Myocardial protection in the operating room refers to strategies and methods used to attenuate or prevent perioperative infarction and/or postischemic ventricular dysfunction. In the setting of an acute myocardial infarction (MI) it refers to adjuvant therapy administered before, during or after reperfusion therapy. In transplantation it refers to the methods used to preserve the donor heart. Although the clinical situations are different, the goal of protection is the same, that is, to prevent or treat myocardial stunning and infarction. The underlying pathophysiology in all three settings is the same and relates to the etiology and consequences of ischemia/reperfusion (I/R) injury. After surgery the injury may manifest as low cardiac output, hypotension, and the need for postoperative inotropic support and ultimately mechanical circulatory support. I/R injury may be reversible (stunning) or irreversible (infarction) and is differentiated by electrocardiographic abnormalities (presence of a new Q-wave), elevations in the levels of specific plasma enzymes or proteins such as creatine kinase-MB and troponin I or T and/or the presence of regional or global echocardiographic wall motion abnormalities. Depending upon the criteria used, the incidence of postoperative MI in patients undergoing coronary artery bypass grafting (CABG) with cardiopulmonary bypass (CPB) ranges between 3% and 18%.1-4 While the majority of these are non-Q wave infarctions, they still are independently associated with adverse outcomes and prolonged aortic cross-clamp time and duration of CPB. Despite advances in techniques and cardioprotective strategies, the incidence of severe ventricular dysfunction, heart failure and death postoperatively ranges between 1% and 15%. The higher mortality rates are generally associated with high-risk patients with minimal cardiac reserve. These complications have an enormous impact on both families and society. From an economic standpoint alone, procedures for cardiovascular diseases are costly.
According to Heart Disease and Stroke Statistics–2015 Update,5 in 2010 an estimated 954,000 percutaneous interventions (PCIs), 397,000 CABG operations, 1,029,000 diagnostic cardiac catheterizations, 97,000 defibrillator implants and 370,000 pacemaker procedures were performed on inpatients in the United States. The estimated cost was $204.4 billion. Between 2013 and 2030, medical costs of coronary heart disease alone are projected to increase by 100%. Cardiovascular disease costs more than any other diagnostic group. Therefore, a reduction in perioperative complications associated with heart surgery would have a significant impact on resource utilization and overall operative costs.6-8 Since I/R injury is a major cause of morbidity and mortality after heart surgery, the purpose of this chapter is to review its underlying mechanisms, review the history of myocardial protection, update the reader regarding the current protective techniques and discuss new strategies under investigation.
The etiology of perioperative myocardial necrosis and postischemic myocardial dysfunction after cardiac surgery is multifactorial. Myocardial necrosis and elevation of associated cardiac biomarkers may arise as a result of ischemia due to intrinsic coronary disease unrelated to revascularization, anesthetic factors, atrial cannulation, suturing of heart muscle, plaque rupture or platelet embolism, and graft spasm or thrombosis. Despite the considerable progress made to date, high-risk heart surgery patients including those with unstable angina, poor ventricular function, diabetes, repeat CABG, and advanced age continue to experience postoperative complications such as low cardiac output, perioperative MI and heart failure requiring prolonged intensive care. In many of these instances the complications are due to I/R injury and inadequate myocardial protection. Thus there is a compelling unmet need to develop better methods to protect the heart during surgery.
Myocardial I/R injury manifests as postischemic “stunning”, which is reversible, and apoptosis and/or necrosis, which are irreversible. Myocardial stunning is an injury that lasts for hours to days despite the restoration of normal blood flow. Typically these patients require some type of temporary inotropic support in the immediate postoperative period in order to maintain an adequate cardiac output. Stunned cardiomyocytes exhibit minimal ultrastructural damage that resolves within hours to days following relief of ischemia. Apoptosis, or programed cell death, is a pattern of cell death that affects single cells. It is characterized by an intact cell membrane with blebbing, cell shrinkage, condensation of the nucleus and oligonucleosomal fragmentation of DNA. Externalization of phosphatidylserine on the outer leaflet of the plasma membrane serves as a cue for phagocytic removal of apoptotic cells by neighboring cells or professional phagocytes without inflammation. It is unclear whether apoptosis is initiated during ischemia and but manifests during reperfusion or whether it is a consequence of reperfusion, but the preponderance of evidence favors the latter.9,10 Regardless, apoptotic cell death occurs in the infarct zone and continues to occur in the border zone subsequently. Prolonged ischemia results in necrotic cell death characterized by cell swelling, plasma membrane rupture, mitochondrial swelling and loss of cristae, and DNA degradation followed by an inflammatory response to the cellular debris. Dying cells may exhibit features of both apoptosis and necrosis, that is, both nuclear condensation and plasma membrane damage.11-14
While the end result of inadequate myocardial protection is usually apparent in the immediate postoperative period, for example, low cardiac output syndrome, the full impact may not be evident for months to years. Klatte and colleagues reported that the 6-month mortality was greater in patients with increased peak creatine kinase-myocardial band (CK-MB) enzyme ratios after CABG surgery.15 The 6-month mortality rates were 3.4%, 5.8%, 7.8% and 20% for patients with peak CK-MB ratios that were <5, 5-10, 11-20, and >20-fold higher than the upper limits of normal (UNL), respectively. In another study, Costa and colleagues16 reported that normal postoperative CK-MB levels were observed in only 38.1% of 496 patients who underwent CABG surgery. When the CK-MB levels were stratified, the incidence of death at 30 days was 0.0%, 0.5%, 5.4%, and 7.0% when the enzyme levels were normal, 1 to 3 times higher, 4 to 5 times higher, and >5-fold higher than ULN, respectively. The 1-year mortality for these groups was 1.1%, 0.5%, 5.4%, and 10.5%. The peak postoperative cardiac enzyme level correlated significantly with worse clinical outcome. Thus, while CK-MB elevations are often dismissed as inconsequential in the setting of multivessel CABG surgery, they occur frequently and are associated with significant increases in both repeat MI and death beyond the immediate perioperative period. Consistent with this is the report by Steuer17 and associates in which they examined postoperative serum aspartate aminotransferase and CK-MB levels and their relationship to early and late cardiac-related death in 4911 consecutive patients who underwent CABG during a 6-year period. They reported that elevated enzyme levels on the first postoperative day greatly increased the risk of early cardiac death and were associated with a 40 to 50% increased risk in late mortality at 7 years.
In a retrospective analysis Brener et al showed that CK-MB elevation was common after both percutaneous and surgical revascularization.18 The incidence of CK-MB elevation above the normal range was 90% for CABG surgery and 38% for percutaneous coronary intervention (PCI). In a small fraction of patients the elevations exceeded 10 × ULN (6% for CABG and 5% for PCI). At 3 years follow-up the cumulative mortality was 8% for CABG and 10% for PCI. Even relatively small CK-MB elevations after interventions were associated with increased mortality over time. Similar observations were made when troponin release was used as a biomarker of myocardial injury. Lehrke et al reported in a series of 204 patients that a serum troponin-T concentration of 0.46 mcg/L or more, 48 hours after surgery was associated with a 4.9-fold increase in long-term risk of death.19
Finally, in one of the largest studies to date Domanski et al20 reviewed the relationship between peak postoperative levels of biomarkers of myocardial damage and early-, intermediate- and long-term mortality reported in previously reported and randomized clinical trials and registries. A total of 18,908 patients from seven studies were included for analysis. The follow-up ranged from 3 months to 5 years. The investigators reported that, in the aggregate, even modest elevations of troponin I at the 24-hour level were prognostically significant, and any CK-MB elevation after CABG was associated with increased mortality. In short, biomarkers of myocardial injury are frequently elevated after CABG surgery and are associated with decreased short-, medium-, and long-term survival.
The primary mediators of I/R injury include intracellular Ca2+ overload and oxidative stress induced by reactive oxygen species (ROS) generated at the onset of reperfusion21 (Fig.16-1). The molecule nitric oxide (NO) also can interact with superoxide (O2–) or peroxides to generate reactive nitrogen species that are capable of inducing injury as well.22 In addition, metabolic alterations occurring during ischemia can contribute directly and indirectly to Ca2+ overload and ROS formation. For example, decreased cytosolic phosphorylation potential, that is, [ATP]/([ADP] × [Pi]), results in less free energy from ATP hydrolysis than is necessary to drive the energy-dependent pumps (sarcoplasmic reticulum calcium ion adenosine triphosphatase (Ca2+-ATPase), the sarcolemmal Ca2+-ATPase) that maintain intracellular calcium homeostasis.23 With the onset of ischemia and the build-up of lactic acid, pH falls to as low as 6.6, resulting in proton efflux and sodium influx via the Na+-H+ exchanger. The driving forces for Na+/H+ exchange are the relative transmembrane N+ and H+ gradients. The activity of the exchanger is regulated by the interaction of the H+ with a sensor site on the exchanger protein; it can be additionally modulated by phosphorylation.24 During reperfusion the accumulated intracellular Na+ competes with Ca2+ for sites on the Na+/Ca2+ exchanger, effectively causing the exchanger to run in reverse, thereby exacerbating intracellular calcium accumulation. The elevated intracellular Ca2+ leads to activation of calcium-dependent proteases and phospholipases, calpain-mediated damage to myofibrillar contractile elements, gap junction dysfunction, injury of the sarcoplasmic reticulum and opening of the mitochondrial permeability transition pore (mPTP). Opening of the pore in the inner mitochondrial membrane is associated with a collapse of the membrane, uncoupling of the electron transport chain, release of cytochrome c and other proapoptotic factors, swelling of the matrix and ultimately rupture of the outer mitochondrial membrane and cell death.25
FIGURE 16-1
Myocardial alterations associated with ischemia-reperfusion injury. PKC, protein kinase C; Ca2+, calcium; [Ca2+]i, intracellular calcium concentration; [Na+]i, intracellular sodium concentration; GPRC, G-protein coupled receptor; Gs, stimulatory G proteins; PLC, phospholipase C; IP3 inositol trisphosphate; DAG, diacylglycerol; NHE, sodium-hydrogen exchanger; NCX, sodium-calcium exchanger; Na+, sodium; RP, reperfusion; NO, nitric oxide; mKATP channel, mitochondrial ATP-sensitive potassium channel; mPTP, mitochondrial permeability transition pore; ER, endoplasmic reticulum; [Ca2+]m, intramitochondrial calcium concentration.

The metabolic changes that occur during ischemia also deplete the endogenous antioxidant defense systems of cardiac myocytes. I/R damages mitochondrial complex I generating excessive ROS (O2– and H2O2) and resulting in damage to cellular lipids, proteins and nucleic acids. Glutathione is a critical defense against oxidative stress, in which GSH (reduced glutathione) is used to repair oxidized protein thiols, generating GSSG (oxidized glutathione) system; GSH is regenerated by glutathione reductase which depends upon the nicotinamide adenine dinucleotide phosphate, reduced form: nicotinamide adenine dinucleotide phosphate (NADPH:NADP+) ratio. Depletion of glutathione impairs the ability to detoxify ROS-mediated damage to proteins.26,27 During ischemia NADPH levels drop thereby limiting the ability to regenerate reduced glutathione. Thus, the formation of ROS during reperfusion occurs at a time when the myocyte’s endogenous defense mechanisms are depressed. The NADPH:NADP+ ratio is a primary determinant of the redox state of the cell, and there is evidence that the redox state plays a key role in determining the bioactivity and the redox state of NO.22,28 In addition, there are several reports that in the absence of normal levels of its cofactors, nitric oxide synthase (NOS), itself can generate the superoxide anion.29 Although systolic intracellular calcium concentration, [Ca2+]i, may decrease to normal levels early in the reperfused stunned myocardium, transient increases in [Ca2+]i can result in sustained activation of protein kinase C (PKC) isoforms, calpain proteases and deoxyribonuclease II (DNase II).30 Calpain-mediated proteolysis of contractile proteins has been implicated in the reduction of myofilament Ca2+ sensitivity observed in stunned myocardium.31
There is considerable evidence that ROS are involved in mediating myocardial stunning. Various spin-trap agents and chemical probes have demonstrated the rapid release of ROS into the vascular space during reperfusion after brief ischemia in vivo.32 While mitochondria are a major source of intracellular ROS in cardiac myocytes, they are not the only source of ROS during I/R.33,34 It has been shown that antioxidants administered before the onset of ischemia attenuate myocardial stunning in vitro and in vivo. Antioxidants administered prior to or at the onset of reperfusion may show efficacy, although there are contradictory studies.21,35 It has been shown that ROS can attack thiol residues of numerous proteins such as the sarcoplasmic reticulum Ca2+ ATPase (SR Ca2+-ATPase), the ryanodine receptor, and contractile proteins, which may explain why myofibrils isolated from in vivo reperfused stunned, but not necrotic myocardium, exhibit reduced Ca2+ sensitivity.36,37 Prolonged ischemia resulting in irreversible injury is associated with more severe intracellular Ca2+ overload and further depletion of endogenous antioxidants, conditions that both contribute to and are exacerbated during reperfusion by the production of ROS. Overproduction of ROS during reperfusion increases in [Ca2+]i through increased L-type Ca2+ channel current.27,38,39 Ca2+ overload with I/R exacerbates mitochondrial ROS production.39,40 Mitochondria can buffer small increases in intracellular Ca2+ via the Ca uniporter, a process that is energetically favorable owing to the [Ca2+] gradient and the mitochondrial membrane potential. During reperfusion the increase in cytosolic Ca2+ enhances mitochondrial Ca2+ uptake. Since excess cytosolic Ca2+ has been associated with the loss of myocyte viability, mitochondrial Ca2+ buffering is initially cardioprotective.41 However, continued mitochondrial Ca2+ uptake in the face of decreased antioxidant reserves and ongoing oxidative stress ultimately triggers opening of the mitochondrial permeability transition pore, a large conductance channel that allows release of stored mitochondrial Ca2+, osmotic swelling of the mitochondria, collapse of mitochondrial membrane potential and cell death. The synergistic interactions between Ca2+ overload and ROS formation during conditions of decreased antioxidant reserves also may explain why ROS scavengers are not very effective at reducing irreversible injury when administered at reperfusion.42 It also possible that antioxidants employed in studies to date are not targeted to the sites where ROS are generated and mediate most of their damage. Specific mitochondrial antioxidants may be more effective.43-45
Historically myocardial I/R injury has been characterized as either reversible or irreversible, based on staining techniques, enzyme release and histology. There is now increasing evidence that the transition from reversible to irreversible injury occurs on a continuum rather than an all-or-none phenomenon. Apoptosis depends on the availability of ATP and precedes loss of membrane integrity; however, if ATP depletion occurs rapidly, the Na+/K+-ATPase will be unable to regulate cell volume, resulting in plasma membrane rupture and necrotic cell death before the enzymatic processes of apoptosis are complete.46,47 Intracellular ROS and/or intracellular calcium overload can initiate the apoptotic program through proapoptotic BH3-only proteins Bnip3 (activated by ROS) and Bid (proteolytically activated by calpain).48,49 (Fig. 16-2) The BH3-only proteins trigger oligomerization of Bax or Bak directly or indirectly (through interaction with antiapoptoic Bcl-2 family members, Bcl-2, Bcl-xL, or Mcl-1), thus permeabilizing the outer mitochondrial membrane and allowing the release of cytochrome c into the cytosol.49-51 Formation of a cytosolic complex comprising cytochrome c, apoptotic protease activating factor 1 (APAF-1), and caspase-9 leads to proteolytic activation of caspase 3 and subsequent cleavage of multiple targets and activation of endonucleases and poly(ADP)-ribose polymerase (PARP). Proteolytic activation of PARP results in rapid consumption of adenine nucleotides and culminates in cell death. In parallel, intracellular ROS and calcium overload trigger mPTP opening and mitochondrial swelling; the mitochondrial F0-F1 ATP synthase runs in reverse hydrolyzing ATP in a futile effort to restore membrane potential across the inner mitochondrial membrane. ATP depletion compromises the ability to maintain ion and volume homeostasis via the Na+/K+-ATPase culminating in cell swelling, rupture of the plasma membrane and necrotic cell death. Myocardial damage can be further aggravated by the influx of macrophages and leukocytes, complement activation, and endothelial plugging by platelets and neutrophils.
FIGURE 16-2
Mechanisms of cardiomyocyte cell death following ischemia-reperfusion injury. Bax, proapoptotic family of proteins; Bcl-2, antiapoptotic protein; Ca2+, calcium; ROS, reactive oxygen species; Apaf, apoptotic protease-activating factor 1; PS, phosphatidylserine; SR, sarcoplasmic reticulum. Intracellular Ca2+ overload during ischemia and reperfusion and reactive oxygen species (ROS) formation during reperfusion are primary mediators of cell death via apoptosis and necrosis. The mechanisms of Ca2+ overload and ROS formation are described in detail in the text. In the intrinsic apoptosis pathway, BH3-only proapoptotic members of the Bcl-2 family are activated by calpain or caspase 8 (Bid) or ROS (Bnip3); they interact with Bax or Bak to form pores in the outer mitochondrial membrane allowing release of cytochrome c. This interaction is opposed by the antiapoptotic Bcl-2 family members including Bcl-2, Bcl-xL, and Mcl-1. In the cytosol, cytochrome c interacts with Apaf-1 and ATP or dATP to promote proteolytic activation of caspase 9 and the downstream executioner protease, caspase 3. Caspase 3 cleaves multiple targets, inactivating many cell survival pathways and activating endonucleases responsible for oligonucleosomal DNA fragmentation (DNA laddering). Cleavage of spectrin internal to the plasma membrane is permissive for phosphatidylserine redistribution to the external leaflet of the cell membrane, marking the dying cell for phagocytic removal by tissue macrophages or neighboring cells without an inflammatory response. In the necrotic death pathway, Ca2+ overload and ROS trigger opening of the mitochondrial permeability transition pore (mPTP), resulting in swelling of the matrix, disruption of the outer mitochondrial membrane, and rampant ATP hydrolysis by the ATP synthase running in reverse in a futile attempt to restore mitochondrial membrane potential. This energetic crisis leads to failure of the plasma membrane Na+/K+ ATPase, loss of plasma membrane integrity, and cell rupture, triggering an inflammatory response.

Detection of apoptosis based on DNA fragmentation (TUNEL assay), the final event in apoptotic cell death underestimates the extent of apoptosis. Detection of externalized phosphatidylserine with annexin V conjugated to a fluorescent dye allows earlier recognition of apoptosis and has been used for in vivo imaging of apoptosis in the mouse heart; however, not all cells recognized by Annexin V are irreversibly committed to cell death.52-55 Estimates of apoptosis in I/R injury are further confounded by the fact that the apoptotic remnants are efficiently cleared by neighboring cells or professional phagocytes. Thus the magnitude and significance of apoptosis in I/R injury has remained controversial. Genetic manipulations of mice have shown that disrupting the apoptotic machinery can result in a 60 to 70% reduction in infarct size. However, attempts to mitigate apoptosis with caspase inhibitors have not resulted in substantial reductions in infarct size, possibly because interruption of the late stages of apoptosis may simply result in necrosis. As a result, many investigators have turned their attention to interventions targeting the early events in apoptosis or preventing mPTP opening and necrotic cell death. Regardless of which stage is being addressed, current cardioprotection strategies are designed to reduce cellular and subcellular ROS formation and oxidative stress to enhance the heart’s endogenous antioxidant defense mechanisms and prevent intracellular Ca2+ overload.
In 1883, Ringer described the antagonistic effects of calcium and potassium ions on cardiac contraction. In 1929, Hooker reported the successful resuscitation of dogs with potassium in which ventricular fibrillation was induced by electric shock.56,57 In 1930, Wiggers reported that injections of potassium chloride were capable of abrogating ventricular fibrillation and arrested the heart in diastole. He also demonstrated that revival of the heart was possible using calcium chloride and massage.58 The work by Wiggers led Beck, a thoracic surgeon, to successfully apply defibrillation therapy and save a human life using this method.59 This resulted in a surge in basic and clinical research in the field of fibrillation and defibrillation with the principles applied to cardiac surgery.
With the advent of CPB, there was a need to refine techniques to protect the heart while creating a quiescent and bloodless field for the unhurried repair of intracardiac defects. Over the next 50 years, a variety of cardioprotective methods and techniques were introduced (Table 16-1). One of the first methodologies evolved from the concept of shielding the heart from perioperative insult using hypothermia. Bigelow and colleagues suggested using hypothermia “as a form of anesthetic” to expand the scope of surgery. The technique “might permit surgeons to operate upon the ‘bloodless heart’ without recourse to extra corporal pumps and perhaps allow transplantation of organs.”60 Five years later Melrose and colleagues reported another way to stop and restart the human heart reliably by injecting potassium citrate into the root of the aorta at both normal and reduced body temperatures.61 Soon thereafter, the clinical application of potassium citrate arrest was adopted by many centers. Interest in using the Melrose technique waned, however, with subsequent reports that potassium citrate arrest was associated with myocardial injury and necrosis. Within a short time, many cardiac surgeons shifted from using potassium-induced arrest to normothermic cardiac ischemia, that is, normothermic heart surgery performed with the aorta occluded while the patient was on CPB, intermittent aortic occlusion or coronary artery perfusion. Experimental and clinical evidence showed, however, that normothermic cardiac ischemia was associated with metabolic acidosis, hypotension and low cardiac output.62-64 As a consequence, there was a renewed interest in discovering ways to arrest the heart. Bretschneider published the principle of arresting the heart with a low-sodium, calcium-free solution.65 It was Hearse and colleagues however who studied the various components of cardioplegic solutions which led to the development and use of St. Thomas solution.66 The components of this crystalloid solution were based on Ringer’s solution with its normal concentrations of sodium and calcium with the addition of potassium chloride (16 mmol/L) and magnesium chloride (16 mmol/L) to arrest the heart instantly. The latter component was shown by Hearse to provide an additional cardioprotective benefit. In 1975, Braimbridge and colleagues introduced this crystalloid solution into clinical practice at St. Thomas Hospital.67 Gay and Ebert showed experimentally that lower concentrations of potassium chloride could achieve the same degree of chemical arrest and myocardial protection afforded by the Melrose solution without the associated myocardial necrosis reported earlier.67,68 Shortly thereafter, Roe and colleagues reported an operative mortality of 5.4% for patients who underwent cardiac surgery with potassium-induced arrest as the primary form of myocardial protection.69 In 1977, Tyers and colleagues demonstrated that potassium cardioplegia provided satisfactory protection in over 100 consecutive cardiac patients.70 By the 1980s, normothermic aortic occlusion had been replaced for the most part by cold blood cardioplegia to protect the heart during cardiac surgery. The major controversy at the time was not whether cardioplegic solutions should be used, but what was the ideal composition. The chief variants consisted of (1) the Bretschneider solution (containing primarily sodium, magnesium, and procaine); (2) the St. Thomas solution (consisting of potassium, magnesium, and procaine added to Ringer’s solution); and, (3) potassium-enriched solutions containing no magnesium or procaine (Table 16-2). Coincident with this controversy, another variant of cardioplegia was introduced, that is, the use of potassium-enriched blood cardioplegia.71,72 Theoretically blood cardioplegia would be a superior delivery vehicle based on its oxygenating and buffering capacity. Ironically, Melrose and colleagues had initially used blood as the vehicle to deliver high concentrations of potassium citrate more than 20 years earlier. While hypothermia and potassium infusions remain the cornerstone of myocardial protection today, a variety of cardioprotective techniques and solutions are used which allow patients to undergo heart operations with excellent 30-day outcomes.73,74
Reference | Year | Innovation |
---|---|---|
Bigelow WG60 | 1950 | Studied the application of hypothermia to cardiac surgery in canines |
Swan H75 | 1953 | Showed that hypothermic arrest (26°C) in humans provided a bloodless field for operating |
Melrose DG61 | 1955 | Introduced the concept of reversible chemical cardiac arrest in canines |
Lillehei CW76 | 1956 | Heart was protected by retrograde coronary sinus infusion of oxygenated blood |
Lam CR77 | 1957 | One of the earliest known uses of the term “cardioplegia” |
Gerbode F78 | 1958 | Used potassium citrate to induce cardiac arrest in humans |
McFarland JA79 | 1960 | Challenged the safety of the Melrose technique; changed from potassium arrest to intermittent aortic occlusion or coronary artery perfusion for myocardial protection |
Bretschneider HJ65 | 1964 | Developed a sodium-poor, calcium-free, procaine-containing solution to arrest the heart |
Sondergaard KT80,81 | 1964 | Adopted Bretschneider’s solution for clinical use |
Gay WA68 | 1973 | Credited with revival of potassium-induced cardioplegia; demonstrated that potassium solution could arrest a canine heart for 60 minutes without cellular damage |
Roe BB69 | 1973 | Demonstrated that “the modalities of cardioplegia, hypothermia, and capillary washout” provided effective myocardial protection |
Tyers WA62 | 1974 | Demonstrated in preclinical studies that an infusion of cold blood to maintain myocardial temperature <4°C provided 90 minutes of protection |
Hearse DJ66 | 1975 | Emphasized preischemic infusions to negate ischemic injuries in rats; this formula became known as St. Thomas solution no. 1 |
Braimbridge MV67 | 1975 | One of the first to use St. Thomas solution no. 1 clinically |
Effler DB82 | 1976 | Simple aortic clamping at room temperatures recommended |
Solarzano J83 Buckberg GD71 | 1978 1979 | Introduced concept of retrograde coronary sinus perfusion as adjunct to myocardial protection Blood is an effective vehicle for infusing potassium into coronary arteries |
Akins CW84 | 1984 | Hypothermic fibrillatory arrest for coronary revascularization without cardioplegia |
Murry CE85 | 1986 | First to report that brief periods of ischemia (preconditioning) and reperfusion enable the heart to withstand longer periods of ischemia in a canine model |
Lichtenstein SV86 Salerno TA87 | 1991 | Reported warm antegrade and retrograde blood cardioplegia safe |
Ikonomidis JS88 | 1995 | Combined normothermic continuous retrograde cardioplegia with intermittent antegrade infusions |
Teoh LK89 | 2002 | Introduced concept that intermittent cross-clamp fibrillation in CABG surgery patients confers cardioprotection via ischemic preconditioning and adenosine receptor activation |
Quinn DW90 | 2006 | Phase II human trial demonstrated efficacy of cardioprotective effects of systemic glucose-insulin-potassium (GIK) when administered perioperatively |
Mentzer Jr RM1 | 2008 | Phase III myocardial protection trial demonstrated proof of concept that perioperative MI can be reduced with an intravenous infusion of a pharmacologic agent in CABG surgery patients |
Cardioplegic surgery techniques utilize solutions containing a variety of chemical agents that are designed to arrest the heart rapidly in diastole, create a quiescent operating field and provide reliable protection against ischemia/reperfusion injury. While contemporary cardiac surgery in low-risk patients is relatively safe, patient characteristics have been changing over the past decade. In addition to coronary heart disease and poor ventricular function, patients are presenting with more comorbidities such as obesity, diabetes, renal dysfunction, peripheral vascular disease and emphysema. Despite ongoing improvements in cardioplegic techniques, low cardiac output syndrome (LCOS) still occurs after surgery and is a major factor associated with poor outcomes. In the absence of technical complications, the most common cause of postoperative LCOS is inadequate myocardial protection. For this reason, there is a real need to develop more effective strategies and novel additives to existing cardioplegic solutions. Currently there are two types of cardioplegic solutions that are used: crystalloid cardioplegia and blood cardioplegia. These solutions are administered most frequently under hypothermic conditions.
One of the first cardioplegic solutions used to protect the heart during surgery consisted of a cold crystalloid formulation. It was developed on the premise that its administration would protect the heart by reducing its metabolism and aid the surgeon by providing a bloodless field. Over the years a number of crystalloid cardioplegic solutions have been developed which contain different ingredients. The rationale for these ingredients has been based on the need to: (a) induce a rapid cardiac arrest using potassium or magnesium; (b) reduce energy demand and conserve ATP reserves using hypothermia; (c) maintain intracellular ionic and metabolic homeostasis; (d) lower myocardial oxygen consumption; (e) enhance anaerobic and aerobic energy production utilizing glucose and amino acids; (f) stabilize the pH using bicarbonate, phosphate, or histidine buffers; (g) stabilize cellular membranes by using steroids, oxygen free radical scavengers such as glutathione, calcium antagonists and/or procaine; (h) avoid intracellular calcium overload by providing a hypocalcemic environment and adding magnesium; and (i) prevent cellular edema by the addition of colloids such as mannitol to maintain normal oncotic pressures.
There are basically two types of crystalloid cardioplegic solutions: the intracellular type and the extracellular type.91 Both types can be used as preservation solutions for organ transplantation. The intracellular types are characterized by absent or low concentrations of sodium and calcium. The extracellular types contain relatively higher concentrations of sodium, calcium and magnesium. Both types avoid concentrations of potassium >40 mmol/L (typical range is 10-40 mmol/L), contain bicarbonate for buffering and are osmotically balanced. Examples of various crystalloid cardioplegic solutions are shown in Table 16-2.
Composition* | ||||||||
---|---|---|---|---|---|---|---|---|
Sodium | Potassium | Magnesium | Calcium | Buffer | pH | Osmolarity (mOsm/L) | Other Components | |
Intracellular crystalloid CP | ||||||||
Bretschneider’s no. 3 | 12.0 | 10.0 | 4.0 | 0 | Histidine | 7.4 | 320 | Procaine; mannitol |
Bretschneider’s HTK | 15.0 | 9.0 | 4.0 | 0 | Histidine | 7.3 | 310 | a-ketoglutarate; tryptophan; mannitol |
Roe’s | 27.0 | 20.0 | 3.0 | 0 | Tham | 7.6 | 347 | Glucose |
Extracellular crystalloid CP | ||||||||
del Nido solution†† | 140 | 5 | 0.75 | 0 | Bicarbonate | 7.4 | 375 | Lidocaine; mannitol |
St. Thomas no. 1 | 144.0 | 20.0 | 32.0 | 4.8 | None | 5.5 | 285 | Procaine |
St. Thomas no. 2 | 110.0 | 16.0 | 32.0 | 1.2 | Bicarbonate | 7.8 | 324 | None |
Tyer’s | 138.0 | 25.0 | 3.0 | 1.0 | Bicarbonate | 7.8 | 275 | Acetate; gluconate |
Blood CP† | ||||||||
Cold Induction | 118.0 | 18.0 | 1.6 | 0.3-0.5 | ± Tham | 7.6-7.8 | 320-340 | Glucose; oxygen |
Warm Induction | 122.0 | 25.0 | 1.6 | 0.15-0.25 | ± Tham | 7.5-7.6 | 340-360 | Glucose; oxygen; glutamate; aspartate |
While the degree of core cooling varies from center to center, patients undergoing CPB are cooled to between 33 and 28°C. To initiate immediate chemical arrest after cross-clamping the aorta, the solution is infused through a cardioplegic catheter inserted into the aorta proximal to the cross-clamp. The catheter may or may not be accompanied by a separate vent cannula. The cold hyperkalemic crystalloid solution is then infused (antegrade) at a volume that generally does not exceed 1000 mL. One or more infusions of 300 to 500 mL of the cardioplegic solution may be administered if there is evidence of resumption of electrical heart activity or if a prolonged ischemic time is anticipated. If myocardial revascularization is being performed, the aortic cross-clamp can be removed after completing the distal anastomoses and the heart reperfused while the proximal anastomoses are completed using a partial occlusion clamp. Alternatively, the proximal grafts can be performed after the distal grafts have been completed with the cross-clamp still in place (the single-clamp technique). Another approach is to perform the proximal aortic grafts first with a partial occlusion clamp and then cross-clamp the aorta and infuse the cardioplegic solution. When valve repair or replacement is being performed, the crystalloid cardioplegia can be administered directly into the coronary arteries via cannulation of the coronary ostia. Crystalloid cardioplegia also can be administered retrograde via a coronary sinus catheter with or without a self-inflating silicone cuff.
Although there is a concern that crystalloid cardioplegia lacks blood components and therefore has a limited oxygen carrying capacity, this has not been shown to be clinically relevant. Likewise while there is preclinical evidence that hyperkalemic crystalloid cardioplegia may damage coronary vascular endothelium and impair the ability of endothelial cells to replicate and produce endothelial derived factors, this has not been shown to be of clinical significance.92,93 In fact, there are numerous clinical studies which demonstrate that crystalloid cardioplegia is as effective as blood cardioplegia, particularly in centers where it is the primary form of protection.94,95
Cold blood cardioplegia, widely employed throughout the world, is the cardioplegic technique used most often in the United States. The rationale for using blood as a vehicle for hypothermic potassium-induced cardiac arrest is that it (a) provides an oxygenated environment and a method for intermittent reoxygenation of the heart during arrest; (b) limits hemodilution when large volumes of cardioplegic solution are used; (c) affords an excellent buffering capacity and osmotic properties; (d) allows for electrolyte composition and pH that are physiologic; (e) offers a number of endogenous antioxidants and free-radical scavengers; and (f) is less complex to prepare than other solutions.
Although there are a variety of formulations it is usually prepared by combining autologous blood, obtained from the extracorporeal circuit while the patient is on CPB, with a crystalloid cardioplegic solution that consists of citrate-phosphate-dextrose (CPD) buffered with tris-hydroxymethyl-aminomethane or bicarbonate and supplemented with potassium chloride. The CPD is used to lower the ionic calcium, and the buffer is used to maintain an alkaline pH at approximately 7.8. The final concentration of potassium used to arrest the heart is approximately 20 to 25 mEq/L. After the initial induction dose for rapid arrest, subsequent administrations may be intermittent or continuous and utilize a concentration of 8 to 10 mEq/L (the low concentration maintenance dose).96,97 (Table 16-2)
Prior to administering blood cardioplegia, the temperature of the solution usually is lowered with a heat-exchanging coil to between 12 and 4°C. The ratio of blood to crystalloid varies among centers with the most common ratios being 8:1, 4:1, and 2:1; this in turn affects the final hematocrit of the blood cardioplegia infused. For example, if the hematocrit of the autologous blood obtained from the extracorporeal circuit is 30, these ratios would result in a blood cardioplegia with a hematocrit of approximately 27, 24, and 20, respectively.
The use of undiluted blood cardioplegia, or “miniplegia” (using a minimum amount of crystalloid additives), also has been reported to be effective. Petrucci et al studied the use of all-blood miniplegia in a clinically relevant swine preparation and compared miniplegia with crystalloid cardioplegia. They concluded that the use of all-blood miniplegia was effective or superior in the acutely ischemic heart.98 Velez and colleagues used an acute ischemia/reperfusion canine preparation to test the hypothesis that an all-blood cardioplegia in 66:1 blood:crystalloid ratio would provide superior protection compared with a 4:1 blood cardioplegia delivered in a continuous retrograde fashion.99 They found very little difference between the animal groups with respect to infarct size or postischemic recovery of function. This is consistent with the findings by Rousou and colleagues years earlier; that, it is the level of hypothermia that is important in blood cardioplegia not necessarily the hematocrit.100
With respect to efficacy, there are numerous preclinical studies and nonrandomized and randomized clinical trials that demonstrate cold blood cardioplegia is an effective way to provide excellent myocardial protection. Many of these same studies have suggested that cold blood cardioplegia is superior to cold crystalloid cardioplegia. It is important to note that other investigators have shown crystalloid cardioplegia to be as cardioprotective and cost-effective if not more so. Unfortunately, most contemporary clinical trials comparing the efficacy of blood cardioplegia with crystalloid cardioplegia have involved single-center studies, enrolled a limited number of patients, focused on a specific subset of patients and/or omitted details regarding clinical management. In 2006, Guru et al reported the results of a meta-analysis of 34 clinical trials that compared blood cardioplegia to crystalloid cardioplegia. A lower incidence of low cardiac output syndrome and CK-MB release was observed in patients administered blood cardioplegia; no difference, however, was observed in the incidence of perioperative MI or mortality.101 Jacob et al (2008) analyzed clinical data from 15 randomized trials. Although eight of the trials reported statistically significant outcomes favoring blood cardioplegia, and five showed differences in enzyme release, the bulk of the evidence favoring one over the other was inconclusive.95
In 2014, Zeng performed a meta-analysis of 12 randomized control trials in which cold blood cardioplegia was compared to cold crystalloid cardioplegia.102 The trials accounted for 2866 patients: 1357 received cold crystalloid cardioplegia; 1509 received cold blood cardioplegia. The analysis showed no significant differences in mortality risk, incidence of fibrillation or stroke. The incidence of perioperative MI was lower however in the patients receiving cold blood cardioplegia. Regardless, the authors concluded that both crystalloid and cold blood cardioplegia are safe and effective for revascularization. The limitations of the study include the inherent problems associated with meta-analyses, and mortality was assessed only at the end of 30 days.
The concept of using warm (normothermic) blood cardioplegia as a cardioprotective strategy dates back to the 1980s. Proponents held that warm blood cardioplegic solutions would provide nutrients and endogenous factors that were absent in crystalloid solutions, and that normothermia would avoid possible adverse consequences of hypothermia. In 1982, Rosenkranz and colleagues reported that warm induction with normothermic blood cardioplegia, followed by maintenance of arrest with multi-dose cold blood cardioplegia, resulted in better recovery of function in canines than a similar protocol using cold blood induction.103 In 1986, Teoh and colleagues provided experimental evidence that a terminal infusion of warm blood cardioplegia before removing the cross-clamp (a “hot shot”) accelerated myocardial metabolic recovery.104 This was followed by a report in 1991 by Lichtenstein and colleagues that normothermic blood cardioplegia in humans is an effective cardioprotective approach.86 They compared the results of 121 consecutive patients undergoing CABG surgery who received antegrade normothermic blood cardioplegia to a historical group of 133 patients who received antegrade hypothermic blood cardioplegia. The operative mortality in the warm cardioplegic group was 0.9% compared with 2.2% for the historical controls. Despite these encouraging reports, there are concerns that for any given patient, it is difficult to determine how long a warm heart can tolerate an ischemic insult if the infusion is interrupted or flow rates are reduced owing to an obscured surgical field or maldistribution of the cardioplegic solution. Another concern is the report by Martin and colleagues that warm cardioplegia is associated with an increased incidence of neurologic deficits.52 In a prospective, randomized study conducted in 1001 patients, the efficacy of continuous warm blood cardioplegia with systemic normothermia (≥35°C) versus intermittent cold oxygenated crystalloid cardioplegia and moderate systemic hypothermia (≤28°C) were compared. While operative mortalities were similar (1.0 vs 1.6%, respectively), the incidence of permanent neurologic deficits was threefold greater in the warm blood normothermic group (3.1 vs 1.0%). Thus in this studywarm blood cardioplegia offered no advantage over cold crystalloid cardioplegia in terms of myocardial protection and carried with it the risk of increased ischemic injury if the oxygen supply to the warm heart was interrupted.
In 2014, DeBryn and colleagues performed a retrospective review of a prospectively collated database to compare the relative efficacies of using either antegrade warm blood cardioplegia (32°C) or antegrade cold crystalloid cardioplegia (7°C).105 The database included patient demographics, preoperative risk factors, operative technique, postoperative course and 30-day, in-hospital morbidity and mortality. The first 150 patients received cold crystalloid cardioplegia; the second group of 143 patients received cold blood cardioplegia. Overall the investigators found the clinical outcome for the patients was comparable and concluded that while warm blood cardioplegia is safe; it did not confer superior protection. Thus it appears that appropriately designed protocols using intermittent antegrade warm blood cardioplegia provide clinically acceptable myocardial protection.106-108
Both cold blood (4-10°C) and warm blood cardioplegic solutions (37°C) have temperature-related advantages and disadvantages. As a consequence, a number of studies were performed in the 1990s to determine the optimal temperature. Hayashida and colleagues were one of the first groups to study the efficacy of tepid blood (29°C) cardioplegia.109 In this study, 72 patients undergoing CABG were randomized to receive cold (8°C) antegrade or retrograde, tepid (29°C) antegrade or retrograde, or warm (37°C) antegrade or retrograde blood cardioplegia. While protection was adequate for all three, the tepid antegrade cardioplegia was the most effective in reducing anaerobic lactate acid release during the arrest period. These authors reported similar findings when the tepid solution was delivered continuously retrograde and intermittently antegrade.110 In contrast, Baretti et al reported in anesthetized open-chest swine placed on CPB that tepid normokalemic continuous antegrade blood cardioplegia was associated with an increased incidence of intermittent fibrillation.111 Subsequent to this report Mallid et al, in a retrospective chart review, analyzed the effects of cold blood and warm or tepid blood cardioplegia on early and late outcomes in 6064 patients who underwent CABG surgery. In the patients receiving warm or tepid blood cardioplegia (n = 4532), the systemic temperature was maintained between 32 and 37°C. The temperature of warm blood cardioplegia was 37°C. In the tepid cardioplegia cohort the systemic temperature was allowed to drift between 32 and 34°C; the temperature of the tepid cardioplegia ranged between 28 and 30°C. In the cold blood cardioplegia cohort (n = 1532) the systemic temperature was maintained between 25 and 32°C; the temperature of the blood cardioplegia ranged between 5 and 8°C. At 5 years, actuarial survival was 91.1% in the warm cardioplegia group and 89.9% in the cold blood cardioplegia group (p = .09). While this study has all the limitations associated with a large retrospective chart review conducted over the course of a decade, the findings are consistent with the basic conclusion that current methods of cardioplegia all show similar degrees of efficacy. To date, most tepid blood cardioplegia studies have been single-center studies conducted in relatively small cohorts of patients. Whether tepid cardioplegia confers superior protection over other methods remains to be determined. For the most part, however, the current methods of cardioplegia all show similar efficacy.
In addition to a variety of formulations and temperatures, there are also many different ways of administering the cardioplegic solutions (Fig. 16-3). As one might expect with so many options, the optimal delivery method also remains controversial. The various methods include intermittent antegrade, antegrade via the graft, continuous antegrade, continuous retrograde, intermittent retrograde, antegrade followed by retrograde and simultaneous antegrade and retrograde infusions. While all methods appear to be similarly efficacious, comparisons across trials are difficult because the studies also have differed with respect to: (1) the composition of the solution; (2) the temperature of the solution; (3) the duration of the infusion; and, (4) the infusion pressure. Additional factors that affect a surgeon’s choice for delivery method include the type and complexity of the operation and the anticipated cross-clamp time.
The most widely used method of delivering cardioplegic solutions is the antegrade method. It entails infusion of the solution into the aortic root while the ascending aorta is cross-clamped. After the initiation of CPB, chemical arrest can be achieved quickly with the infusion of the solution through a catheter inserted into the aorta proximal to the cross-clamp. The catheter may or may not be accompanied by a separate vent cannula to decompress the left ventricle between infusions. The induction dose usually has a higher concentration of potassium than subsequent maintenance doses. It is infused rapidly to ensure aortic valve closure usually at a rate of 250 to 300 mL/min (10 and 15 mL/kg body weight/min). If cardiac activity persists or recurs additional infusions may be necessary. For maintenance, infusions can be administered at 15 to 20 minute intervals or as needed. Concurrent external cooling can be achieved by suffusion with cold saline (4°C).
The typical aortic root perfusion pressure is maintained between 60 mm Hg and 80 mm Hg. Adjustments in rates of administration are made to accommodate patients with hypertrophied ventricles. Intermittent maintenance doses of 300 to 500 mL of low-dose cardioplegia are administered every 15 to 20 minutes or earlier if there is evidence of resumption of electrical activity. During CABG surgery, in order to minimize cold ischemia and aortic cross clamp time, the heart can be reperfused earlier by removal of the aortic cross-clamp as soon as the distal anastomoses are completed; the proximal anastomoses are then performed using a partial occlusion clamp. Alternatively the proximal grafts can be performed after the distal grafts have been completed with the cross-clamp still in place (the single-clamp technique).
The administration of antegrade cardioplegia depends on a competent aortic valve and hence is liable to be ineffective and relatively contraindicated in patients with significant aortic valve incompetence. In such cases, antegrade cardioplegia can be administered directly into the coronary arteries via cannulation of the individual coronary ostium. This technique is used in cases requiring the opening of the ascending aorta such as in aortic valve replacement, ascending aortic aneurysm repair and aortic root replacement procedures. Coronary ostial infusion may be necessary for induction in patients with severe aortic incompetence in the presence of coronary sinus pacing leads. Potential complications include coronary dissection, unintentional selective delivery into left anterior descending or left circumflex artery due to a short left main coronary artery, and the late occurrence of coronary ostial stenosis.112
Retrograde cardioplegia entails placement of a coronary sinus catheter with or without a self-inflating silicone cuff and infusion of the cardioplegic solution either for induction or maintenance of cardioplegia. This approach originated with a concept developed by Pratt in 1898, who suggested that oxygenated blood could be supplied to the ischemic heart via the coronary venous system.113 Sixty years later, Lillehei and colleagues used retrograde coronary sinus perfusion to protect the heart during aortic valve surgery.76 Today, it is an accepted method for delivering a cardioplegic solution and is used frequently as an adjunct to antegrade cardioplegia. Placement of the catheter is often facilitated by the use of catheters that are precurved and the simultaneous use of transesophageal echocardiography to guide placement. Care must be taken to avoid rupture of the coronary sinus, an uncommon but real and potentially fatal complication. This can be prevented by infusing the solution at a rate that ensures that the coronary sinus perfusion pressure does not exceed 45 to 50 mm Hg. The retrograde approach can be used for continuous or intermittent delivery of blood or crystalloid cardioplegia as well. In situations where the native coronary arteries are severely stenotic or totally occluded, the antegrade approach may result in an uneven distribution in the myocardium. In this situation retrograde infusion or infusion via the vein grafts as they are completed can be complementary. While most routine on-pump cardiac procedures can be done with good outcomes using just the antegrade technique, patients with poor ventricular function, high-risk patients requiring long aortic cross clamp times and those with occlusive coronary disease may benefit from a dual approach, that is, both antegrade and retrograde techniques. Another advantage to the retrograde technique is that it may be effective in reducing the risk of embolization from saphenous vein grafts that could occur during antegrade perfusion for reoperative coronary artery bypass surgery. The retrograde approach also has the theoretical advantage of ensuring a more homogeneous distribution of the cardioplegic solution to regions of the heart that are poorly collateralized.
Despite these advantages, retrograde cardioplegia is not without its limitations. There are experimental and clinical studies which suggest that cardioplegia administered via the coronary sinus results in a suboptimal distribution of cardioplegia to the right ventricle. This is due in part to the anterior region of the right ventricle being poorly drained by the coronary sinus, the marked variability in venous anatomy, the frequency of coronary sinus anomalies and the Thebesian veins draining directly into the cavities of the heart. As a consequence a number of studies have been performed to evaluate not only the safety and efficacy of the retrograde approach but also to compare its efficacy to a combined antegrade/retrograde approach.114-117 While the findings have been mixed, most of results have shown that retrograde delivery of cardioplegic solutions is safe and effective during cardiac surgery. Taking a different approach, Bhaya et al compared the efficacy of an antegrade approach with an integrated antegrade/retrograde method in patients undergoing on pump heart surgery using three-dimensional transthoracic speckle tracking echocardiography.118 Twenty-two patients were studied one day prior to surgery and 4 to 5 days after the operation. They found that the integrated approach conferred better protection in terms of maintenance of strain parameters in segments of the septum and free wall of the left ventricular free wall and septum. These findings support the general consensus that a combined approach allows for a more reliable homogeneous distribution of cardioplegic solutions. The combined approach may be most relevant when it is anticipated that the cross-clamp time will be long and/or when the coronary artery stenoses are particularly extensive and severe.
To minimize the interruption of coronary flow during aortic cross-clamping, cardioplegia can be administered continuously using a retrograde coronary sinus catheter or cannulating the coronary ostia directly via an open aortic root. A continuous infusion, especially if it is oxygenated, has the theoretical advantage of minimizing ischemia particularly during periods of prolonged aortic cross-clamping. One of the first studies to test this assumption was by Louagie and colleagues.119 Seventy patients undergoing CABG surgery were prospectively randomized to receive retrograde cold blood cardioplegia either intermittently (n = 35) or in a continuous fashion (n = 35). Hemodynamic measurements included right ventricular ejection fraction, cardiac output, left and right ventricular stroke work index and systemic and pulmonary vascular resistance. Coronary sinus blood samples were obtained before aortic cross-clamping and immediately after unclamping for the measurement of lactate and hypoxanthine levels; venous blood samples were collected at predetermined times to measure CK-MB and troponin levels. Overall, they found a trend for better hemodynamics and a lower use of inotropic agents and significantly lower lactate and hypoxanthine levels in the patients who received continuous retrograde cardioplegia. On the other hand there were no differences in patient outcomes, and the CK-MB and troponin levels were comparable postoperatively. Given this was a single-center study, the surgery was performed by the same surgeon, and the relatively small sample size, it is difficult to extrapolate the findings to the general population of patients undergoing cardioplegic surgery with aortic cross-clamping. To date it remains unclear as to whether continuously administered cardioplegia is superior to intermittent cardioplegia. The major advantage of using intermittent cardioplegia is the ability to achieve and sustain a dry, quiescent operative field. Moreover, continuous cardioplegia still necessitates interruption of the surgical steps while the infusion is delivered.
In summary, controversy persists as to whether blood cardioplegia or crystalloid cardioplegia is better, which temperature is ideal and what is the best method of delivery. A survey of practice patterns in the United Kingdom in 2004 showed that 56% of all cardioplegia on-pump surgery was performed with cold blood cardioplegia; whereas, warm blood cardioplegia was used in 14% of cases.120 In the same survey, 14% of surgeons used crystalloid cardioplegia, 21% used retrograde cardioplegia and 16% did not use any cardioplegia, preferring to use cross-clamp fibrillation only. Based on these observations and experiences in the United States, it appears that most surgeons favor cold intermittent blood cardioplegia. Nevertheless a wide spectrum of variation exists among heart centers; there is no international consensus regarding the ideal cardioplegic solution or its use.120 Awareness of the options allows the surgeon to utilize the best approach that meets the individual needs of the patient.
Intermittent cross-clamping with fibrillation (ICCF) and systemic hypothermia with intermittent elective fibrillatory arrest are the most common forms of noncardioplegic heart surgery used today. The objective is to provide a relatively quiescent surgical field without having to arrest the heart with a cardioplegic solution.
This technique is the earliest method developed to protect the heart during surgery and is still used at many centers. The patient is placed on CPB, the ascending aorta is cannulated and generally a dual-stage single-venous cannula is used. Often the patient is cooled to 30 to 32°C; this technique allows the surgeon to operate in a relatively quiet but not quiescent field during ventricular fibrillation. In the setting of CABG surgery, the aortic cross-clamp is removed intermittently after the completion of each graft. The duration of fibrillation is determined by how long it takes to perform the distal anastomosis. After completion of the revascularization, the heart is defibrillated and the proximal anastomoses performed on the beating heart using an aortic partial occlusion clamp. This technique is particularly useful in patients with cold agglutinin disease, an autoimmune phenomenon in which antibody directly agglutinates human red cells at low temperatures. In these patients open heart operations with hypothermia carry the risk of red cell agglutination and may result in hemolysis, MI, renal insufficiency and cerebral damage.
As a result of increasing pressures to reduce cost and yet maintain acceptable levels of cardioprotection, there remains an interest in using this approach. There are a number of reports which indicate that satisfactory protection can be conferred using this technique. In 1992, Bonchek et al reported a large clinical series in which the advantages and safety of this technique were meticulously analyzed.121 In this study, the authors reviewed the outcomes of the first 3000 patients at their institution who underwent primary CABG using ICCF. Preoperative risk factors, for example, age, gender, left ventricular dysfunction, preoperative use of the intra-aortic balloon pump (IABP), the urgency of operation and operative deaths were analyzed. In this series, 29% of the patients were older than 70 years of age, 27% were females, 9.7% had an ejection fraction of less than 0.30, 13% had an MI less than 1 week preoperatively and 31% had preinfarction angina in the hospital; only 26% underwent purely elective operations. Using the noncardioplegic cardioprotective technique, the authors reported an elective operative mortality rate of 0.5%, an urgent mortality rate of 1.7% and an emergency rate of 2.3%. Postoperatively, inotropic support was needed in only 6.6% of the patients, and only 1% required insertion of the IABP. It is important to note however that this was a retrospective, single-center institutional experience. The findings would have been enhanced if the analysis had included a similarly matched group of patients at the same institution in which cardioplegic arrest had been employed. Nevertheless the findings support the perspective that noncardioplegic strategies can provide satisfactory myocardial protection even in high-risk patients.
In 2002, Raco and colleagues reported their experience in 800 consecutive CABG operations performed by a single surgeon using ICCF in both elective and nonelective settings. The patients were divided into three cohorts: (1) elective; (2) urgent; and, (3) emergent. Among these cohorts the mean age, number of distal grafts and mortality among the three groups was comparable. The mortality rate in the elective, urgent and emergent groups was 0.6%, 3.1%, and 5.6%, respectively, and consistent with outcomes associated with cardioplegic surgery. Since this report reflects the experience of a single surgeon, there is the concern that the technique may not be generally applicable. Regardless, the findings do support the notion that ICCF is a safe technique for both elective and nonelective patients undergoing CABG surgery.122-124 In 2003, Bonchek et al reported their experience in 8300 patients who underwent CABG surgery without cardioplegia. The unadjusted mortality rates in elective, urgent and emergent patients was 0.9%, 1.5%, and 4.0%, respectively. The overall mortality of 1.7%, was considerably lower than the 3.27% predicted based on the Society of Thoracic Surgeons National Database model.125 This experience represented the work of five surgeons, three of whom had not been trained in noncardioplegic surgery; it provides additional evidence that ICCF is an effective form of cardioprotection. Evidence that the cardioprotective effects of ischemic preconditioning (IPC) may contribute to the efficacy of ICCF in the human heart has resulted in an interest in using this method of protection especially in the United Kingdom. Results in animal studies indicate that the protective effect of ICCF is blocked by protein-kinase C inhibitors and KATP channel antagonists, both of which have been implicated in the signaling pathways involved in IPC.126 Regardless of the mechanism underlying the protection, there are numerous reports which indicate that noncardioplegic strategies such as ICCF can provide satisfactory myocardial protection even in high-risk patients.127
Elective fibrillatory arrest is another safe approach to protect the heart during noncardioplegic heart surgery. The use of systemic hypothermia (26-30°C) and maintenance of systemic perfusion pressure between 80 and 100 mm Hg are key elements. This approach is particularly applicable in the setting of the severely calcified “porcelain aorta,” where clamping the aorta may be associated with increased risk of stroke and aortic dissection. Under these circumstances the distal anastomoses are performed locally occluding the coronary artery using vascular clamps or sutures. The proximal anastomoses can be completed during short periods of hypothermic circulatory arrest. Alternatively the proximal anastomoses can be based entirely on an in situ internal thoracic artery. Using this approach one can avoid manipulation of the aorta altogether. Akins and colleagues, in 1984, reported a low incidence of perioperative MI and a low hospital mortality rate in 500 consecutive patients using this technique.84 In 1987, Akins and Carroll assessed the late results of hypothermic fibrillatory arrest in 1000 consecutive patients undergoing nonemergent CABG surgery. They concluded that the technique is effective and yields excellent event-free survival. Potential disadvantages include (1) the surgical field may be obscured as a result of existing collateral circulation; (2) ventricular fibrillation may be associated with increased muscular tone and thus compromise the surgeon’s ability to position the heart for optimal exposure; (3) aortic valvular regurgitation may be exacerbated; and (4) it is generally not applicable for intracardiac procedures. An advantage with the technique is that it can be used when aortic occlusion or cardioplegic arrest is undesirable, for example, in the setting of a calcified ascending aorta. This approach can also be used in patients undergoing mitral valve surgery.128 Imanaka et al, in 2003, published a retrospective observational study in which mitral valve surgery was performed in 27 patients with ischemic mitral regurgitation using perfused ventricular fibrillation. Concomitant procedures included CABG in 23 patients and the Dor procedure in five patients. Surgery was performed using moderate hypothermia (~28°C) and fibrillatory arrest; flow rates on bypass were maintained at 2.4 L/min/m2 and the perfusion pressure was 70 mm Hg. Among these select patients the mortality rate was 3.7%. The authors concluded that extended periods of ventricular fibrillation during hypothermic surgery can be well-tolerated without excessive morbidity and mortality and is appropriate in patients in whom aortic cross-clamping is unsuitable or the duration of cross-clamping is expected to be long.129
There are currently a number of physiological processes and pharmacological agents known to confer protection against I/R injury in the experimental setting. Several clinical trials are underway to determine the relevance of these new approaches. The purpose of this section is to review the cardioprotective strategies that are being examined and hold promise for the future.
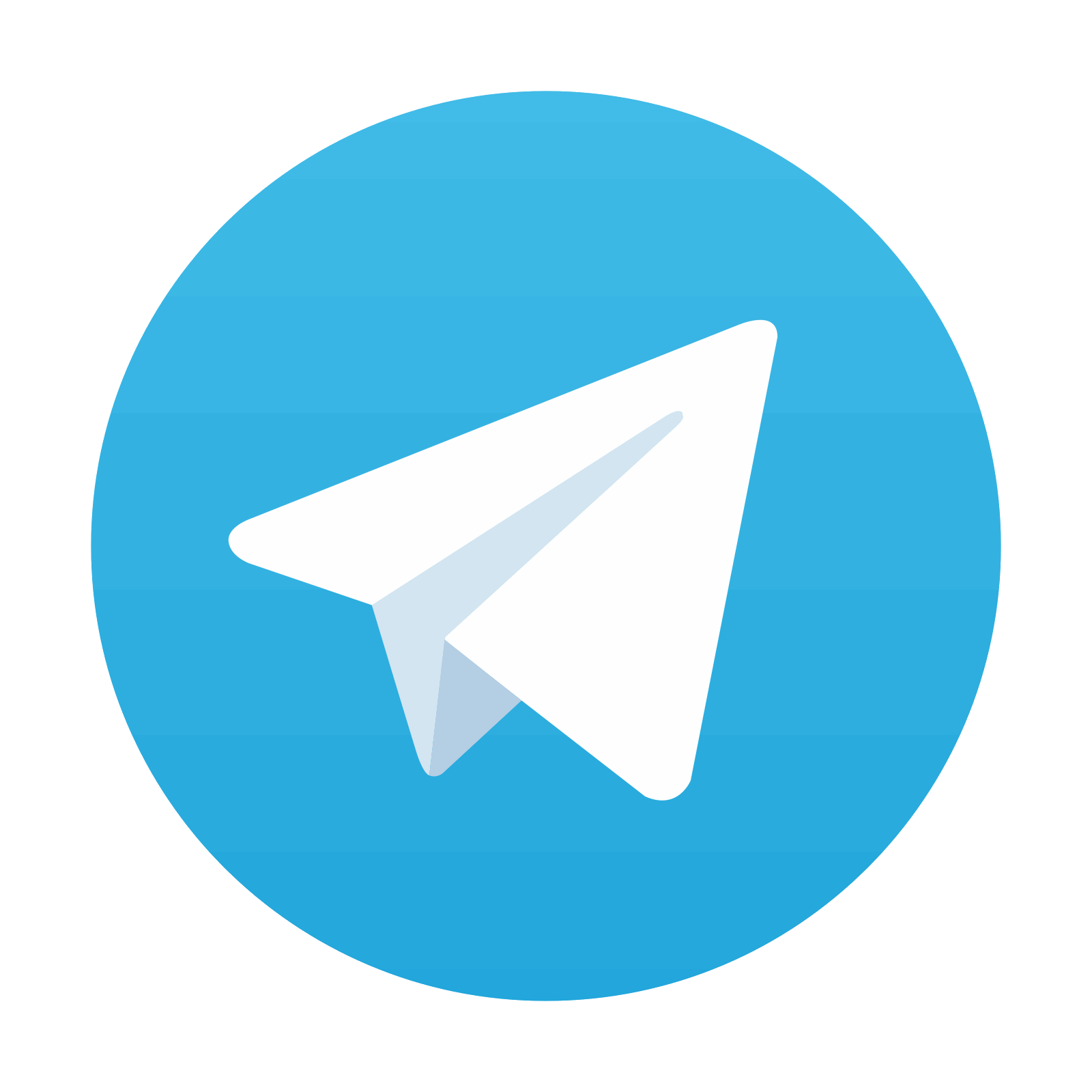
Stay updated, free articles. Join our Telegram channel

Full access? Get Clinical Tree
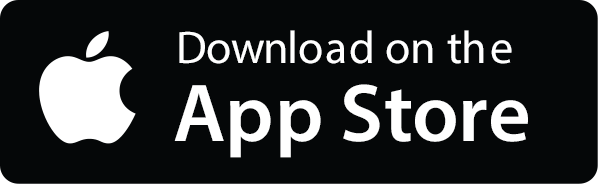
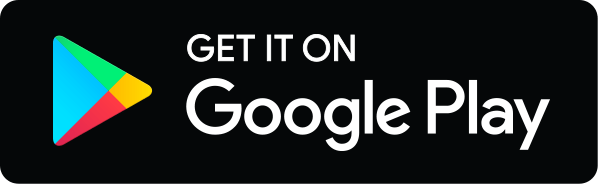