Background
The aim of this study was to determine whether two-dimensional speckle-tracking echocardiography can identify the myocardial damage detected by delayed enhancement (DE) magnetic resonance imaging via the differences in myocardial deformation in patients with extracardiac sarcoidosis who showed no structural and functional abnormalities in the heart.
Methods
Forty-five patients with biopsy-proven extracardiac sarcoidosis were analyzed retrospectively. Patients with abnormal electrocardiographic and echocardiographic findings, including ventricular arrhythmias, heart block, regional wall motion abnormalities, valvular heart disease, and cardiomyopathy, were excluded. Ten age-matched healthy control subjects were recruited as a control group. Comprehensive echocardiography and DE magnetic resonance imaging were performed, and circumferential, longitudinal, and radial strain were consecutively assessed using two-dimensional speckle-tracking echocardiographic software in a 16-segment model of the left ventricle in accordance to the presence (DE+) or absence (DE−) of DE.
Results
Among the 45 patients, 36 segments in 13 patients showed DE. DE+ segments had lower peak circumferential strain than DE− and control segments (−14 ± 5% vs −28 ± 7% vs −30 ± 7%, P < .0001). Peak longitudinal strain in DE+ segments was significantly decreased compared with control segments (−19 ± 4% vs −23 ± 5%, P = .005). However, peak radial strain was similar among the three groups: 41 ± 17% in DE+ segments, 45 ± 23% in DE− segments, and 46 ± 18% in control segments ( P = .50).
Conclusions
Circumferential and longitudinal strain via two-dimensional speckle-tracking echocardiography can identify the myocardial damage detected by DE magnetic resonance imaging in patients with extracardiac sarcoidosis.
Sarcoidosis is a multisystem granulomatous disease of unknown etiology. It is not commonly fatal, but cardiac involvement is responsible for more than two thirds of the deaths. Early diagnosis and initiation of corticosteroid therapy in patients with cardiac sarcoidosis (CS) was proved to improve left ventricular (LV) function and prevents malignant arrhythmia. However, early diagnosis by echocardiography, electrocardiography, and radionuclide imaging, which are commonly used to assess cardiac involvement, is still challenging.
Delayed enhancement (DE) cardiac magnetic resonance imaging (MRI) has evolved into the noninvasive reference technique for the analysis of myocardial fibrosis in patients with CS. DE MRI has been shown to have high accuracy in the identification of myocardial damage in the early stage of CS. Unfortunately, the test is not always available, because it cannot be effectively used in patients with claustrophobia, non-MRI-compatible pacemakers and implantable cardioverter-defibrillators, and renal dysfunction.
Recently, two-dimensional (2D) speckle-tracking echocardiography (STE) has been introduced as a new echocardiographic technique to assess regional and global LV strain. Speckle-tracking strain is measured independently of angle and measures regional deformation in the longitudinal, circumferential, and radial directions of the left ventricle. Several studies have found that speckle-tracking strain is associated with the amount and location of LV fibrosis assessed by DE MRI in patients with cardiomyopathy. We therefore hypothesized that 2D STE could identify the myocardial damage detected by DE MRI in patients with extracardiac sarcoidosis who show no obvious echocardiographic and electrocardiographic abnormalities.
Methods
Patients
Forty-eight patients who presented with biopsy-proven extracardiac sarcoidosis were retrospectively screened between May 2012 and December 2013 at Wakayama Medical University Hospital. Exclusion criteria were clinical findings of cardiac involvement, including LV dysfunction (LV ejection fraction [LVEF] < 50%) and abnormal electrocardiographic (atrioventricular block, ventricular arrhythmias, complete right and left bundle branch block, axis deviation, and abnormal Q wave) and echocardiographic (wall thinning, regional abnormal wall motion, and ventricular aneurysm) findings according to Japanese Ministry of Health and Welfare criteria. Other exclusion criteria included atrial fibrillation. Among the 48 patients, one was excluded for an LVEF < 50%, one for right bundle branch block, and one for atrioventricular nodal conduction disease. No patients were excluded because of contraindications to MRI (severe renal impairment, implantable cardioverter-defibrillator, or pacemaker). Therefore, the final study population consisted of 45 patients. Ten age-matched, completely healthy control subjects were recruited as a control group, with no evidence of sarcoidosis, coronary artery disease, or valvular disease; these subjects did not have noncardiac comorbidities such as hepatic, renal, and malignant diseases. All subjects underwent conventional and color Doppler echocardiography and DE MRI on the same day.
The study was approved by the regional ethics committee, and all patients gave written informed consent.
Echocardiography
Echocardiograms were performed with a Vivid E9 system (GE Vingmed Ultrasound AS, Horten, Norway) equipped with a 2.5-MHz transducer. Parasternal long- and short-axis views at the basal, midventricular, and apical levels as well as three standard apical views (four-chamber, two-chamber, and long-axis) were acquired from three consecutive beats (frame rate, 56–92 frames/sec). Septal and posterior wall thicknesses were measured at end-diastole and the level of the LV minor axis, approximately at the mitral valve leaflet tips, from 2D recordings. LVEF was determined by manual tracing of end-systolic and end-diastolic endocardial borders using apical four- and two-chamber views, using the biplane Simpson method from the average of three beats. The original 16-segment model of the American Society of Echocardiography was used to divide the left ventricle. Left atrial volume was determined using the modified Simpson method. LV mass was estimated using M-mode echocardiography.
Electrocardiography
A 12-lead surface electrocardiogram was recorded. The findings were interpreted by an experienced cardiologist and classified as abnormal when right and left bundle branch block, axis deviation, atrioventricular nodal conduction disease, ventricular tachycardia, premature ventricular contraction, pathologic Q wave, or ST-T abnormality was noted.
Measurement of Strain by 2D Speckle-Tracking
The three acquired parasternal short-axis views and apical levels were analyzed considering the 16-segment model (six segments for the basal and midventricular short-axis view and four segments for the apical short-axis view). The recordings were stored digitally for offline analysis with a dedicated image processing and analysis program (EchoPAC; GE Vingmed Ultrasound AS). Radial and circumferential strain was assessed by 2D STE in three short-axis views (basal, midventricular, and apical), and segmental longitudinal strain was assessed by 2D STE in apical four-chamber, two-chamber, and long-axis projections. The endocardial borders were manually traced in end-systole and adjusted if automatic tracking was considered suboptimal by visual or automated assessment. Segmental peak strain was automatically calculated as the average strain within each segment. Peak strain was defined as the maximum positive or negative strain value during systole or early diastole. End-systole was defined as aortic valve closure in the apical long-axis view. Each strain parameter was acquired from the average of three beats. In segments with poor tracking, the observer readjusted the endocardial trace line until a better tracking score was achieved. If even one strain parameter was unanalyzable in the tracking segment, the segment was excluded. Global radial, circumferential, and longitudinal strain for the left ventricle were also calculated as the mean value of 16 segments in all patients. All echocardiographic measurements and peak strain data were obtained by two independent observers without knowledge of the clinical status and findings of the other imaging modalities of the patient.
Delayed Enhancement MRI
All patients underwent DE MRI on the same day of the echocardiographic study. All DE MRI procedures were performed with a 1.5-T clinical scanner (Intera Achieva; Philips Medical Systems, Best, The Netherlands) equipped with a five-element cardiac phased-array coil for signal reception, as previously described. First, breath-hold cine steady-state free precession images with a time resolution of 35 msec were acquired. Next, myocardial edema was assessed using breath-hold, black-blood, T2-weighted, short–inversion time, inversion-recovery, fast spin-echo imaging in the cardiac short-axis orientation. Finally, a breath-hold three-dimensional turbo gradient-echo sequence with inversion recovery was used to obtain the late enhanced images. Contiguous short-axis slices and representative long-axis slices of the left ventricle were obtained 10 min after intravenous injection of 0.1 mmol/kg gadolinium diethylenetriamine penta-acetic acid (Magnevist; Schering AG, Berlin, Germany). Scan parameters were as follows: repetition time, 4.1 msec; echo time, 1.25 msec; flip angle, 15°; field of view, 350 × 350 mm; partial echo; matrix size, 224 × 256; and spatial resolution, 1.56 × 2.24 × 10 mm 3 reconstructed to 0.68 × 0.68 × 5 mm 3 . All images were acquired during breath-hold at end-expiration. The inversion time was optimized (200–300 msec) to null the normal myocardium. There were no complications related to the MRI procedures, and all patients tolerated the procedure well.
Images were subsequently transferred to a workstation equipped with a dedicated cardiac software package (View Forum; Philips Medical Systems) for further analysis as described previously. Each myocardial segment was evaluated for the presence of DE, defined as an area of signal enhancement ≥5 SDs of the signal intensity of nonenhanced myocardium. The presence or absence of DE was determined by consensus of two experienced readers for each of the 16-segments of the left ventricle, blinded to clinical data and the results of the other imaging technique. We also classified the subsegmental layers with DE in each segments as subepicardial, midwall, subendocardial, and transmural. The subepicardial layer included the subepicardial side of the LV free wall and right ventricular side of the ventricular septum. We defined transmural lesions as those characterized by continuous DE from the endocardium to the epicardium.
Reproducibility
To define intraobserver agreement of three strain parameters, strain analysis, was reperformed 1 week apart by the same observer on the same cardiac cycle in 10 randomly selected subjects with 160 segments. We also analyzed interobserver agreement by a second independent observer who was blinded to previous measurements. Strain analysis was reperformed on the same cardiac cycle in the same 10 subjects. Bland-Altman analysis was conducted to assess intraobserver and interobserver agreements (expressed as the absolute value of the mean difference ± 2 SDs), and intraclass correlation coefficients were calculated.
Statistical Analysis
Data are expressed as mean ± SD. Categorical data are expressed as frequencies and percentages. Categorical variables were compared using χ 2 tests. Comparison between segments with or without DE and control subjects about each strain parameters were performed with analysis of variance.
The output of this analysis allowed the derivation of receiver operating characteristic (ROC) curves, which were used to designate cutoffs and calculate the area under the curve (AUC). Sensitivities and specificities for all strain values were determined for the ability to identify DE. The AUC for three strain parameters were compared by using the method of Hanley and McNeil. The correspondence between AUC and the Wilcoxon statistic was used, and underlying Gaussian distributions (binormal) were assumed to provide a table that converted the observed correlations in paired ratings of images into a correlation between the two ROC areas. This between-area correlation could be used to reduce the standard error (uncertainty) about the observed difference in areas. This correction for pairing, analogous to that used in the paired t test, can produce a considerable increase in the statistical sensitivity (power) of the comparison. All tests were two sided and assessed at the 5% significance level. All statistical analyses were performed using SPSS version 11.0.1 (SPSS, Inc, Chicago, IL).
Results
Study Group
Clinical baseline characteristics of all patients are given in Table 1 . There were 13 patients in whom MRI studies were positive for DE, while in the other 32 patients, MRI studies were negative for DE. Myocardial edema was not detected in any patients. We separated the patients into positive (DE+) and negative (DE−) for DE. There was no difference in age, gender, years since diagnosis, steroid use, angiotensin-converting enzyme use, B-type natriuretic peptide level, serum and urinary calcium levels, and high-sensitivity C-reactive protein level between the two groups. A list of the medications is also shown in Table 1 .
Variable | DE+ ( n = 13) | DE− ( n = 32) | P |
---|---|---|---|
Age (y) | 64 ± 9 | 57 ± 13 | .07 |
Women | 8 (62%) | 20 (63%) | .61 |
Years since diagnosis | 5 ± 5 | 5 ± 3 | .83 |
Coronary risk factors | |||
Hypertension | 3 (23%) | 5 (16%) | .42 |
Diabetes mellitus | 2 (15%) | 1 (3%) | .20 |
Dyslipidemia | 3 (23%) | 5 (16%) | .42 |
Lung involvement | 9 (69%) | 23 (72%) | .56 |
ACE (U/L) | 20.0 ± 8.5 | 20.9 ± 7.4 | .71 |
BNP (pg/mL) | 26 ± 21 | 30 ± 13 | .82 |
Serum calcium (mEq/L) | 9.8 ± 0.5 | 9.4 ± 1.0 | .21 |
Urinary calcium (mEq/L) | 14.1 ± 8.1 | 12.4 ± 9.8 | .63 |
High-sensitivity CRP (mg/dL) | 0.29 ± 0.32 | 0.13 ± 0.15 | .14 |
Medications | |||
Steroids | 1 (7%) | 5 (16%) | .43 |
ACE inhibitors or ARBs | 2 (15%) | 3 (9%) | .45 |
β-receptor blocker | 1 (7%) | 0 (0%) | .29 |
Statin | 3 (23%) | 5 (16%) | .42 |
During follow-up (mean duration, 21 ± 7 months), no patients had major adverse events, including heart failure, atrioventricular block, ventricular arrhythmias, and death.
Echocardiographic and Electrocardiographic Findings
As demonstrated in Table 2 , septal and posterior wall thickness, LV end-diastolic and end-systolic volumes, LV mass, and left atrial volume were similar in the DE+, DE−, and control groups (three men; mean age, 57 ± 13 years; range, 37–78 years). LVEF was also similar in the three groups: 62 ± 4.6% in the DE+ group, 61 ± 4.0% in the DE− group, and 61 ± 2.7% in the control group ( P = .91). There were no significant differences in heart rate, PR interval, QRS duration, and corrected QT intervals among the three groups ( Table 2 ).
Variable | DE+ ( n = 13) | DE− ( n = 32) | Control ( n = 10) | P |
---|---|---|---|---|
Electrocardiography | ||||
Heart rate (beats/min) | 74 ± 11 | 68 ± 13 | 69 ± 8 | .17 |
PR interval (msec) | 183 ± 50 | 164 ± 30 | 169 ± 14 | .19 |
QRS duration (msec) | 95 ± 19 | 91 ± 13 | 91 ± 8 | .96 |
QT interval (msec) | 383 ± 23 | 399 ± 33 | 388 ± 16 | .35 |
Corrected QT interval (msec) | 407 ± 18 | 411 ± 16 | 410 ± 13 | .78 |
Echocardiography | ||||
Septal wall thickness (cm) | 0.9 ± 0.2 | 0.8 ± 0.1 | 0.8 ± 0.1 | .80 |
Posterior wall thickness (cm) | 0.9 ± 0.1 | 0.8 ± 0.1 | 0.8 ± 0.1 | .54 |
LVEDV (mL) | 84 ± 17 | 89 ± 24 | 86 ± 16 | .86 |
LVESV (mL) | 32 ± 9 | 35 ± 12 | 34 ± 9 | .82 |
LVEF (%) | 62 ± 4.6 | 61 ± 4.0 | 61 ± 2.7 | .91 |
LV mass index (g/m 2 ) | 78 ± 21 | 74 ± 20 | 77 ± 20 | .80 |
LA volume maximum (mL) | 52 ± 19 | 49 ± 15 | 56 ± 16 | .16 |
Feasibility of Echocardiographic and Strain Measurements
In 677 of 720 LV segments (94%), echocardiographic image quality allowed analysis of segmental function and strain imaging. The feasibility of radial, circumferential, and longitudinal strain assessment was 88%, 94%, and 95%, respectively. Distribution of the missed segments showed a high incidence of the basal anterolateral (42%) and apicolateral (39%) segments.
Association with DE Segments Using 2D Speckle-Tracking Echocardiographic Analysis
Of 677 segments in patients with extracardiac sarcoidosis, 36 segments showed DE. Figure 1 shows the distribution of DE lesions among the myocardial segments. Nine of the 36 segments (25%) were distributed in the ventricular septum, and 18 of the 36 segments (50%) were distributed in the inferior-inferolateral segments. DE on the right ventricular side of the ventricular septum was observed in four of 13 patients (31%). Thirteen segments in seven patients (36%) showed in subepicardial lesions, 21 segments in five patients (58%) showed in midwall lesions, two segments in two patients (6%) showed in subendocardial lesions, and transmural lesions were not observed.
Peak radial, circumferential, and longitudinal strain data for DE+, DE−, and control segments are given in Table 3 . DE+ segments had lower peak circumferential strain (−14 ± 5%) than DE− segments (−28 ± 7%) and control segments (−30 ± 7%) ( P < .0001). Peak longitudinal strain in segments with DE+ (−19 ± 4%) was significantly decreased compared with the control segments (−23 ± 5%) ( P = .005). However, peak radial strain was similar among the three groups: 41 ± 17% in DE+ segments, 45 ± 23% in DE− segments, and 46 ± 18% in control subjects ( P = .50) ( Table 3 and Figure 2 ).
Variable | DE+ ( n = 36) | DE− ( n = 641) | Control ( n = 157) | P |
---|---|---|---|---|
Myocardial deformation imaging (%) | ||||
Radial strain | 41 ± 17 | 45 ± 23 | 46 ± 18 | .50 |
Circumferential strain | −14 ± 5 | −28 ± 7 | −30 ± 7 | <.0001 |
Longitudinal strain | −19 ± 4 | −22 ± 5 | −23 ± 5 | .005 |
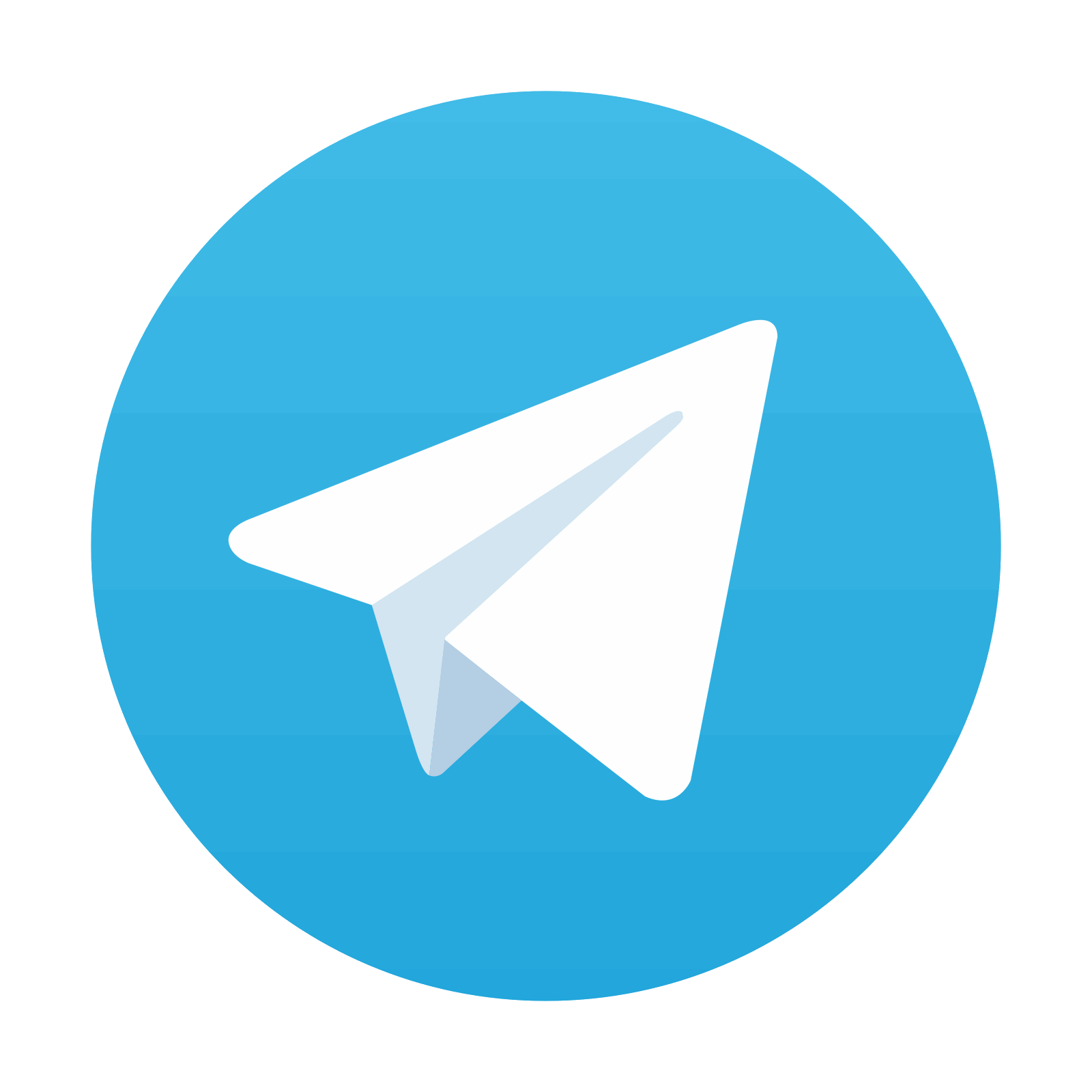
Stay updated, free articles. Join our Telegram channel

Full access? Get Clinical Tree
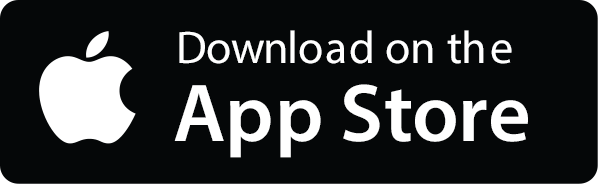
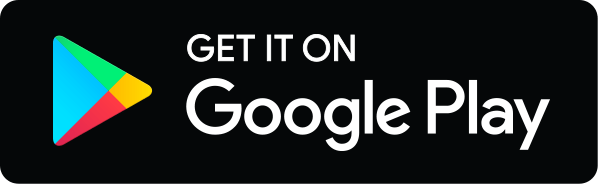
