Myocardial and Coronary Blood Flow and Metabolism
Mathew Liakos, MD
Kiran V. Reddy, MD, FACC
Allen Jeremias, MD, MSC
BASIC PRINCIPLE OF FFR
Epicardial Coronary Artery Versus Microcirculation
FIGURE 14.1 illustrates the importance of the coronary microcirculation in comparison with the epicardial coronary arteries, which represent only a small fraction (about 5%) of the total coronary vasculature. The left panel demonstrates a coronary angiogram of the left coronary system. The epicardial macrocirculation represents the conductance vessels that under normal conditions (in the absence of any critical coronary lesions) offer little resistance to blood flow. The right panel shows an ex vivo angiogram of the human heart submerged in saline to eliminate myocardial tissue shadowing and allowing visualization of the microvasculature that is not seen on typical coronary angiogram.1
Regulation of Coronary Blood Flow
The graph depicted in FIGURE 14.2 demonstrates coronary blood flow at rest and with maximal hyperemia.2 With maximal hyperemia, the coronary flow can normally increase 3- to 5-folds. As the luminal diameter of the vessel narrows to greater than 50%, the hyperemic flow reserve begins to diminish while resting flow is preserved. As the diameter stenosis further increases, both resting and hyperemic flows are affected, with coronary reserve flow diminishing in greater proportion to resting flow.
Derivation and Experimental Basis for FFR
The theoretical derivation of FFR was first described by Pijls et al in 19933 (FIGURE 14.3). While direct measurement of coronary blood flow may be ideal to determine the myocardial metabolism, in clinical practice, determination of coronary flow is technically difficult and thus impractical. The basic concept of coronary pressure-based measurements is to induce maximal hyperemia to eliminate the resistance of the microcirculation and thus “isolate” the epicardial vessel. Under those circumstances, based on the illustrated equations, coronary pressure equals coronary blood flow and allows the assessment of coronary stenoses on myocardial metabolism.
As the illustration demonstrates, aortic pressure (Pa) is used as a “reference” pressure. In the absence of coronary artery disease, the pressure within the coronary tree should be equal to the aortic pressure. A coronary stenosis will introduce a resistance in the artery (Rs) and diminish both coronary pressure (Pd) and blood flow (Qs). In the presence of collaterals, total myocardial blood flow (Q) will be the sum of coronary flow through the coronary stenosis (Qs) and collateral blood flow (Qc). The original equations separate FFRcor, which represents a pressure measurement in the coronary artery distal to the stenosis, from FFRmyo which adds collateral
flow and the subsequent rise in distal coronary pressure. For clinical purposes, FFRmyo is now commonly equated to an “FFR” measurement, as it more comprehensively predicts myocardial perfusion pressure and thus the extent of myocardial ischemia.
flow and the subsequent rise in distal coronary pressure. For clinical purposes, FFRmyo is now commonly equated to an “FFR” measurement, as it more comprehensively predicts myocardial perfusion pressure and thus the extent of myocardial ischemia.
![]() FIGURE 14.1 A and B, Epicardial coronary artery versus microcirculation. Reprinted with permission from Fulton WF. Immersion Radiography Of Injected Specimens. Br J Radiol. 1963;36:685-688. |
Finally, the original FFR equation also incorporated right atrial pressure, as it represents a resistance distal to the myocardial capillary system. However, right atrial pressure is typically very low and, for simplicity reasons, can be negated in most clinical FFR measurements.4
Core Concept of FFR
The core FFR concept demonstrates the relationship between hyperemic myocardial blood flow and hyperemic coronary perfusion pressure, which are directly related (FIGURE 14.4). FFR is thus the theoretical construct of hyperemic coronary blood flow in the presence of a coronary stenosis divided by hyperemic coronary blood flow in the hypothetical case of absence of any coronary artery disease. Under those circumstances, any degree of stenosis or pressure drop will be compared with the normal state and thus the “normal” value for FFR is 1.
FFR is derived by dividing the pressure distal to a coronary stenosis or Pd by the pressure proximal to a stenosis or Pa under maximal hyperemia (for practical purposes aortic pressure is used and measured from the tip of the guiding catheter). As demonstrated in the illustration, a normal FFR is 1 in the absence of any coronary lesions. In the presence of a focal stenosis, there may be a pressure drop distal to the lesion; in this example the proximal or aortic pressure is 100 mm Hg and the distal coronary pressure is 70 mm Hg, yielding an FFR of 0.70. Note that the right atrial or venous pressure is assumed to be ˜0 mm Hg and thus not incorporated into the equation.
The importance of inducing myocardial hyperemia has been elegantly demonstrated by Gould et al in a dog model of coronary physiology (FIGURE 14.5).5 The coefficient for pressure loss across a stenotic lesion increases with the degree of stenosis. However, an increase in coronary flow velocity will produce a substantial increase in stenosis resistance and thus will cause an increase in pressure gradient. Depending on the severity of the stenosis, the pressure/flow relationship will result in various steepness of the curve with more significant stenosis producing a steeper curve. In the presence of a moderate stenosis, an increase in coronary flow velocity (which is achieved by inducing coronary hyperemia) will create a significant pressure gradient from 10 mm Hg at baseline to 37 mm Hg with hyperemia and thus “unmask” a significant coronary stenosis.
Coronary Pressure Remains Constant Throughout the Coronary Tree
The coronary pressure tree exhibits the hemodynamic principle of sustained pressure through a system in parallel (FIGURE 14.6). As resistance increases with lower luminal diameter, flow in the individual branches decreases. However, coronary pressure remains unchanged in the absence of significant coronary artery disease. This constitutes one of the reasons why it is preferable to measure coronary pressure instead of coronary flow.
Myocardial Perfusion Area Factored in by FFR
FFR is affected by both degree of coronary stenosis and myocardial bed supplied by the epicardial coronary vessel. FFR will be higher (and more likely negative) in vessels that do not supply a large myocardial bed (FIGURE 14.7). Consequently, a coronary stenosis has to be of higher severity to produce the same degree of FFR reduction when compared with a lesion located in a large coronary artery that serves a large amount of myocardium. This is a very important concept and frequently leads to a “visual versus physiology” mismatch, where coronary lesions appear to be severe by coronary angiography but only have a modest impact on producing myocardial ischemia (FIGURE 14.8).6 Other reasons that result in the same phenomenon are previous myocardial infarction with significant scar formation, microvascular disease, nondominant vessel or coronary branches that supply a small amount of myocardium.
FIGURE 14.7 exhibits that for the same degree of coronary stenosis, the FFR value can be very different based on the amount of viable myocardium that is supplied by the vessel. If half of the myocardial territory is replaced by scar tissue from a prior myocardial infarction, the resulting FFR will be substantially higher (indicating less myocardial ischemia) when compared with a large amount of viable myocardium.
Similarly, in the presence of significant collateral flow, the distal perfusion pressure will increase, leading to higher Pd and thus to a higher FFR, despite a similar degree of coronary stenosis (FIGURE 14.9). The fundamental advantage of using FFR, therefore, is the fact that it accurately reflects myocardial perfusion pressure and thus potential for myocardial ischemia, factoring in variables such as size of myocardial territory, viability of myocardium, and collateral blood flow beyond coronary stenosis severity. The impact of myocardial supply area on transstenotic hemodynamics as determined by FFR has been well demonstrated in a recent publication, which showed that coronary lesion severity and myocardial supply area were similarly predictive of FFR (FIGURE 14.10).6
FFR as a Continuum of Risk
Although clinical trials have initially used an FFR of ≥0.75 and later ≥0.80 to set the ischemic threshold as a cutoff point for an abnormal or “positive” FFR, it is important to remain cognizant that the risk associated with FFR is a continuum. The lower the FFR value, the more ischemia is present in the myocardial territory that is supplied by the coronary artery and conceptually the greater the risk of an adverse event. Like other biological variables, FFR thus represents a continuum of risk, with lower risk, the closer the FFR gets to the normal range of 1 (FIGURE 14.11). Conceptually, patients with very low FFR will have the greatest benefit from revascularization, whereas patients with a normal FFR will have no benefit at all and even potential harm from the
procedure. Theoretically, there is an optimal threshold at which point coronary revascularization will reduce the risk over medical therapy alone and that will represent the patient population that may benefit most from revascularization therapy. In a retrospective meta-analysis of published data reporting patient outcomes, Johnson et al demonstrated that this optimal threshold for FFR is 0.75 with a gray zone between 0.75 and 0.80 (FIGURE 14.12).7 Similar data have been reported from FAME-2, showing a curvilinear relationship between major adverse cardiovascular events (MACE) and FFR values in the cohort randomized to medical therapy alone (FIGURE 14.13).8 Based on these data, it is recommended to utilize FFR as a marker of overall cardiac risk and not solely as a decision tree for coronary revascularization.
procedure. Theoretically, there is an optimal threshold at which point coronary revascularization will reduce the risk over medical therapy alone and that will represent the patient population that may benefit most from revascularization therapy. In a retrospective meta-analysis of published data reporting patient outcomes, Johnson et al demonstrated that this optimal threshold for FFR is 0.75 with a gray zone between 0.75 and 0.80 (FIGURE 14.12).7 Similar data have been reported from FAME-2, showing a curvilinear relationship between major adverse cardiovascular events (MACE) and FFR values in the cohort randomized to medical therapy alone (FIGURE 14.13).8 Based on these data, it is recommended to utilize FFR as a marker of overall cardiac risk and not solely as a decision tree for coronary revascularization.
FFR Cutoff Point
Although there is a gray zone between 0.75 and 0.80, an FFR value of <0.80 has been accepted as the cutoff to guide clinical decision-making. A recent observational study by Adjedj et al examined 1459 patients in that gray zone who were treated medically (69%) versus coronary revascularization (31%). MACE was similar between the 2 groups. However, there was a strong trend toward higher mortality or MI (9.4% vs 4.8%, P = .06) and overall death (7.5% vs 3.2%, P = .059) in the medically treated group9 (FIGURE 14.14).
FFR TECHNIQUE AND INDUCTION OF HYPEREMIA
Basic FFR Setup
Confirm pharmacologic pretreatment with anticoagulation and IC nitroglycerin.
“Zero” guide and pressure wire ex vivo to atmosphere on table after flushing with saline (FIGURE 14.15).
Insert the wire into guide until the pressure sensor and proximal end of opaque wire tip are immediately outside of guide catheter either into aorta or coronary artery (FIGURE 14.15, upper right panel). If there is ostial disease, the guide can be pulled slightly back into the aorta, allowing equalization to take place using the aortic pressure.
“Equalize” or “Balance” just outside of guide (FIGURE 14.15, lower left panel). Confirm that the introducer needle has been removed and guide catheter is flushed free of contrast (FIGURE 14.15, lower right panel). The pressure transducer setup should be zeroed at the correct height. The guide catheter pressure should represent aortic pressure. The purpose of this step is to ensure that the pressure from the pressure sensor is equalized to the aortic pressure and thus the starting point is the same for both pressures.
Advance wire into the distal coronary artery or at least 2 to 3 cm distal to the lesion (FIGURE 14.16). This should be a point at which laminar blood flow is reconstituted distal to the stenosis. Again, confirm that the introducer needle has been removed and guide catheter is flushed free of contrast.
Note the resting Pd/Pa and confirm adequate waveforms. It is important to ensure that the aortic pressure is not ventricularized or distorted.
FIGURE 14.17: Administer hyperemic agent (preferably IV or IC adenosine, see Table 14.1 and FIGURE 14.19 for properties and dosing of these medications).
Confirm maximal hyperemia—1 to 2 minutes for IV adenosine and 20 seconds for IC adenosine. For IC adenosine, the guide catheter must be well engaged during the injection to ensure adequate delivery of adenosine; it can be slightly disengaged thereafter.
Measure the FFR—The length of the recording is generally ˜2 minutes with IV adenosine. For IC adenosine, the recording should last about 30 seconds to 1 minute. The baseline, maximal hyperemic phase, and recovery phase should be recorded. The FFR value is measured as the pressure difference at the nadir of the Pd and Pa tracings.
Confirm accuracy with pressure pullback (FIGURE 14.18) across stenosis and verify that no drift is present with remeasuring Pd/Pa at tip of guide catheter. The 2 pressures should be identical if no drift has occurred.
Hyperemic Agents
Although intravenous adenosine and intracoronary adenosine are used most often to achieve hyperemia, intracoronary papaverine and nitroprusside and intravenous dobutamine have been used for this purpose. However, they are less popular owing to limiting properties with these medications. Intracoronary papaverine is as effective as adenosine but is associated with QT prolongation and (rarely) torsades de pointes. Additionally, it cannot be used concomitantly with heparin infusions. Dobutamine may cause tachycardia, increasing myocardial oxygen demand; nitroprusside can precipitate undesired hypotension (Table 14.1).
Inducing Hyperemia With Adenosine
Adenosine is the most frequently used pharmacologic agent for inducing hyperemia owing to its short half-life and limited side effects (Table 14.2). Adenosine can be administered IV or IC. Adenosine IV is administered by nursing through a peripheral IV and should be dosed at 140 µg/kg per minute for 2 to 4 minutes. Adenosine IC should be given in bolus doses of 100µg in the right coronary artery and 200 µg in the left coronary artery (FIGURE 14.19).10 A fluctuating baseline with IC adenosine suggests submaximal hyperemia.
TABLE 14.1 Hyperemic Agents | ||||||||||||||||||||||||||||||||||||
---|---|---|---|---|---|---|---|---|---|---|---|---|---|---|---|---|---|---|---|---|---|---|---|---|---|---|---|---|---|---|---|---|---|---|---|---|
|
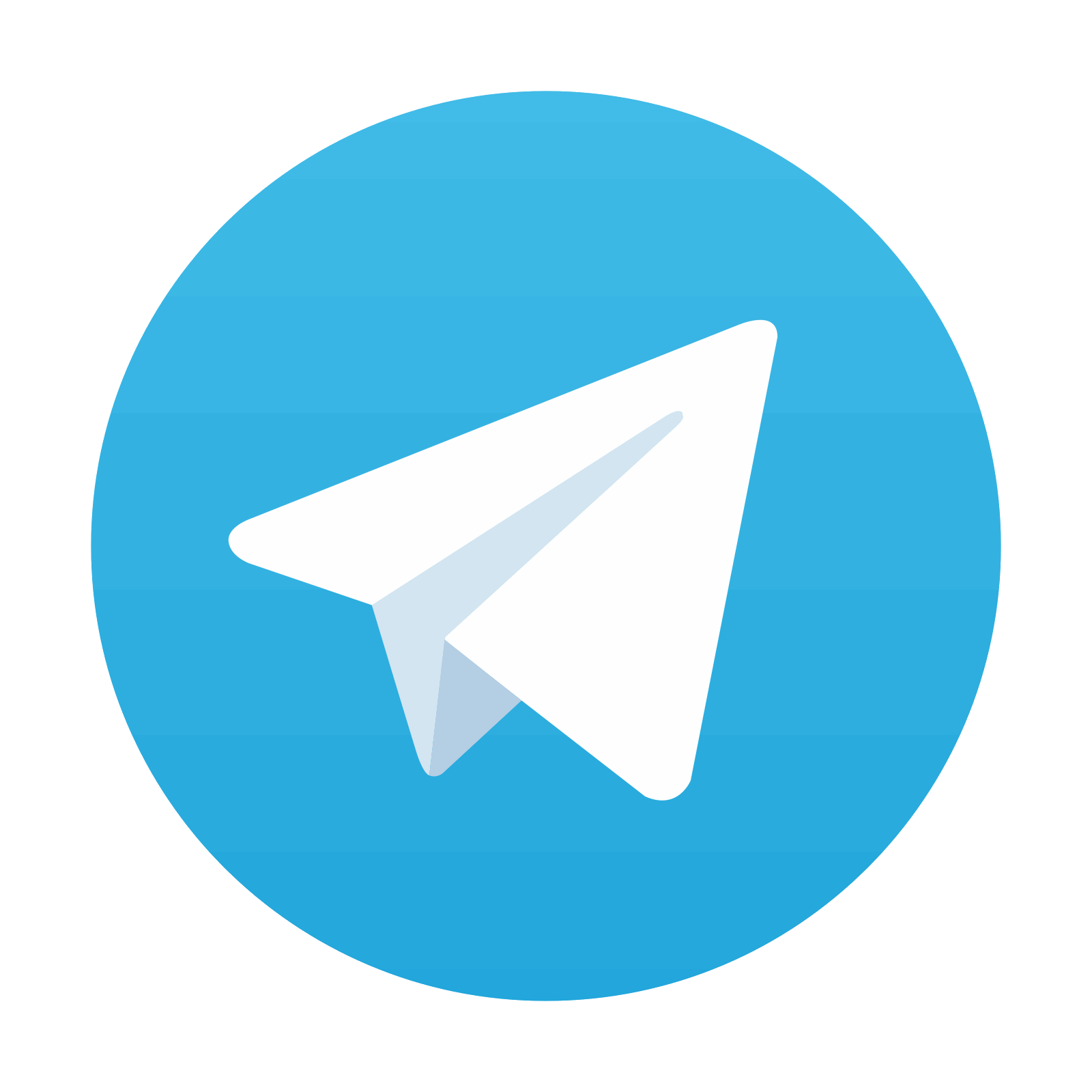
Stay updated, free articles. Join our Telegram channel

Full access? Get Clinical Tree
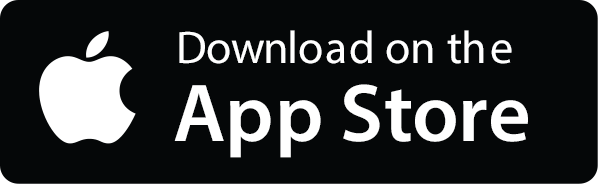
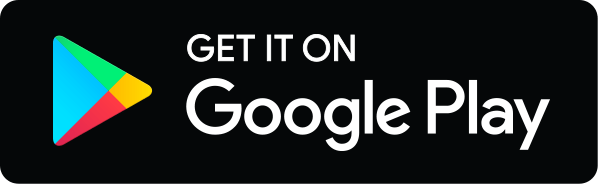