a survival benefit from revascularization, and for emergency revascularization during ST-segment elevation myocardial infarction (MI). Surgical and percutaneous revascularization, however, cannot be considered equivalent.1
In the early balloon angioplasty era, several technical limitations restricted the use of percutaneous techniques to low-risk patients with proximal, discrete coronary artery stenoses, and procedural outcomes lacked predictability. As advances in tools and techniques were developed, higher-risk patients became candidates for percutaneous therapy. Over time, several principles for safety and success were recognized (Table 19-1).
|
Guiding catheters differ from diagnostic catheters in that a wire braid supports a thin catheter wall, allowing for a larger central lumen and providing enough rigidity to support the advance of subselective catheters to the distal regions of the coronary bed. The anatomy of the ascending aorta and the origin of the treated coronary artery determine which shape of guiding catheter will provide the most secure positioning (Fig. 19-1). The choice of guiding catheter often is the deciding factor for success when the arterial anatomy is challenging or when complications increase procedural difficulty. Guiding-catheter manipulation is a common cause of procedural complications that necessitate the urgent transition to coronary artery bypass surgery.
FIGURE 19-1
Commonly used guiding catheters are shown in the relaxed state (A) and as engaged with the coronary ostia in preparation for PCI: left Judkins (B), left Amplatz (C), XB (extra backup) (D), right Judkins (E), right Amplatz (F), and left coronary bypass (G). PCI = percutaneous coronary intervention. (Reprinted with permission from Cordis Corporation, a Cardinal Health company.)







A recent innovation in guiding catheter technology is the Guideliner®. The Guideliner is a device for subselective intubation of the coronary artery branches or saphenous vein grafts; its design borrows from that of the original Palmaz-Schatz stent platform. Subselective delivery of a catheter at or beyond the origin of a lesion substantially increases the probability of successful stent delivery. Most useful in delivering stents to the obtuse marginal saphenous vein graft, the Guideliner has been used for almost every difficult anatomic variant.2
When the guiding catheter’s position remains secure, the guidewire allows control of the distal vessel. Different wires vary in stiffness, coating, diameter, and design of the distal steering tip. For most procedures, the chosen wire is a 190- to 300-cm monofilament that is 0.0254 to 0.0356 cm in diameter, with either a graded tapering segment welded to the tip or a gradual taper of the monofilament. The central wire core at the tip is “plastic,” or malleable, and may be shaped by the operator. In many wire designs, a wire coil wrapped around the central filament projects a blunter, less traumatic tip to the vessel that it must traverse. Generally, the softest tip is the safest tip. However, in certain situations, such as treatment of a chronic total occlusion, a stiffer wire tip with a bonded, hydrophilic coating, rather than the wire coil, is frequently most effective. Although using these stiffer, “slicker” wires increases the likelihood of crossing the lesion successfully, the wires also increase the risk of complications, such as the creation of a subintimal wire course, the induction of dissection, or the perforation of the vessel (Fig. 19-2).
FIGURE 19-2
Support for subselective device introduction into the coronary vessels is determined primarily by the shape of the guiding catheter in relation to the anatomy of the ascending aorta and the origin of the left main coronary artery. Shown graphically, advance of the device forces the catheter backward. This movement is opposed by the alignment of the catheter with the coronary artery and by friction developing through contact between the primary curve of the catheter and the opposing wall of the aorta. (Reproduced with permission from Ikari Y, Nagaoka M, Kim JY, et al: The physics of guiding catheters for the left coronary artery in transfemoral and transradial interventions, J Invasive Cardiol. 2005 Dec;17(12):636-641.)

Most subselective devices for coronary artery manipulation are balloon inflation catheters or something similar. Balloon catheter designs vary in the placement of the proximal opening of the central lumen. The “on the wire” design has a central lumen that extends the length of the catheter. This design affords the best trackability and capability for maneuvering through difficult anatomy, but it requires an assistant to manipulate the wire during device advance. The “monorail” design has a central lumen, extending only through the distal balloon shaft of the catheter. The remaining catheter shaft communicates only with the balloon lumen. This design, though less trackable, allows the procedure to be performed without the need for an assistant.
Properties of the angioplasty balloon include compliance, maximally tolerated pressure, profile, and friction coefficient. Compliance, or growth under pressure, and maximally tolerated pressure are a function of balloon wall thickness and material. Compliance is divided into three categories depending on the growth of the balloon under pressure: noncompliant, semicompliant, and compliant. The thin-walled, compliant balloon has the lowest deflated profile, which allows the device to pass through the most severely occluded vessels. However, the balloon may not expand uniformly or lengthen when exposed to high pressures, such as pressures in excess of 20 atm (15,200 mm Hg), which are required to dilate hard and heavily calcified stenoses or stents. A variety of balloon coatings may be used to reduce the friction coefficient or protect the lumen from abrasion (eg, from passing a stent).
During an angioplasty procedure, blood may stagnate in the guiding catheter or near the treated lesion when a wire or device is placed within the lumen of the target lesion. In addition, metallic components of the guidewire or other devices attract fibrinogen, thus stimulating thrombosis. Therefore, a thrombus may form easily unless prevented with intense anticoagulation therapy (Table 19-2).3 Most angioplasty procedures are performed with unfractionated heparin (UFH) titrated to an activated clotting time (ACT) value of more than 300 seconds.4 Alternative anticoagulants, such as low-molecular-weight heparins and direct antithrombin antagonists, may be used.5-9 Direct thrombin inhibitors may be associated with reduced risk for major bleeding complications but are not reversible in the event that emergency surgical referral is needed.10 Antiplatelet therapy also reduces the risk of thrombosis at the treated lesion. In addition to aspirin and thienopyridines, glycoprotein IIb/IIIa (GP IIb/IIIa) complex inhibitors may be administered in specific circumstances. The GP IIb/IIIa complex inhibitors are unique in their ability to impair platelet-platelet aggregation, regardless of the type or intensity of stimulus. Anticoagulation is titrated to a lower intensity (an ACT of 200-250 seconds) when GP IIb/IIIa inhibitors are used.
Drug | Use | Setting | Dose | Duration of effect | Duration of therapy | Complications |
---|---|---|---|---|---|---|
Aspirin | Antiplatelet | All PCI | 81 mg | 5-7 days | Permanent | GI bleeding |
Clopidogrel | Antiplatelet | All PCI | 600 mg 6 h before PCI + 75 mg/day afterward | 5-7 days | 6 mo-1 year | Bleeding TTP (rare) |
Prasugrel3 | Antiplatelet | All PCI | 60 mg bolus + 10 mg/day | 72 h | 6 mo-1 year | Bleeding |
Ticagrelor3 | Antiplatelet | All PCI | 180 mg bolus + 90 mg 2/2 | 48 h | 6 mo-1 year | Bleeding Dyspnea |
Abciximab | Antiplatelet | ACS | 0.25 μg/kg bolus + 0.125 μg/kg/min | 72 h | 12 h | Bleeding Thrombocytopenia |
Eptifibatide | Antiplatelet | ACS | Two 180-μg/kg boluses (10 min apart) + infusion 2 μg/kg/min | 4 h | 12-72 h | Bleeding |
Tirofiban | Antiplatelet | ACS | 0.4 μg/kg/min for 30 min, then 0.1 μg/kg/min | 4 h | 12-72 h | Bleeding |
Heparin | Anticoagulation | All PCI | 100 IU/kg *60 IU/kg | 6 h | During procedure | Bleeding Thrombocytopenia Thrombosis |
Bivalirudin | Anticoagulation | UFH alternative | 1 mg/kg bolus + 2.5 mg/kg/h for 4 h | 2 h | During procedure + 4-6 h if no clopidogrel bolus | Bleeding |
Argatroban | Anticoagulation | UFH alternative | 350 μg/kg bolus + 25 μg/kg/min | 2 h | During procedure + 4-6 h if no clopidogrel bolus | Bleeding |
Enoxaparin | Anticoagulation | UFH alternative | 1 mg/kg *0.7 mg/kg | 6 h | During procedure | Bleeding |
Dalteparin | Anticoagulation | UFH alternative | 100 IU/kg *70 IU/kg | 6 h | During procedure | Bleeding |
Acetylcysteine | Contrast nephropathy prophylaxis | GFR < 60 mL/min | 600 mg every 12 h | Unknown | Begin 12 h before and continue for 12 h afterward | None |
Verapamil/diltiazem/nicardipine | Vasodilator | No-/slow-reflow | 0.1-0.5 mg IC | 20-30 min | As needed | Hypotension Bradycardia |
Nitroprusside | Vasodilator | No-/slow-reflow | 30 μg IC | 30-60 s | As needed | Hypotension |
Adenosine | Vasodilator | No-/slow-reflow | 50 μg IC | 30 s | As needed | Bradycardia |
Balloon angioplasty transmits increased intraluminal pressures circumferentially to the rigid intimal surface of the diseased vessel. Because atherosclerotic lesions typically are heterogeneous in their circumferential distribution and physical characteristics, the nondiseased or less diseased wall may be overstretched during balloon inflation. In most instances, the lesion segment with the greatest structural integrity is a focal point for applied stress. Adjacent regions of the vascular wall can shift, and the diseased, inelastic intima can fracture. Although this mechanism allows balloon expansion and an increase in luminal diameter, extension of the fracture into the intima-media border creates a dissection plane, the growth of which is determined by the mechanical characteristics of the lesion and the amount of force applied. If growth of the dissection plane results in significant displacement of the diseased intima, the vessel will close. This event, termed abrupt or acute occlusion, complicates about 10% of balloon angioplasty procedures. Overstretching the minimally diseased or nondiseased wall without causing plaque fracture typically results in early recoil of the treated lesion to its original state.
After balloon deflation, a small amount of thrombus accumulates, providing the stimulus and framework for colonization by inflammatory cells and myofibroblasts and the eventual, local synthesis of temporary (intimal hyperplasia) and permanent (collagen-rich) connective tissue. In addition, mechanical injury to the media and adventitia results in scar formation. The scar’s contracture may reduce vessel cross-sectional area—a phenomenon termed negative remodeling.11,12 During vascular healing, the encroaching intimal hyperplasia that peaks in volume at about 3 months, combined with negative remodeling, results in restenosis after 40 to 50% of balloon angioplasty procedures.13-15
In approximately 2 to 10% of balloon angioplasty procedures, intimal dissection, thrombosis, and perhaps medial smooth muscle spasm combine to produce abrupt closure.16,17 Abrupt closure may be treated successfully with repeat balloon inflation but is treated more commonly with stent implantation.18 The specter of abrupt closure and MI or emergency bypass surgery and its complications historically has limited the application of balloon angioplasty.
In patients with stable angina, mortality from balloon angioplasty procedures is 1% at 1 month.19 About half of the deaths are the result of a procedural complication, and most are related to low cardiac output (Table 19-3).20 Although the incidence of restenosis (>50% diameter stenosis during follow-up) is 40 to 50% within 6 to 9 months of a PTCA procedure,13,15,21 only 25% of patients report recurrent angina that warrants further investigation.22 Patients with restenosis have an increased risk of MI and needing coronary artery bypass surgery.23
Low-output failure | 66.1% |
Ventricular arrhythmias | 10.7% |
Stroke | 4.1% |
Preexisting renal failure | 4.1% |
Bleeding | 2.5% |
Ventricular rupture | 2.5% |
Respiratory failure | 2.5% |
Pulmonary embolism | 1.7% |
Infection | 1.7% |
The two failure modes of balloon angioplasty—abrupt closure and restenosis—have stimulated the development of a myriad of devices, all intended to reduce procedure-related risk, the risk of restenosis, or both. Only coronary stenting has been shown to be advantageous over balloon angioplasty alone, except in severely calcified lesions (Table 19-4). There are numerous coronary stent designs, but the majority of those in current use consist of a cylinder that is made from stainless steel (or an alloy, such as cobalt chromium) and that has been “carved,” creating a so-called slotted-tube design. Stent expansion creates a series of interlocking cells, resembling a cylindrical mesh-work (Fig. 19-3). Stents are thus deformable, but when expanded, they maintain sufficient rigidity to act as scaffolding after the angioplasty balloon is deflated. Intimal disruption is contained and far less likely to propagate and occlude the treated vessel. In addition, the rigid framework left behind becomes part of the vessel wall, addressing the issue of remodeling, which is one of the mechanisms of restenosis.
Experience | Ease of use | Complications | Efficacy | Lesion type | |
---|---|---|---|---|---|
Standard balloon angioplasty | ++++ | ++++ | + | +++ | Any |
Cutting balloon | + | ++ | ++ | +++ | Calcified lesion, ISR, bifurcation |
Rotational atherectomy | +++ | + | +++ | +++ | Heavily calcified, nondilatable ISR |
Directional atherectomy | + | + | +++ | + | Bifurcation, ostial lesion |
Laser atherectomy | ++ | ++ | ++ | ++ | Calcification, ISR, thrombus |
Aspiration (mechanical) | ++ | + | + | ++ | Thrombus |
Aspiration (manual) | + | +++ | + | ++ | Thrombus |
FIGURE 19-3
The coronary stent is a metallic “meshwork” that increases its rigidity when cold worked by balloon expansion. Buttressing of the vascular wall, propagation of dissection, and early vascular recoil are reduced significantly. (Used with permission from Texas Heart Institute, www.texasheart.org.)

Stents allow safe expansion of the vessel beyond that typically achieved with PTCA at the time of balloon expansion; however, stent use increases the thrombotic and inflammatory responses of the vessel wall. The increased injury and a foreign-body response to stent struts result in a more intense and prolonged local inflammatory response.24 Consequently, stent placement paradoxically exacerbates intimal hyperplasia.25,26 If one uses the late (6 or 9 months) loss in lumen diameter after stent implantation as a measure of intimal hyperplasia, even the most modern stent designs fall within a range of about 0.8 mm—more than twice the loss incurred after PTCA (0.32 mm). As a result, with regard to restenosis after angioplasty, the impact of stenting for percutaneous coronary revascularization (PCR) is rather small in comparison with that of PTCA.25,26 Intimal hyperplasia and restenosis risk are primarily functions of the size of the treated lumen on completion of the procedure, the length of the treated lesion, and the presence of unstable angina, hypertension, and diabetes mellitus (Table 19-5).27-29 Long-term follow-up studies indicate that a stent that does not reocclude during the first 6 to 9 months after implantation is not subject to late, rapid disease progression.30-34
By reducing the likelihood of abrupt closure requiring emergency coronary artery bypass surgery and of restenosis, stent-assisted angioplasty is more effective than routine balloon angioplasty for virtually any type of coronary artery lesion. Registry data describe a risk for emergency surgery of only 0.3 to 1.1% and a procedural mortality of less than 1%.35-38 The likelihood of procedural complications may be estimated on the basis of lesion characteristics (Table 19-6).39 Depending on lesion characteristics and the number of lesions treated, after 1 year, 5 to 10% of patients require coronary artery bypass surgery, and 15 to 20% undergo a second percutaneous coronary intervention (PCI) procedure.40-43 After 5 years, 10 to 15% of patients require another revascularization procedure because of the development of severe stenosis at an untreated site.34 Diabetes increases the risk for adverse outcomes by increasing the risk of restenosis and disease progression at untreated sites.
Strongest correlates | ||
Nonchronic total occlusion | ||
Degenerated SVG | ||
Moderately strong correlates | ||
Length ≥ 10 mm | ||
Lumen irregularity | ||
Large filling defect | ||
Calcium + angle ≥ 45 | ||
Eccentric | ||
Severe calcification | ||
SVG age ≥ 10 years | ||
Outcomes | ||
Group | Definition | Death/MI/Emergency CABG (%) |
Highest risk | Either of the strongest correlates | 12.7 |
High risk | ≥3 moderate correlates | 8.2 |
Moderate risk | 1-2 moderate correlates | 3.4 |
Low risk | No risk factors | 2.1 |
As noted previously in the description of the guidewire, iron components of stent struts attract fibrinogen and provide a site for platelet attachment and thrombosis. The increased risk of thrombosis at the treated site persists until endothelialization is complete. As a result, more intense antithrombotic therapy is required during the procedure and for up to 1 year afterward.44,45
Stents may be used as a drug-delivery system. However, rather than simply applying a drug to the stent’s surface from which it will dissipate quickly, drug delivery is controlled by using a surface polymer or by altering the design or the material used to construct the stent.46 This drug-delivery device, called a drug-eluting stent (DES), allows the drug to be applied at high concentrations at the site of interest and reduces the probability of systemic toxicity.
DESs reduce the primary determinant of restenosis by 50 to 100%, as determined by measuring late lumen loss after angioplasty (Fig. 19-4). Studies that examined the efficacy of the DES resulted in the introduction of new nomenclature for the follow-up endpoints. The most useful follow-up endpoint is termed target vessel failure (TVF), signified by cardiac death, MI, or repeat revascularization of the treated vessel. One year after the procedure, the risk of TVF is reduced from 19.4 to 21% with a bare-metal stent (BMS) to 8.8 to 10% with a DES.40,43,47
Reduced rates of repeat intervention after DES placement have been reported for every type of lesion studied except bifurcation lesions, for which the risk of restenosis remains significant, and the risk of potentially fatal early thrombosis is as high as 3.5% (Table 19-7).40,43,48-66 Although the use of a DES reduces the rate of failure of long-term treatment and of repeat procedures, this reduction does not translate to a reduced risk of procedure-related complications.67 In addition, incomplete healing, a response to the eluting polymer, or both confer an increased risk of stent thrombosis that extends beyond 1 year.68 As a result, after intervention with a first-generation DES or in a patient with acute coronary syndrome (ACS), dual antiplatelet therapy should be continued for at least 1 year in all patients and indefinitely in patients with complex lesions. Newer-generation DESs allow for more rapid reendothelialization and a slight increase in late loss without increasing the risk of in-stent restenosis. As a result, the duration of dual antiplatelet therapy can safely be reduced to less than a year. For clinically stable patients, only 6 months of dual therapy is required, which increases the number of patients who may safely receive a DES while facing the prospect of noncardiac surgery in the near future.69
Population | Endpoint | BMS (%) | DES (%) |
---|---|---|---|
Total40,43 | TVF | 20-24.1 | 9.9-10.8 |
Diabetes mellitus60 | MACE 9 mo | 27.2-36.3 | 11.3-15 |
Insulin-treated53 | MACE 9 mo | 31.5 | 19.6 |
Myocardial infarction65 | TVR 8 mo | 32 | 18 |
“Complex” lesions56 | TLR 12 mo | 29.8 | 2.4 |
Long lesion, small vessel51,61,62 | MACE 9 mo | 18.3-22.6 | 4-8 |
Small vessel48 | MACE 8 mo | 31.3 | 9.3 |
Bifurcation49,50,64,66 | TVR 6 mo | 13.3-38 | 8.6-19 |
Restenosis55 | TVR 6 mo | 33 | 8-19 |
Saphenous vein graft lesion52 | MACE 6 mo | 28.1 | 11.5 |
Before coronary stents came into routine use, abrupt closure owing to dissection could be treated with repeat balloon inflation and, if that was unsuccessful, with coronary artery bypass surgery. Prolonged balloon inflation generally was necessary for successfully restoring patency, but in the event of failure, transport for emergency surgery often was accompanied by severe ischemia of the treated territory. As a result, balloon catheters with a short third-lumen opening just proximal and distal to the balloon were developed. These catheters, or “perfusion balloons,” allowed prolonged balloon inflation with far less ischemia and could be used to ameliorate ischemia during transport for surgery after an unsuccessful procedure. Since the introduction of the coronary stent, the use of perfusion balloons has become rare.
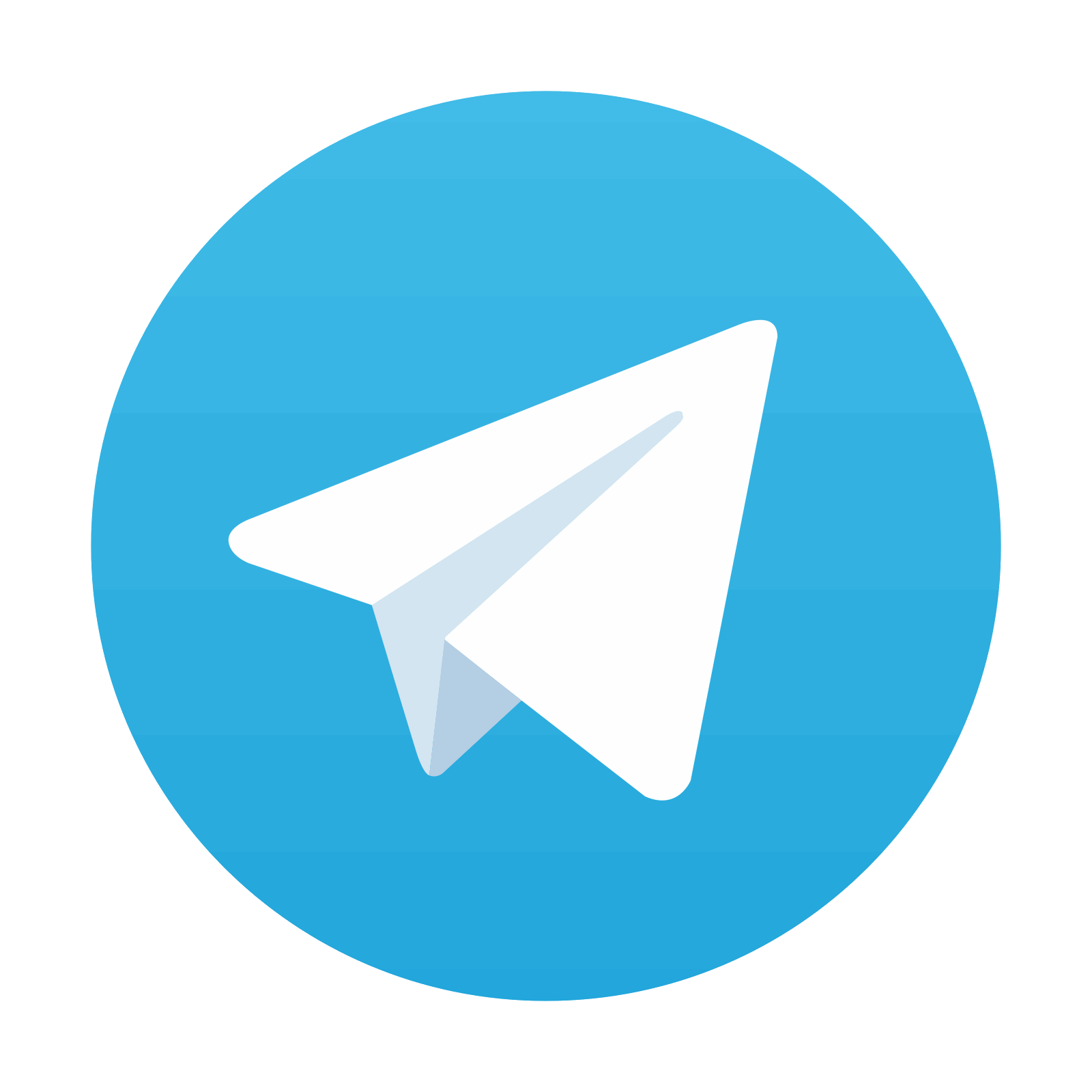
Stay updated, free articles. Join our Telegram channel

Full access? Get Clinical Tree
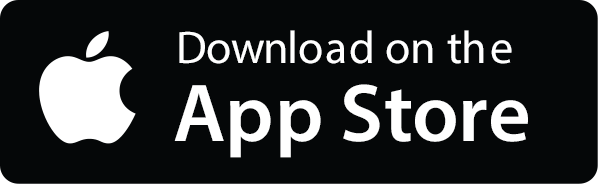
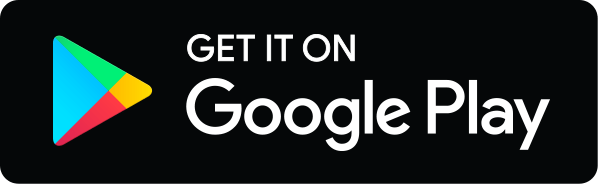