Acute myocardial infarction (MI) affects approximately 1.5 million individuals each year in the United States.1 Of these patients, 30% die before reaching the hospital and another 5% die during their hospital admission.1 Since 1998, the death rate from cardiovascular disease has declined 30.6%; however, cardiovascular disease remains the leading cause of death in the United States.2 Prompt medical attention including transport to the hospital, diagnosis, and treatment of the MI is critical to patient survival. Over the past 40 years, many developments have led to a decrease in the overall morbidity and mortality associated with acute MI. These developments include new pharmacologic agents, advancement in interventional cardiology procedures, modifications of coronary artery bypass surgical techniques, and the development of treatment algorithms and guidelines. Despite these advances, mechanical and electrical complications such as cardiogenic shock, rupture of the ventricular septum or free wall, acute mitral regurgitation, pericarditis, tamponade, and arrhythmias continue to challenge the medical community caring for patients presenting with acute MI on a daily basis.3 Of these complications, cardiogenic shock complicating acute MIs has the most significant impact on in-hospital mortality and long-term survival. The loss of more than 40% of functioning left ventricular mass and its accompanying systemic inflammatory response are the major causes of cardiogenic shock. Factors that impact the development of cardiogenic shock include the degree of pre-infarct ventricular dysfunction, the size of the infarcted vessel, and pathologic level of inflammatory mediators.4,5 Revascularization of threatened myocardium offers the best chance of survival following acute coronary occlusion, but the technique and timing of revascularization continue to evolve. The available therapies that can decrease the mortality associated with acute MIs include thrombolytics, percutaneous coronary intervention (PCI), and coronary artery bypass graft (CABG) surgery.
Myocardial ischemia resulting from coronary occlusion causes ischemic zone changes from a state of active systolic shortening to one of passive systolic lengthening within 60 seconds of onset of ischemia.6 Occlusions for less than 20 minutes usually cause reversible cellular damage and depressed function with subsequent myocardial stunning. Furthermore, reperfusion of the infarct results in variable amounts of salvageable myocardium. After 40 minutes of ischemia followed by reperfusion, 60 to 70% of the ultimate infarct is salvageable, but this decreases to approximately 10% after 3 hours of ischemia.7,8 Evidence from animal model has also demonstrated that 6 hours of regional ischemia produces extensive transmural necrosis.9 The exact timing in humans is more difficult to analyze because of collateral flow, which is a major determinant of myocardial necrosis in the area at risk in humans.8 The collateral blood supply is extremely variable, especially in patients with long-standing coronary disease. In addition, collateral flow is jeopardized with arrhythmias, hypotension, or the rise of left ventricular end-diastolic pressure above tissue capillary pressure.7 Thus, loss of collateral flow to the infarct area may lead to the cellular death of salvageable myocardium, and control of blood pressure and prevention of arrhythmias are vital during this time immediately after infarction.
Table 22-1 outlines the effects of anatomic, physiologic, and therapeutic variables on the evolution of final infarct size.
Anatomic |
Site of lesion |
Size of myocardium at risk |
Collateral circulation |
Physiologic |
Arrhythmias |
Coronary perfusion pressure |
Myocardial oxygen consumption |
Reperfusion injury |
Stunned myocardium |
Therapeutic options |
Medical management |
Revascularization |
Thrombolysis |
Percutaneous coronary angioplasty |
Coronary artery surgery |
Controlled reperfusion |
Buckberg solution and technique |
Mechanical circulatory support |
Disruption of coronary perfusion can result in three states of impaired myocardium: infarcted, hibernating, and stunned. Each state has a unique cause of injury and carries different prognostic implications (Table 22-2). Infarcted myocardium has suffered irreversible myocardial cell death owing to prolonged ischemia and will not benefit from revascularization.
Condition | Viability of cells | Cause of injury | Return of function |
---|---|---|---|
Infarcted | Nonviable | Prolonged ischemia | No recovery |
Stunned viable | Limited ischemia | Delayed with reperfusion | Recovery |
Hibernating | Viable | Ongoing ischemia | Prompt, sometimes unpredictable recovery |
Hibernating myocardium is a state of impaired myocardial and left ventricular function at rest resulting from reduced coronary blood flow and impaired coronary vasodilator reserve that can be restored to normal if a normal myocardial oxygen supply-demand relationship is reestablished.10 Hibernating myocardium is defined as contractility-depressed myocardial function secondary to severe chronic ischemia that improves clinically immediately after myocardial revascularization. This may be acute or chronic. Carlson and associates11 showed that hibernating myocardium was present in up to 75% of patients with unstable angina and 28% with stable angina. The entity also occurs after MI. Angina after MI commonly occurs at a distance from the area of infarction.12 In fact, mortality is significantly higher in patients with ischemia at a distance (72%) compared with ischemia adjacent to the infarct zone (33%).12 It is the hibernating myocardium that may be in jeopardy and salvageable, although its presence is usually incidental to the occurrence of the acute infarction. By distinguishing between hibernating myocardium and irreversibly injured myocardium, a more focused approach to restoring or improving blood flow to the area at risk is possible. Ventricular function often improves immediately after revascularization of appropriately selected regions.
Stunned myocardium is left ventricular dysfunction without cell death that occurs following restoration of blood flow after an ischemic episode. The phrase was initially coined by Braunwald and Kloner in 1982.13 If a patient survives the insult resulting from a temporary period of ischemia followed by reperfusion, the previously ischemic areas of cardiac muscle eventually demonstrate improved contractility. Stunning can be a fully reversible process despite the severity and duration of the insult if the cells remain viable. Within 60 seconds of coronary occlusion, the ischemic zone changes from a state of active shortening to one of passive shortening. Coronary occlusions lasting less than 20 minutes provide the classic model of the stunning phenomenon.14 The most likely mechanisms of myocardial stunning are listed in Table 22-3.14,15
Generation of oxygen-derived free radicals* |
Excitation-contraction uncoupling due to sarcoplasmic reticulum dysfunction |
Calcium overload |
Insufficient energy production by mitochondria |
Impaired energy use by myofibrils |
Impairment of sympathetic neural responsiveness |
Impairment of myocardial perfusion |
Damage of the extracellular collagen matrix |
Decreased sensitivity of myofilaments to calcium |
Stunned myocardium can occur adjacent to necrotic tissue after prolonged coronary occlusion and can be associated with demand-induced ischemia, coronary spasm, and cardioplegia-induced cardiac arrest during cardiopulmonary bypass. Pathologically, these regions are edematous, and even hemorrhagic, and can clinically present as both systolic and diastolic dysfunction.16 They also have a propensity for arrhythmias, which can lead to more extensive stunning and hypotension with subsequent infarction of these regions.
The primary distinction between stunned and hibernating myocardium is that in the former blood flow has been re-established after total occlusion whereas in the latter, coronary artery obstruction and reduced blood flow are still present.7
Mechanisms to identify patients with myocardial stunning and hibernation include electrocardiogram (ECG) findings, radionuclide imaging, positron emission tomography (PET), dobutamine echocardiography, and more recently magnetic resonance imaging (MRI). Thallium identifies perfusion-related defects of the myocardium and can distinguish between viable and scarred myocardium as well. However, early redistribution of thallium does not distinguish between hibernating and scarred myocardium because many segments with irreversible defects by thallium improve after reperfusion. Redistribution imaging and reinjection imaging improve the predictive value of thallium imaging in distinguishing hibernating myocardium.
PET measures the metabolic activity of myocardial cells. It has high positive and negative predictive values. It is now regarded as the best method to determine myocardial viability, particularly in patients with severe left ventricular dysfunction in whom other modalities are less accurate.17
Dobutamine echocardiography identifies hibernating and stunned myocardium by monitoring changes in segmental wall motion while the heart is stressed inotropically and chronotropically by dobutamine infusion. It has high specificity, sensitivity, and more importantly, positive predictive value.18
MRI has also been established as an effective method to assess hibernating myocardium.19 It has been proven to accurately diagnose the degree of both acute and chronic MI and predict functional recovery.20,21 A number of advantages exist with cardiac MRI, such as superior image resolution allowing accurate identification of transmural infarction (Fig. 22-1). By providing morphologic, functional, and metabolic information, it may ultimately supplant other modalities for the diagnosis of cardiac injury and recovery.
FIGURE 22-1
Cardiovascular MRI (CMR) characterization of myocardial infarction (MI) and post-myocardial infarction sequelae. Examples are shown of patients with myocardial infarction complicated by the presence of (A) microvascular damage (no reflow, purple arrows), and (B) right ventricular involvement (red arrows). (C) Acute infarcts (red arrows) can be differentiated from chronic infarcts by the use of T2-weighted imaging, which can show increased signal in areas of acute necrosis (green arrows). (D) Post-MI sequelae such as mural thrombus (blue arrows) can be identified by delayed-enhancement-CMR. Long-inversion-time imaging may improve detection because the image intensity of viable myocardium is gray rather than black. Thrombus is often immediately adjacent to infarcted myocardium (red arrows). (E) Acute pericarditis can be diagnosed by the presence of hyperenhanced pericardium (orange arrowheads). (F) CMR image may be used to define ventricular septal defect location (orange stars), extent of associated infarction (red arrows), and severity of shunting. (Reproduced with permission from Kim HW, Farzaneh-Far A, Kim RJ: Cardiovascular magnetic resonance in patients with myocardial infarction: current and emerging applications, J Am Coll Cardiol. 2009 Dec 29;55(1):1-16.)

Finally, multislice computed tomography has been used to measure hibernating myocardium, and early data suggest it may be a reliable and sensitive method compared with MRI, although its use in clinical practice is limited.22
Initial management of patients presenting with acute MI requires rapid diagnosis and assignment into the appropriate treatment algorithm. Treatment strategies should be directed toward reducing myocardial oxygen demand, maintaining circulatory support, and protecting the threatened myocardium before irreversible damage and expansion of the infarct occur.
Patients presenting with ongoing chest pain and ST-segment elevation in two contiguous leads or new left bundle branch block or anterolateral ST depression are classified as having ST-segment elevation myocardial infarction (STEMI). These patients are referred for primary revascularization, typically with fibrinolytics or percutaneous intervention in the cardiac catheterization suite, as immediately as possible assuming no contraindications exist. The other group of patients, those with non-ST-segment elevation myocardial infarction (NSTEMI), present with chest pain at rest for 10 minutes or more and ST-segment depression greater than 0.5 mm or ST-segment elevation 0.6 to 1 mm or T-wave inversions greater than 1 mm or positive troponin levels. Alternatively, there is a history of unstable angina in a patient with coronary artery disease risk factors. The initial management of these patients includes a combination of antiplatelet therapy, intravenous heparin, and other medications with a less urgent plan for cardiac catheterization. Both clinical and basic science research have demonstrated that reperfusion is the main treatment option for acute MI. Unfortunately, the majority of patients with MI receive only conservative medical management; only 40% of patients having an acute MI receive thrombolytic therapy, the most common means of reperfusion.23
Patients who present with STEMI can be further categorized by the presence or absence of cardiogenic shock. This distinction is important as it clearly predicts patient outcome, and it will impact treatment strategy. Cardiogenic shock can be defined as a systolic blood pressure of less than 90 mm Hg that is secondary to myocardial dysfunction. Clinically, it is associated with signs of hypoperfusion including decreased urine output, altered mental status, and peripheral vasoconstriction with cool extremities. Hemodynamic parameters consistent with cardiogenic shock include a cardiac index less than 2.2 L/min/m2, stroke volume index less than 20 mL/m2, mean pulmonary capillary wedge pressure greater than 18 mm Hg, and a systemic vascular resistance of over 2400 dyn-s/cm5. These patients are defined as type IV by the Killip classification system, a widely used system to classify MIs.24
Shock is the most common cause of in-hospital mortality after MI.25 The in-hospital mortality associated with cardiogenic shock has remained unchanged at approximately 80% despite the development of new treatment modalities.26 Since 1975, the incidence of cardiogenic shock complicating acute MIs has remained constant at 7.5%, ranging between 5 and 15% (Fig. 22-2).25 The progressive reduction in time delay from symptom onset to treatment may account for these constant figures. Previously, patients with excessive time delays would have died before reaching the hospital or soon thereafter, as treatment delays increase 1-year mortality exponentially (Fig. 22-2).27
FIGURE 22-2
Relationship between time to treatment and 1-year mortality, as a continuous function, was assessed with a quadratic regression model. Dotted lines represent 95% confidence intervals of predicted mortality. (Reproduced with permission from De Luca G, Suryapranata H, Ottervanger JP, et al: Time delay to treatment and mortality in primary angioplasty for acute myocardial infarction: every minute of delay counts, Circulation. 2004 Mar 16;109(10):1223-1225.)

Shock is directly related to the extent of the myocardium involved, and infarctions resulting in loss of at least 40% of the left ventricle have been found on autopsy in patients with cardiogenic shock.4,28 Autopsy findings also revealed marginal extension of the recent infarct and focal areas of necrosis in patients with cardiogenic shock.4 Extensive three-vessel disease is usually found in individuals with cardiogenic shock, and extension of the infarct is an important determinant in those individuals.4,28 Thus, limiting the size of the infarct and preventing its extension are the primary goals of therapeutic interventions in patients with MI.
Randomized trials have shown beneficial effects of early reperfusion within 12 hours and possibly up to 24 hours after acute MI.11,24,26,29 Early reperfusion clearly reduces infarct size in the major areas at risk, and controlled reperfusion may even be superior. The arguments are more difficult to make for patients outside the 24-hour window; however, patients with ongoing ischemia often have ischemic border regions that are prone to arrhythmias and necrosis. In addition, these patients are at risk for prolonged periods of hypotension with resulting end-organ injury and further left ventricular dysfunction. The benefit to early revascularization may be greatest in those patients with cardiogenic shock. Even if revascularization does not appear critical, ventricular unloading with intra-aortic balloon pump (IABP) or left ventricular assist device (LVAD) may provide the bridge to recovery needed in patients dying after MI. The primary factors limiting an aggressive surgical approach are major comorbidities, which make continuation of life undesirable or unlikely, and an unclear neurologic status, especially after a period of cardiopulmonary arrest.
Although restoration of blood flow to ischemic regions is essential, the accompanying reperfusion injury initially can worsen rather than improve myocardial dysfunction. The area at risk is affected not only by reperfusion but also by the conditions of reperfusion and the composition of the reperfusate.30 Thus, controlling reperfusion itself may aid in reducing myocardial infarct size and ventricular injury. At the cellular level, myocardial ischemia results in a change in energy production from aerobic metabolism to anaerobic metabolism. The consequences of ischemia vary from decreased adenosine triphosphate production and increased intracellular calcium to decreased amino acid precursors such as aspartate and glutamate. These changes can be reversed only by reperfusion. However, as oxygen is reintroduced into an ischemic region, oxygen free radical generation ensues with resulting cellular damage. Cellular swelling and/or contracture can lead to a “no-reflow phenomenon” that limits the recovery of some myocytes and possibly results in irreversible injury of others. The production of oxygen free radicals during ischemia, and at the time of reperfusion, is the leading mechanism proposed to explain cellular injury. Four basic types of reperfusion injury have been described: lethal cell death, microvascular injury, stunned myocardium, and reperfusion arrhythmias (Table 22-4).
Lethal | Cell death secondary to reperfusion |
Vascular | Progressive damage causes an expanding zone of “no reflow” and deterioration of coronary flow reserve during the phase of reperfusion |
Reperfusion arrhythmias | Arrhythmias, mainly ventricular, that occur shortly after reperfusion |
Stunned myocardium | Postischemic ventricular dysfunction |
Buckberg and coworkers31-36 conducted studies of controlled reperfusion after ischemia and produced a clinical application for controlled reperfusion. The surgical strategy of controlled reperfusion includes several elements. First, extracorporeal circulation is established as expeditiously as possible with venting of the left ventricle as required. Initially, antegrade cardioplegia is delivered using either a warm Buckberg solution to rebuild adenosine triphosphate stores or cold high-potassium cardioplegia to achieve rapid diastolic arrest. Retrograde cardioplegia can be added to ensure global cooling, even in areas of active ischemia. The temperatures of the anterior and inferior walls of the ventricle are measured to ensure adequate cooling. After each distal anastomosis, cold cardioplegia is infused into each graft and the aorta at 200 mL/min over 1 minute. This is followed by retrograde infusion through the coronary sinus for 1 minute. After completion of the final distal anastomosis, warm substrate-enriched blood cardioplegia is given at 150 mL/min for 2 minutes into each anastomosis and the aorta. After removal of the aortic cross-clamp, regional blood cardioplegia is given at 50 mL/min into the graft supplying the region at risk for 18 minutes. This controlled rate of reperfusion minimizes cellular edema and myocyte damage. The proximal vein grafts are then completed, followed by reestablishment of normal blood flow. To decrease oxygen demand, the heart is allowed to beat in an empty state for 30 minutes. After this time, the patient is weaned off bypass.
Application of the Buckberg solution and technique has been shown to be effective in improving mortality rates and myocardial function after acute coronary occlusion. With ischemic times averaging 6 hours, a prevalence of multivessel disease, and cardiogenic shock, the overall mortality in patients with acute coronary occlusion who underwent surgical revascularization applying this method of reperfusion was 3.9%. Postoperative ejection fraction averaged 50%.37 Surgical revascularization in this manner using controlled reperfusion compared favorably with percutaneous transluminal coronary angioplasty (PTCA) in several large series. These principles are reflected primarily in the surgical management of acute MI and will be outlined more specifically later in the chapter.
Once a patient who is a candidate for reperfusion therapy has been identified there are essentially three options available to the practitioner. They include thrombolytic therapy, primary PCI, and CABG. The intervention chosen for any particular patient will undoubtedly reflect the services that are available at the time of presentation, but they should also conform to the recommendations outlined by the ACCF/AHA guidelines including its most recent update in 2013.38 The following is a review of the available therapies, their roles, and the data that exist to support their use.
Because myocardial salvage depends on reperfusion of occluded coronary arteries, rapid dissolution of an occluding thrombus with thrombolytic therapy is an appealing intervention. Intracoronary streptokinase in patients with acute MI demonstrated that thrombolytic therapy is a safe and efficient way to achieve the desired early reperfusion.39 Following this study, a number of multi-institutional megatrials showed the effectiveness of thrombolytic therapy in treating acute MIs. The trial of the Italian Group for the Study of Streptokinase in Myocardial Infarction (Gruppo Italiano per lo Studio della Streptochinasi nell’Infarto Miocardio [GISSI])40 and the Second International Study of Infarct Survival [ISIS-2]41 found a reduced hospital mortality in patients treated with streptokinase. The effectiveness of tissue-type t-PA also has been evaluated in randomized studies. The Thrombolysis in Myocardial Infarction (TIMI) study42 and the European Cooperative Study Group43 demonstrated the effectiveness of t-PA for the treatment of acute MI. When streptokinase and t-PA were compared, two studies failed to demonstrate any difference in mortality.44,45 A third study, however, the Global Utilization of Streptokinase and Tissue Plasminogen Activator for Occluded Coronary Arteries (GUSTO) trial, supported the use of t-PA by demonstrating a more rapid and complete restoration of coronary flow that resulted in improved ventricular performance and reduce mortality.46,47
Although thrombolysis improves survival and ventricular function the patency of infarct-related arteries is reported to be between 50 and 85%.40-47 Normal flow should be achieved in 60% of patients by today’s standards. Thrombolytic therapy works well but is not without complications, including bleeding and intracranial hemorrhage.48 Bleeding is usually minor and occurs mostly at the sites of vascular puncture. Intracranial hemorrhage and stroke rates are approximately 1%. The relative benefits of thrombolytic therapy appear to decrease as patient age increases, and a higher risk of intracranial hemorrhage in the elderly may partially account for these findings.46,49,50 Careful selection of patients suitable for fibrinolyitc therapy is warranted, especially in an increasingly older population.
Thrombolytic therapy for patients presenting in cardiogenic shock or heart failure does not appear to improve survival in this population but may decrease the incidence of patients developing heart failure after MI.51
In summary, studies evaluating the effectiveness of thrombolytic therapy have demonstrated several useful points. First, survival is improved by decreasing time to reperfusion. The GUSTO trial showed that patients treated within the first hour had the greatest improvement in survival, with a 1% reduction in mortality for each hour of time saved.46,47 Thrombolytic therapy is easy to administer in the community by trained personnel, although a significant risk of bleeding exists in certain patients. Because the time to reperfusion is a critical element in preserving myocardium, thrombolytic therapy is ideal for most communities without percutaneous interventional capabilities. The importance of these elements is evidenced by two points made in the updated ACCF/AHA guidelines. First, in the absence of contraindications, fibrinolytic therapy should be administered to patients with STEMI at non-PCI-capable hospitals when the anticipated first medical contact (FMC)-to-device time at a PCI-capable hospital exceeds 120 minutes because of unavoidable delays.38 Second, when fibrinolytic therapy is indicated or chosen as the primary reperfusion strategy, it should be administered within 30 minutes of hospital arrival.38
Since the first reported PTCA by Gruntzig and associates52 in 1979, the efficacy of this percutaneous intervention in the treatment of coronary artery disease has been well recognized. A number of studies have evaluated the efficacy of primary PTCA in the treatment of acute MI. The overall PTCA hospital mortality rates range from 6 to 9%.53-56 Initial experience utilizing only balloon angioplasty was termed PTCA. One of the areas of technological advancement has been the development of new percutaneous therapies. These options include not only implantation of intracoronary stents, but also rotational atherectomy, directional atherectomy, extraction atherectomy, and laser angioplasty. In the current era, these techniques collectively are referred to as PCI. Here, we will use the term PTCA to relate data from studies limited to angioplasty, and the term PCI to accurately describe trials and studies that involve one or more of these additional techniques. This is consistent with the approach taken by the authors of 2001 ACCF/AHA Guidelines for PCI, which was a revision of the 1993 PTCA Guidelines.57
Several different strategies employing PCI for acute MI have been developed and examined through clinical trials. Primary, rescue, immediate, delayed, and elective PCI are options for the treatment of acute MI. Primary PCI uses percutaneous intervention as the method of reperfusion in patients presenting with acute MI. Rescue, immediate, delayed, and elective PCI are all done in conjunction with or following thrombolytic therapy. Rescue PCI is performed for recurrent angina or hemodynamic instability after thrombolytic therapy. Immediate PCI is performed in conjunction with thrombolytic therapy, and delayed PCI occurs during the intervening hospitalization. Finally, elective PCI is done following thrombolytic therapy and medical management when a positive stress test is obtained during the same hospitalization or soon thereafter.
Several studies evaluated the role of PTCA compared with thrombolytic therapy. The first study, the Primary Angioplasty in Myocardial Infarction Study Group trial in 1993, concluded that immediate PTCA without thrombolytics reduced occurrence of reinfarction and death and was associated with a lower rate of intracranial hemorrhage.53 Since then, more than 20 studies have compared PTCA to thrombolysis. The results from these studies have consistently and conclusively demonstrated the superiority of PTCA to thrombolysis, regardless of the thrombolytic agent used. The findings include a lower short-term mortality rate, lower rates of reinfarction, reduced stroke and intracranial hemorrhage rates, and a decreased composite end point of death, reinfarction, and stroke.58 These findings have been reconfirmed on long-term follow-up. A higher overall rate of bleeding was observed, likely because of vascular access complications. Myocardial salvage is similar for PTCA and thrombolytic therapy. However, primary PTCA may be slightly less costly than thrombolytic therapy.54
The use of intracoronary stents after MI has expanded as PTCA has become more prevalent. Benefits of stenting include lowered rates of restenosis and abrupt closure, and a reduced need for target revascularization after PTCA. Although the STENT-PAMI trail in 1999 using first-generation stents showed lower restenosis rates compared with thrombolysis, a trend toward higher mortality was seen, and its effectiveness as first-line therapy was questioned.59 Composite end points of death, reinfarction, and urgent target vessel revascularization at 30 days have now been shown to be lower in subsequent studies, such as the CADILLAC, ISAR-2, and ADMIRAL trials, which employed newer-generation stents.60-62 In these trials, a glycoprotein (GP) IIb/IIIa inhibitor, abciximab, was added to primary stenting. A significant reduction in restenosis rates with stenting plus abciximab versus PTCA alone was evident at 12-month follow-up in the CADILLAC study (41 vs 22%).60 More recent data suggest that stenting combined with antiplatelet therapy provides superior benefit to PTCA alone. Drug-eluting stents offer the potential to lower restenosis rates even further through the use of anti-inflammatory medication delivered via the stent. More recently, patients in the STRATEGY trial treated with drug-eluting stents after acute STEMI had a significantly lower composite end point of death, reinfarction, stroke, and angiographic evidence of restenosis at 8-month follow-up compared with bare-metal stenting plus abciximab (50 vs 19%).63 Using propensity score matching, retrospective analysis of patients post-acute MI in Massachusetts has shown a slight 2-year mortality benefit for drug-eluting stents when compared with bare-metal stents (10.7 vs 12.8%), as well as a lower need for repeat revascularization.64 Further prospective studies are under way to determine if long-term data confirm these findings.
The optimal pharmacotherapy for these patients continues to be debated and is an area of ongoing investigation. Initial experience with heparin was believed to be improved by the addition of GP IIb/IIIa inhibitors.65 However, routine use of GP IIb/IIIa inihibitors has fallen out favor, and current guidelines recommend limiting their use to high-risk situations. The HORIZONS-AMI (Harmonizing Outcomes with Revascularization and Stents in Acute Myocardial Infarction) trial66,67 compared bivalirudin, a direct thrombin inhibitor, with heparin plus a GP IIb/IIIa inhibitor for use after primary PTCA with or without stenting. The data disclosed decreased 30-day major bleeding events, death from cardiac causes, and death from all causes, despite a higher 24-hour risk of stent thrombosis in the bivalirudin cohort. Interestingly the mortality benefit persisted after 3-year follow-up. Since this trial, there have been several changes in management strategy including newer antiplatelets, increased radial access, and decreased use of GPIIb/IIIa inhibitors. A similar trial comparing bivalirudin to heparin plus GP IIb/IIIa inhibitors, the EUROMAX trial, demonstrated a decrease in incidence of major bleeding at 30 days but no difference in mortality or reinfarction.68 More recently, results of the HEAT PPCI (How Effective are Antithrombotic Therapies in Primary PPCI) trial were released. This was an open-label, randomized trial of STEMI patients which compared heparin with bivalirudin and used GP IIb/IIIa inhibitors only in bail out situations. Heparin was associated with a significant reduction in the major cardiovascular end points of all-cause mortality, cerebrovascular accidents, reinfarction, and target vessel revascularization (5.7 vs 8.7%, p = .01).69 In addition, there was no significant difference in the rate of major bleeding (3.5 vs 3.1%, p = .59). This study, along with others (NAPLES III, BRAVE IV), have raised significant questions regarding the benefit to using bivalirudin as opposed to heparin.70,71
Primary PCI may play a greater role in patients presenting in cardiogenic shock, as percutaneous interventions have become more common over the past 10 years (Fig. 22-3). The GISSI-1 and GISSI-2 trials demonstrated no benefit from intravenous thrombolysis, with morality rate of 70%.40,44 In patients presenting in or developing cardiogenic shock after acute MI, PTCA improved survival to 40 to 60%.72 This improvement was even greater when angioplasty was successful, as in-hospital survival rates increased to 70%. In most of these series an IABP was used in conjunction with PTCA. The SHOCK trial showed that revascularization by PTCA or CABG within 12 hours of the onset of cardiogenic shock results in improved 1- and 6-year survival rates versus medical stabilization followed by delayed revascularization (32.8 for PTCA/CABG vs 19.6% for initial medical stabilization, 6-year follow-up) in this high-risk group, particularly for those under the age of 75 years (Fig. 22-4).11,26,29 Subgroup analysis in patients undergoing successful PTCA or with TIMI Grade 3 coronary flow after PTCA in the SHOCK trial revealed that 1-year survival was even higher, at 61%.73 Independent predictors of mortality include age, hypotension, lower TIMI flow, and multivessel PTCA.
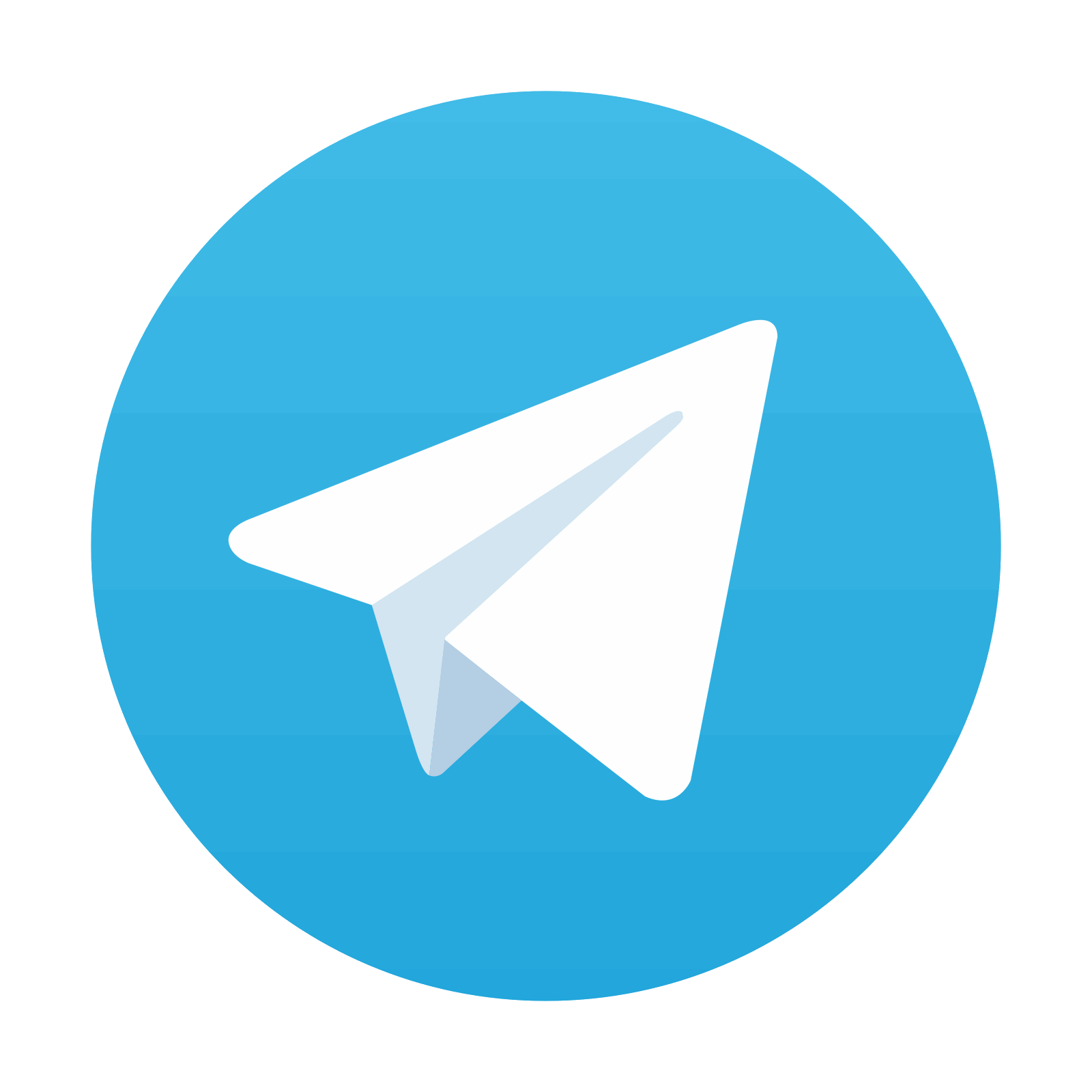
Stay updated, free articles. Join our Telegram channel

Full access? Get Clinical Tree
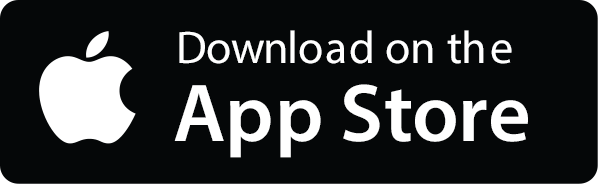
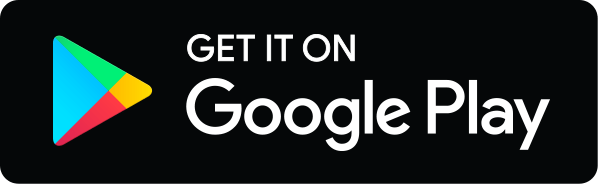