Background
Using speckle-tracking imaging (STI), the aims of this study were to assess dyssynchrony and quantify the myocardial energy wasted by contractility in delayed segments by determining the longitudinal strain delay index (LSDi) in patients with heart failure with preserved ejection fraction (HFpEF).
Method
Thirty-eight patients with HFpEF and 33 matched controls were recruited. All subjects underwent clinical examinations, 12-lead electrocardiography, pulmonary function tests, echocardiography, and metabolic exercise tests. LSDi was determined, the magnitude of which is a measure of the amount of wasted energy. Global and segmental systolic and diastolic dyssynchrony was assessed by STI.
Results
LSDi was significantly higher in patients with HFpEF than controls (−14.36 ± 8.24% vs −10.73 ± 5.62%, P < .05). Patients with HFpEF exhibited left ventricular (LV) systolic and diastolic dyssynchrony, with the LV anterior wall displaying the most delayed motion.
Conclusion
Patients with HFpEF exhibited increased myocardial contractile inefficiency. They also exhibited LV systolic and diastolic dyssynchrony, with the LV anterior wall displaying the most delayed motion.
Many consider heart failure with preserved ejection fraction (HFpEF) to be a disorder of diastolic function, whereas others believe that it may be due to a combination of diastolic abnormalities with subtle disturbances of systolic function that are insufficient to reduce left ventricular (LV) ejection fraction (LVEF). Studies using Doppler tissue imaging (DTI) have demonstrated the presence of diastolic and/or systolic dyssynchrony in patients with HFpEF. Recently, a novel technique known as speckle-tracking imaging (STI) has been used to quantitatively evaluate myocardial strain patterns independent of cardiac translation and insonation angle, which are major limitations of DTI-derived myocardial strain and velocities. STI is also less time-consuming than DTI and has been validated in experimental and human studies. In addition, STI allows for assessments of segmental synchronicity and also the longitudinal strain delay index (LSDi).
LSDi is derived from longitudinal strain (ϵ) patterns and is a strong predictor of responders to cardiac resynchronization therapy (CRT) in both ischemic and nonischemic patients. LSDi quantifies the wasted energy in dyssynchronous ventricles, which is brought about because delayed segments do not contribute fully to end-systolic (ES) function. Furthermore, the wasted energy is greater in delayed segments with preserved contractility compared with delayed segments with reduced contractility (ie, scar or fibrotic myocardium).
In this study, using STI, we aimed to assess LV global and segmental synchronicity and to determine which LV segments display delayed motion, therefore enabling the identification of potential cardiac pacing sites for the correction of LV dyssynchrony. In addition, we aimed to quantify the wasted energy due to systolic dyssynchrony by determining LSDi.
Methods
Study Participants
Patients With HFpEF
We studied 38 patients with HFpEF, who were prospectively and consecutively recruited from heart failure clinics. All study participants underwent clinical examinations, 12-lead electrocardiography, pulmonary function tests, echocardiography, and metabolic exercise tests. All patients had signs and/or symptoms of heart failure, with LVEFs > 50% by transthoracic echocardiography, and met the criteria of Yturralde and Gaasch for diastolic heart failure. Patients with severe pulmonary disease, significant valvular heart disease, atrial fibrillation, or evidence of hypertrophic cardiomyopathy were excluded. Patients with rhythms other than sinus and those with QRS durations > 120 ms were excluded. The study was performed at the University of Birmingham with approval of the research ethics committee. Informed consent was obtained from all patients and controls.
Healthy Controls
We studied 33 healthy controls with no histories of cardiac disease, hypertension, or diabetes mellitus. Healthy volunteers from the community who responded to our advertisements were recruited on the basis that they were of similar age and gender as our patient group and had normal results on clinical cardiovascular examinations, 12-lead electrocardiography, echocardiography, and metabolic exercise testing.
Metabolic Exercise Testing
The metabolic exercise testing was performed using a Schiller CS-200 Ergo-Spiro exercise machine (Schiller, Irvine, CA), which was calibrated before every study. Subjects underwent spirometry, followed by symptom-limited erect treadmill exercise testing using an incremental ramp protocol (speed and inclination were increased every minute) with simultaneous respiratory gas analysis. Samplings of expired gases were performed continuously, and data were expressed as 30-second means. Minute ventilation, oxygen consumption, carbon dioxide production, and the respiratory exchange ratio were obtained. Peak oxygen consumption (Vo 2 max) was defined as the highest value of oxygen consumption measured during the exercise period. Blood pressure and electrocardiograms were monitored throughout. Subjects were encouraged to exercise to exhaustion, with a minimal requirement of a respiratory exchange ratio ≥ 1.
Resting Echocardiography
Echocardiography was performed with participants in the left lateral decubitus position using a Vivid 7 (GE Medical Systems, Milwaukee, WI) and a 2.5-MHz transducer. Resting scans were acquired in standard apical 4-chamber and apical 2-chamber views. All echocardiographic measurements were averaged from 3 heartbeats. LVEF and LV hypertrophy were determined in accordance with the guidelines. From the LV inflow pattern (measured at the tips of the mitral valve), peak early (E) and late (A) filling velocities, E/A ratio, and E-velocity deceleration time were measured. The isovolumic relaxation time was determined using pulsed-wave Doppler velocity data of LV inflow. Tissue Doppler was applied at end-expiration in the pulsed-wave Doppler mode at the level of the lateral mitral annulus from an apical 4-chamber view. The velocities of the mitral annular systolic wave (S′), early diastolic wave (E′), and late diastolic wave (A′) were noted. Lateral mitral annular velocities were recorded to derive E/E′. Parasternal circular short-axis images were taken at the papillary level (identified by the papillary muscles present), similar to previous studies.
STI
STI was performed using a commercially available speckle-tracking system in an EchoPAC (version 4.2.0) workstation (GE Medical Systems). Myocardial deformation measurements were performed using tissue speckle tracking. In this speckle-tracking system, the displacement of speckles of myocardium in each spot was analyzed and tracked from frame to frame. We selected the best-quality digital 2-dimensional image cardiac cycle, and the LV endocardium was traced at end-systole. The region of interest width was adjusted as required to fit the wall thickness. The software package then automatically tracked the motion through the rest of the cardiac cycle. The onset of the QRS complex was taken as the beginning of systole. Adequate tracking was verified in real time. A high frame rate of 70 to 100 Hz was used, as in previous studies. For each subject, longitudinal strain values for all 12 LV myocardial segments in each of the apical 4-chamber and 2-chamber views were measured to derive LV longitudinal strain, strain rates, and velocity curves. In addition, radial and circumferential strain values for all 6 LV myocardial segments in the short-axis view at the papillary level were measured to derive radial and circumferential strain curves.
Strain Delay Index and Synchronicity Assessment
As described previously by Lim et al, in dyssynchronous ventricles, segments that are delayed do not contribute fully to global ES function. The energy wasted per segment because of dyssynchrony is expressed by the difference between peak strain (ϵ peak ) and ES strain (ϵ ES ). If all the LV segments were synchronous, the strain delay index would be zero, meaning that all the myocardial energy during the systolic period is efficiently used to push blood out of the left ventricle. However, as LV systolic dyssynchrony worsens, the strain delay index (ϵ peak − ϵ ES ) also increases. When the strain delay index is high, while some LV segments are contracting, others are still lagging behind, and thus, not all potential energy is efficiently used to move blood out of the left ventricle during systole. The strain delay index is a mathematical representation of the sum of wasted energy because of systolic dyssynchrony across LV myocardial segments:
Strain delay index = ∑ 1 n ( ϵ peak − ϵ ES )

Times to peak longitudinal strain (Ts-LS), circumferential strain (Ts-Circ), and radial strain (Ts-Rad) were also determined using STI. For the assessment of synchronicity, the standard deviations of Ts-LS, Ts-Circ, and Ts-Rad were computed to derive Ts-LS-SD, Ts-Circ-SD, and Ts-Rad-SD, respectively. Times to peak longitudinal velocity S (Ts), E (Te), and A (Ta) and peak longitudinal strain rate S (Ts-LSr), E (Te-LSr), and A (Ta-LSr) were also determined. For the assessment of synchronicity, the standard deviations of Ts, Te, and Ta were computed to derive Ts-SD, Te-SD and Ta-SD, respectively. The standard deviations of Ts-LSr, Te-LSr, and Ta-LSr were also determined to derive Ts-LSr-SD, Te-LSr-SD, and Ta-LSr-SD, respectively. For comparison, all time values were normalized for the R-R interval, which was calculated by dividing the time period by the R-R interval.
Statistical Analysis
Continuous variables are expressed as mean ± SD. Unpaired Student’s t tests (2 tailed) were used to assess group differences. Categorical variables were compared using χ 2 tests. A 2-tailed P value < .05 was considered statistically significant. Variances of data sets were determined using Levene’s test. Pearson’s correlation coefficient ( r ) was used to describe the relationship between two variables. All subjects were included in the model. SPSS version 15.0 (SPSS, Inc, Chicago, IL) was used to perform the statistical analyses.
Results
Characteristics of the Study Group
Patients with HFpEF were generally women (71%), overweight (body mass index > 25 kg/m 2 ), aged 67 ± 9 years, and hypertensive ( Table 1 ). Blood pressure was well treated (systolic blood pressure in patients vs controls, 137 ± 21 vs 134 ± 21 mm Hg; P = .49). The tissue Doppler E/E′ at the basal anterior-lateral segment (a measure of LV end-diastolic pressure) was significantly higher in patients than in controls (11 ± 4 vs 7 ± 2, P < .001). Patients with HFpEF also had significantly reduced V o 2 max compared with controls (21 ± 5 vs 34 ± 7 mL/kg/min, P < .001). The minute ventilation/carbon dioxide production relationship (VE/V co 2 slope) was also higher in patients with HFpEF than in controls (33 ± 6 vs 28 ± 3, P = .001).
Variable | Patients (n = 38) | Controls (n = 33) | P |
---|---|---|---|
Age (y) | 67 ± 9 | 65 ± 6 | .20 |
Women | 27 (71%) | 18 (55%) | .15 |
BMI (kg/m 2 ) | 30 ± 4 | 26 ± 4 | .003 |
Race (Caucasian) | 38 (100%) | 33 (100%) | |
CAD | 4 (11%) | 0 (0%) | |
Diabetes mellitus | 2 (5%) | 0 (0%) | |
Hypertension | 27 (71%) | 0 (0%) | |
LVH | 19 (50%) | 0 (0%) | |
Medications | |||
Loop diuretics | 12 (32%) | 0 (0%) | |
ACE inhibitors or ARBs | 24 (63%) | 0 (0%) | |
β-blockers | 7 (18%) | 0 (0%) | |
Nitrates | 3 (8%) | 0 (0%) | |
Calcium antagonists | 12 (32%) | 0 (0%) | |
Antiplatelet agents | 13 (34%) | 0 (0%) | |
Statins | 20 (59%) | 0 (0%) | |
Resting heart rate (beats/min) | 79 ± 15 | 79 ± 14 | .87 |
QRS duration(ms) | 90 ± 16 | 94 ± 14 | .25 |
Resting SBP (mm Hg) | 137 ± 21 | 134 ± 21 | .49 |
Resting DBP (mm Hg) | 82 ± 11 | 81 ± 11 | .67 |
V o 2 max (mL/kg/min) | 21 ± 5 | 34 ± 7 | <.001 |
RER | 1.07 ± 0.09 | 1.09 ± 0.09 | .31 |
VE/V co 2 | 33 ± 6 | 28 ± 3 | <.001 |
Breathing reserve (L/min) | 36 ± 15 | 40 ± 15 | .29 |
Peak SBP (mm Hg) | 181 ± 24 | 191 ± 24 | .12 |
Peak DBP (mm Hg) | 82 ± 13 | 88 ± 11 | .07 |
Peak heart rate (beats/min) | 136 ± 18 | 163 ± 9 | <.001 |
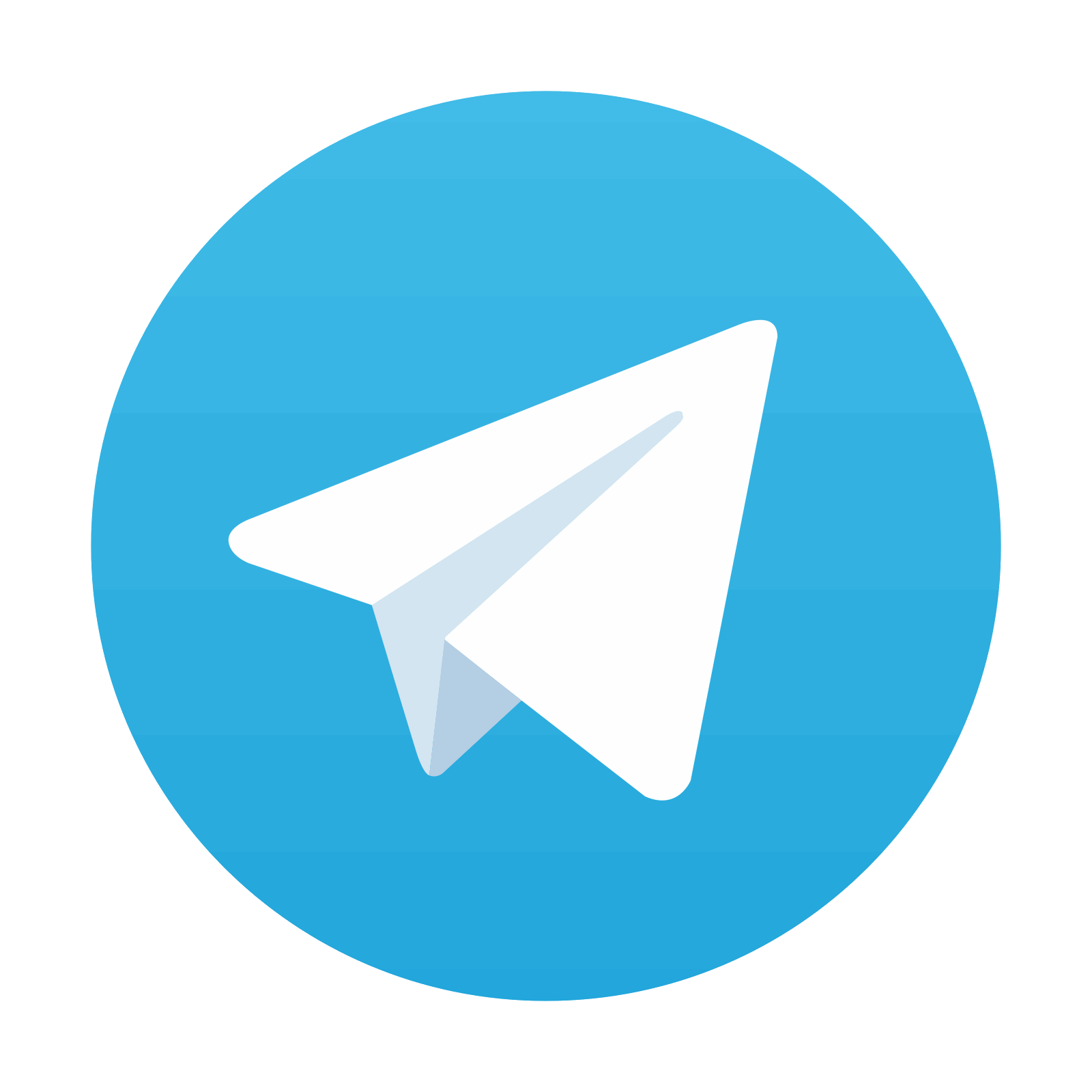
Stay updated, free articles. Join our Telegram channel

Full access? Get Clinical Tree
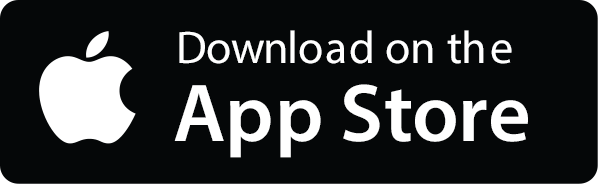
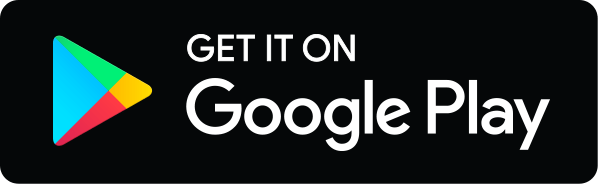
