Multiple Organ Failure
HISTORICAL PERSPECTIVE: EVOLVING CONCEPTS ON THE PATHOGENESIS OF MULTIPLE ORGAN FAILURE
Our increasing ability to keep severely injured trauma patients alive resulted in the clinical syndrome called postinjury multiple organ failure (MOF). As advances in prehospital and acute hospital care conquered “the golden hour” and the trimodal distribution of trauma deaths described in the 1970s and 1980s slowly flattened its first mode, MOF emerged as the leading cause of late trauma death.1–3
Much of advance in the treatment of trauma and shock has been stimulated by military experience.4 In World War I, soldiers’ death on battle was initially attributed to toxins released by dead or dying tissue (Table 61-1).5 Cannon et al, via their observations on the battlefield in 1917, expanded this concept to question the role of hypovolemia; however, it was not until the 1930s that reduced circulating blood volume was recognized as the cause of shock and mortality.6 Casualties in World War II were initially resuscitated with plasma and later with blood. This continued in Korea where blood and plasma were administered until blood pressure returned to normal. In addition to rapid transport for definitive care in field medical units, blood and plasma resuscitation improved battlefield survival but resulted in late deaths due to oliguric renal failure. In the 1960s, Shires et al. proposed that extracellular fluid deficits (third space losses) compounded traumatic shock and demonstrated in animals that survival improved with balanced salt solutions.7 Consequently, crystalloid resuscitation was added to blood and plasma resuscitation in the Vietnam War and resuscitation end points focused on maintaining adequate urine output. Helicopter evacuation also enabled rapid transport of casualties and the overall mortality rate decreased. Although late deaths from renal failure declined, a new entity termed “shock lung” emerged as the primary cause of late deaths.5 This new disorder became recognized in civilian trauma centers as the adult respiratory distress syndrome (ARDS).8 In the 1970s, subsequent improvements in advanced organ support such as mechanical ventilation (MV), vasoactive drugs, total parenteral nutrition, and hemodialysis armed physicians with better treatment to sustain the critically ill. Death from isolated pulmonary failure became rare, and a new syndrome of “multiple, progressive, or sequential systems failure” was recognized.9
TABLE 61-1 Historical Perspective of Postinjury Multiple Organ Failure
Eiseman et al. in Denver coined the term “multiple organ failure” in 1977, and provided the first clinical description of 42 patients with progressive organ dysfunction.10 During the late 1970s MOF was thought to be the “fatal expression of uncontrolled infection.”11 In addition, at this time, studies indicated that organ failure was a bimodal phenomenon with distinct patterns: rapid single-phase MOF due to massive tissue injury and shock or delayed two-phase MOF due to moderate trauma and shock followed by delayed sepsis.12,13 While infection remained a frequent cause of organ failure, by the mid-1980s it was convincingly shown that organ failure occurred in the absence of infection and the concept of “generalized auto-destructive inflammation” emerged.14,15
By the 1990s, noninfectious inflammatory models of MOF became the focus.16 In this conceptual framework, patients are resuscitated into a state of early systemic hyperinflammation, referred to as the systemic inflammation response syndrome (SIRS) (Fig. 61-1). A mild response is presumed to be beneficial and resolves in most patients as they recover. In the “one-event” model, a massive traumatic insult overwhelms the capacity of the patient to respond to resuscitation and precipitates organ failure. In the more common, alternative “two-event” model, patients who are initially resuscitated to a moderate response (primed) are vulnerable to a second (activating) event that can also precipitate hyperinflammation leading to early organ failure. The timing of the second hit may vary from occurring shortly after the first hit, where the two are indistinguishable, to a delay, where the second event is conspicuous 12–36 hours later. Patients who escape early MOF become susceptible to infection during the so-called compensatory anti-inflammatory response syndrome (CARS). If infected during this window, patients are at risk of developing late MOF. Other second hits include abdominal compartment syndrome (ACS), sepsis, fat embolus, MV, blood transfusions, long bone fixation, and secondary surgery.17
FIGURE 61-1 Pathogenesis of multiple organ failure.
More recently, a new model has emerged in which it is believed the injury triggers simultaneous, opposite responses: the proinflammation (SIRS) and the anti-inflammation (CARS) (Fig. 61-1).18 The term compensatory inflammation is in fact considered a misnomer as CARS appears to occur in response to the injury (not to SIRS). In this paradigm, the development of MOF is related to the intensity and the balance between these inflammatory responses to trauma. Severe SIRS, due to unbalanced early proinflammation, causes early MOF and can eventually result in a fulminant proinflammatory death. On the other hand, early anti-inflammation is directed at limiting proinflammation, creating a preconditioned state where the host is protected against second hits, and hastening the healing process. Anti-inflammation is also associated with apoptosis and depression in adaptive immunity. When countering unbalanced proinflammation, persistent anti-inflammation can result in severe CARS. This sets the stage for immunoparalysis, impaired wound healing, recurrent nosocomial infections, and late MOF, which can cause an indolent death.18
This new construct evolved from clinical observations and experimental studies at the bench level, and remains the foundation of contemporary MOF research. Research during the past decade has progressed toward further explaining these inflammatory mechanisms at the cellular and subcellular levels. New strategies to reduce secondary insults and to modulate the inflammatory responses in the early and late postinjury periods are currently undergoing intense clinical investigation. In sum, the presentation and outcome of postinjury MOF has changed considerably over the past 15 years. Yet, as we will describe in the next sections, MOF victims continue to suffer with high morbidity and long hospital stays and consume an inordinate amount of resources.
EPIDEMIOLOGY AND CLINICAL RELEVANCE
The epidemiological descriptions of MOF vary widely. The reported incidence of postinjury MOF varies from as low as single digit numbers to values approaching 40%, with case-fatality rates also varying within similarly large ranges.19 Recent studies have been inconsistent regarding changes in the incidence or the mortality associated with postinjury MOF, with some reporting no change in the incidence but a decreased mortality20 while others reporting both decreased incidence and mortality compared with historical controls.21 Reviews of patients prospectively included in our Denver MOF dataset showed a significant reduction in the incidence of MOF, with the most dramatic decrease being observed from 1998 to 2004, while MOF case-fatality rate has not shown a significant decrease.22
To determine whether the incidence reduction was due to a decline in disease severity of the patients admitted to our center, we examined changes in the major risk factors of postinjury MOF: age, Injury Severity Score (ISS), and number of RBC units transfused within the first 12 hours.23 Patients’ ISS and age increased over time while the proportion of patients requiring more than 6 U of RBC in the first 12 hours decreased after the implementation of a new protocol of judicious blood product utilization. The decrease in blood products use over time remained statistically significant after adjusting for age and ISS.22
In the early 1990s, about half of the MOF cases presented early (<3 days after injury) while the remaining cases presented later, most often around 7 days. This bimodal presentation pattern, first recognized by Faist et al. in 1983,13 seems to be changing in recent years, with the second peak gradually leveling out (Fig. 61-2), possibly as a result of improved prevention and treatment of second insults.
FIGURE 61-2 Temporal distribution of MOF over time.
The risk factors for early MOF include an ISS >24, emergency department (ED) systolic blood pressure less than 90 mm Hg, blood transfusions >6 U within 12 hours of injury, and a lactate level >2.5 mmol/L measured between 12 and 24 hours after injury.24 Major infections are more likely to be a symptom that followed (sometimes worsening organ dysfunction) than a trigger that precipitated early MOF. Early MOF is typically associated with a higher incidence of heart failure than late MOF.
Late MOF (>72 hours postinjury), consistent with the distinct two-event construct, appears to be related to the increased risk of infection and systemic sepsis that is attributed to the relative immunosuppression associated with severe trauma. Independent risk factors for late MOF include age over 55 years, blood transfusion more than 6 U within 12 hours of injury, early base deficit more than 8 mEq/L in the first 12 hours postinjury, and a lactate level greater than 2.5 nmol/L measured between 12 and 24 hours postinjury.24 Although the risk factors for both early and late MOF included blood transfusion, base excess, and lactate levels, the shock indices were stronger risk factors for early MOF, whereas major infections were more frequently classified as triggers in patients who developed late MOF. Patients with late MOF also had a higher incidence of liver failure but a lower mortality (16%) than early MOF patients. Mortality is similar for early and late MOF as well as other adverse outcomes such as intensive care unit (ICU) length of stay and ventilation time.
Regarding individual organ patterns, the lung is virtually always the first organ to show evidence of dysfunction in the absence of preexisting disease.25 Lung dysfunction precedes heart dysfunction by an average of days, liver dysfunction by
days, and kidney dysfunction by
days. The number of involved organs and the severity of other organ dysfunction also seemed dependent on the severity of lung impairment. Of course, these conclusions are limited by our measurements of individual organ dysfunctions, that is, our measurements of lung dysfunction are far more sensitive to function changes than our measurements of liver or kidney function.
While MOF incidence seems to be decreasing, these patients continue to demand an excessive amount of hospital resources. Among MOF patients, the proportion of patients with less than 21 ventilator-free days (VFD) and less than 14 ICU-free days (IFD) has significantly increased steadily over the past decade from 90% to 100%. Indeed, from 1994 to 1998, there were 138 postinjury MOF patients in our ICU, who required 3,428 ICU days and 2,785 MV days. While from 1999 to 2003, there were less MOF patients (, an 11% decrease), they required 3,548 ICU days and 2,812 MV days. In brief, despite reduction in incidence, MOF patients are responsible for an even larger amount of resources than in the past. Each MOF patient required a median number of 21 ICU days and 17.5 MV days from 1994 to 1998, while in the 1998–2003 period, the median number of ICU days was 26 and that of MV days was 21. Collectively, these numbers strongly support that despite a reduction in the MOF incidence, these patients still suffer through long hospital stays, have high morbidity, and consume an inordinate amount of resources.
PATHOPHYSIOLOGY
The First Hit: Initial Inflammatory, Hormonal, and Immune Responses to Trauma
The model of postinjury MOF, described in a previous section, was derived from a two-way translational model in which information was exchanged between epidemiological studies, predictive models, and basic investigations using in vivo and in vitro models. As mentioned above, severe trauma patients are resuscitated into an early state of systemic inflammation or SIRS. The American College of Chest Physicians and the Society of Critical Care Medicine defined SIRS as two or more of the following criteria26: (1) temperature <36.8°C or >38.8°C; (2) heart rate >90/min; (3) respiratory rate >20 breaths/min or PCO2 <32 mm Hg; and (4) WBC <4,000/mL or >12,000 mL or greater than 10% immature forms.
Figure 61-3 illustrates the current framework for the immunoinflammatory response to trauma. SIRS is the manifestation of the immunoinflammatory activation that occurs in response to ischemia–reperfusion (I/R) injury and released factors from disrupted tissue, that is, the damage-associated molecular patterns (DAMPs). In trauma, hemorrhagic shock following injury causes whole-body hypoperfusion, followed by subsequent reperfusion during resuscitation, which circulates cytokines, proinflammatory lipids, and proteins that prime polymorphonuclear neutrophils (PMNs) within 3–6 hours after injury.17 The CARS includes (a) apoptotic loss of intestinal lymphocytes and epithelial cells, (b) PMN and monocytic deactivation, (c) anergy characterized by suppressed T-cell proliferative responses, and (d) a shift from a THI to a TH2 phenotype.18 In the past, most studies attributed SIRS to hyperactivity of the innate immune system and CARS to dysfunction of the adaptive immune system, but recent evidence suggests that interactions between the innate and adaptive immune systems induce both SIRS and CARS and that the predominant mechanism for MOF is the balance between proinflammatory and counterinflammatory states.27
FIGURE 61-3 Immunoinflammatory response to trauma.
Role of the Gut
The gut is the last organ to have its circulation restored after ischemia, and is thought to play a pivotal role in the pathogenesis of postinjury MOF.18,28 Initially, the dominant hypothesis linking the gut to MOF was related to bacterial translocation: intestinal mucosa increased permeability allowed gut bacteria and/or endotoxin to be translocated to the circulation leading to sepsis and MOF. However, inconsistent results regarding the role of bacteria and endotoxin in the genesis of MOF led to experiments demonstrating that the mesenteric lymph acted as a bridge between the gut and the systemic circulation, allowing gut-derived inflammatory mediators and primed neutrophils to reach the systemic circulation. Via the thoracic duct, these mediators reach the pulmonary circulation and affect the lungs before any other organ, which is consistent with human studies demonstrating that postinjury respiratory dysfunction is an obligate event that precedes heart, liver, and kidney failure.29,30
Role of the PMN and Other Cells
PMN kinetics are different between MOF patients and non-MOF patients. Both groups develop neutrophilia at 3 hours postinjury; however, in patients who develop MOF there is a rapid neutropenia between 6 and 12 hours postinjury suggesting end-organ sequestration.31 PMNs marginated to end organs cause direct local cytotoxic cellular effects via degranulation, and the release of nitric oxide and reactive oxygen species. They also have remote systemic proinflammatory cytokine effects, releasing proinflammatory mediators including IL-8, IL-6, and TNF-α. In non-MOF, neutrophil priming and neutrophilia are not followed by neutropenia, and resolve over the next 36 hours without end-organ damage.32
Following trauma there is an immediate increase in adhesion molecules, including L-selectin and CD18, which allow PMNs to slow and roll along the endothelium and marginate out of circulation.17,33 Antibodies directed against the CD11b/CD18 components of the adhesion receptor complex between leukocytes and endothelium significantly attenuate lung injury and prevent the neutropenia associated with tissue sequestration during sepsis, further supporting that adherence of neutrophils to endothelium is a critical step in local tissue injury.33
Circulating monocytes and tissue macrophages also become primed after severe injury and most authorities agree that microvascular endothelium has an integral role in postinjury priming of the innate inflammatory response.32 Finally, other studies have demonstrated that the organ damage is also dependent on complement activation through the classical pathway mediated by natural IgM antibody produced by B1 lymphocytes.27
Cytokines
The hemodynamic, metabolic, and immune responses are mainly regulated by endogenous mediators referred to as cytokines, produced by diverse cell types at the site of injury and by systemic immune cells.33 Cytokines bind to specific cellular receptors resulting in activation of intracellular signaling pathways that regulate gene transcription and influence immune cell activity, differentiation, proliferation, and survival. They also regulate the production and activity of other cytokines, which may either augment or attenuate the inflammatory response. There is also significant overlap in bioactivity among different cytokines.33 Cytokines can be classified into proinflammatory (TNF-α, MIP, GM-CSF, IFN-Y, IL-1, IL-2, IL-6, IL-8, IL-17, etc.) and anti-inflammatory cytokines (IL-4, IL-10, IL-13), which downregulate synthesis of the proinflammatory cytokines.33
Recently, Jastrow et al. assessed the temporal cytokine expression (every 4 hours during 24 hours postinjury) during shock resuscitation in severely injured torso trauma patients.34 Median concentrations of IL-1 receptor antagonist (IL-1Ra), IL-8, eotaxin, granulocyte colony-stimulating factor (GCSF), granulocyte-macrophage colony-stimulating factor (CSF), inducible protein 10 (IP-10), monocyte chemotactic protein-1, and macrophage inflammatory protein-1 (MIP-1) were significantly greater in the MOF compared with those in the non-MOF subgroup at each time interval. Adams et al. demonstrated that IL-8 can activate PMNs via two different receptors, and differential early expression of these receptors may provide an explanation for why only selected patients develop MOF.35
The cytokine pattern after trauma also differs for patients developing early (less than 3 days) versus late (>3 days) MOF. Analysis of cytokine serum biomarkers revealed that whereas early onset MOF was associated with an initial peak of IL-6 and IL-8 followed by a comparatively rapid return to baseline values, late MOF was characterized by a significant secondary increase of the proinflammatory cytokines IL-6 and IL-8.36 Moreover, plasma levels of soluble tumor necrosis factor-alpha receptors (sTNF-R p55, sTNF-R p75) progressively increased during the 10-day observation period, and higher values were associated with lethal outcome.
Although inflammatory mediators’ levels vary greatly according to injury-related factors as well as the patient’s individual characteristics, most studies agree that the changes start very early postinjury. This underscores the importance of measuring inflammatory mediators very early and at short intervals after injury.27 Indeed, a recent German study including 58 multiple injured patients (ISS >16) found that IL-6, IL-8, and IL-10 differentiate patients with MOF (n = 43) and those without MOF (n = 15) within 90 minutes post trauma.37
PAMPS, Alarmins, and DAMPS
Pathogen-associated molecular patterns (PAMPs) are exogenous microbial molecules that alert the organism to pathogens and are recognized by cells of the innate and acquired immunity system, primarily through Toll-like receptors (TLRs), and activate several signaling pathways (e.g., NF-κB). A new awareness of the close relationship between trauma- and pathogen-evoked responses recently emerged and the term “alarmin” was proposed to differentiate the endogenous molecules that signal tissue and cell damage.38 Together, alarmins and PAMPs comprise the DAMPs. Alarmins are rapidly released after nonprogrammed cell death but not by apoptotic cells. They recruit and activate receptor-expressing cells of the innate immune system, and also promote adaptive immunity responses. An example of an alarmin is the high-mobility group box 1 (HMGB1), a nuclear protein which binds to nucleosomes and promotes DNA bending. HMGB1 has been associated with SIRS and end-organ damage in animals, and shown to be elevated in trauma patients more than 30-fold above healthy controls as early as 1 hour postinjury.38–40
A recent study by Zhang et al. showed that injury releases mitochondrial DAMPs (MTDs) into the circulation with functionally important immune consequences. The authors suggest that since mitochondria are evolutionary endosymbionts derived from bacteria, the released MTDs have conserved similarities to bacterial PAMPs. Thus, these MTDs signal through innate immune pathways identical to those activated in sepsis to create a sepsis-like state. This mechanism may provide the key link between trauma, inflammation, and SIRS.41
Toll-Like Receptors
TLRs are transmembranal proteins present in most body cell types, which form the major pattern recognition receptors that transduce signals in response to DAMPs.42 They were shown to participate in the recognition of endogenous alarmins released from damaged tissues after I/R injuries. Innate immune system responses are then initiated, including NF-αB activation, cell activation, and proinflammatory cytokine production.42 Inhibition of TLR2 or TLR4 seems to be beneficial in I/R injury in certain organs (hepatic, renal, cerebral, and heart) but not in gut I/R injuries. It is conceivable that because the gut mucosa is continuously exposed to local bacterial endotoxins, local TLRs are uniquely regulated to prevent persistent inflammatory activity. In spite of the distinct roles played by TR2 and TR4 in individual organs, our understanding of how the several TLR members interact among each other in I/R injuries is still limited, which may hinder the interpretation of interventions aimed at a specific TLR.42
Heat Shock Proteins (HSPs)
HSPs are a family of molecular chaperones (e.g., Hsp70 and Hsp90) necessary for the folding of newly synthesized proteins in the cell and also for the protection of proteins during exposure to stressful situations such as heat shock, which causes proteins folded previously to unfold.43 Extracellular HSPs can interact with several receptors (including TLRs), and have been implicated in inducing secretion of proinflammatory cytokines. However, highly purified HSPs do not show any cytokine effects suggesting recombinant HSP products may be contaminated with PAMPs that appear to be responsible for the reported in vitro cytokine effects of HSPs.43 Thus, the reported HSP’s role in antigen presentation and cross-presentation and in vitro cytokine functions may be attributable to molecules bound to or chaperoned by HSPs.
Complement
Complement system activation occurs immediately after trauma leading to production of biologically active peptides.28 Proinflammatory peptides include C3a, C3b, C4b (chemotaxis of leukocytes; degranulation of phagocytic cells, mast cells, and basophils; smooth muscle contraction and increased vascular permeability), and C5b-9 or membrane attack complex that leads to lysis of the target cells at the end stage of the complement activation cascade. Furthermore, complement activation results in the production of oxygen free radicals, arachidonic acid metabolites, and cytokines. Several studies suggest that complement activation, especially serum C3 and C3a levels, reflects severity and treatment of injury.28
Oxidative Stress
Oxidative stress occurs when the level of toxic reactive oxygen intermediates (ROIs) overcomes endogenous antioxidant defenses as a result from either oxidant production excess or antioxidant defense depletion.44 ROIs are normally generated by mitochondrial oxidation, metabolism of arachidonic acid, activation of reduced nicotinamide adenine dinucleotide phosphate (NADPH) oxidase in phagocytes, and activation of xanthine oxidase (XO) and play important roles in cellular homeostasis, mitosis, differentiation, and signaling.28 Excess ROI, however, causes direct oxidative injury to cellular proteins and nucleic acids, and cell membrane destruction by inducing lipid peroxidation.28,42,44
I/R injury leads to significant disturbances in the production of ROIs.28,42 During ischemia, hypoxemia leads to a shift from aerobic to anaerobic metabolism, with consumption of and decreased production of adenosine triphosphate (ATP). As ATP decreases, changes in cell membrane permeability result in intracellular Na+ increase causing cellular swelling and cell membrane damage. ATP reduction also alters cytosolic Ca2+ levels leading to phospholipase and protease activation and cell damage. Increased ATP hydrolysis is followed by rising levels of AMP and purine metabolites. As reperfusion increases O2 availability, oxidation of purines produces urate and superoxide radicals, which can then produce the toxic hydroxyl radicals. In addition, superoxide radicals may be generated by a plasma membrane–associated NADPH oxidase system, which can be activated by macrophages, neutrophils, and other immunologic cells.45 ROI secreted from PMNs after I/R injury induces cytokines, chemokines (IL-8), HSP, and adhesion molecules (P-selectin, ICAM-1) leading to cell and tissue damage.28
Under normal conditions, NO production greatly exceeds O2− production in the endothelial cell (EC). However, with reperfusion, the balance between NO and O2− shifts in favor of O2−. The relatively low level of NO by constitutive endothelial nitric oxide synthase (NOS-I) reacts with the now abundant O2− to generate peroxynitrites, leaving little NO available to reduce arteriolar tone, prevent platelet aggregation, and minimize PMN adhesion to EC.46 In addition, NO seems to upregulate the production of proinflammatory cytokines.28 Thus, altering the redox state of the cell may contribute to the ongoing inflammatory cytokine production and progression to MOF.42 ROIs also play a role as second messengers in the intracellular signaling pathways of inflammatory cells, in particular, activation of NF-κB and activator protein 1 (AP-1), which can be activated by both oxidants and antioxidants depending on the cell type and on intracellular conditions.44
Endogenous antioxidant defenses, including enzymatic (superoxide dismutase, catalase, glutathione peroxidase) and nonenzymatic (vitamins E and C, provitamin A, glutathione, bilirubin, urate) groups that combat oxidative stress, have been the focus of interventions to modulate the inflammatory response to critical illness, as detailed later.
Hormones
Traditionally, the injury stress response was viewed as a neuroendocrine reflex mediated via counterregulatory hormones (cortisol, glucagon, and epinephrine) that altered substrate metabolism while the body is in a state of repair. Adrenaline is released and suppresses insulin secretion but stimulates secretion of growth hormone and renin, proteolysis and glycogenolysis, enhancing hepatic-mediated gluconeogenesis.33 Glucagon is released by pancreatic islet cells that increase hepatic glucose production from substrate that arises from tissue catabolism. The liver produces acute phase reactants such as opsonins (CRP), protease inhibitors, hemostatic agents (fibrinogen), and transporters (transferrin).33
The hypothalamic–pituitary–adrenal (HPA) axis is activated during stress, stimulating the release of adrenal corticotropic hormone (ACTH) from the pituitary gland, which induces the release of cortisol from the adrenal cortex.47 The HPA axis and the immune response are intrinsically linked in a negative feedback loop in which activated immune cells produce specific cytokines that activate the HPA axis increasing cortisol release that in turn suppresses the immune response and further cytokine release.47 Cytokines also act directly on the adrenal cortex and on glucocorticoid receptors (GR), present in most cells. In addition, different types of stress (sepsis, trauma, elective surgery) seem to be associated with the release of distinct mediators that may inhibit or stimulate cortisol production by acting on the HPA axis, adrenal cortex, and/or GR.
The HPA response is pivotal for survival as adrenal insufficiency (AI) increases the mortality of critically ill or injured patients.47 In the Section “Interventions,” we will return to this important topic in the treatment and prevention of MOF.
Less clear is the role of sexual hormones. Choudhry et al. in Alabama have studied extensively the influence of gender in the response to trauma and hemorrhage (TH) in animal models.48 They suggested that immune and cardiac dysfunctions after TH are depressed in adult males and ovariectomized/aged females, while both are maintained in castrated males and in proestrus females. The female reproductive cycle seems to be an important variable in the regulation of lung injury after TH.49 One of their most recent studies showed that enhanced hepatic heme oxygenase (HO-1) in proestrus- and estradiol-pretreated ovariectomized females modulates inflammatory responses and protects liver following TH.49
In contrast with the animal studies, clinical investigations have shown controversial results regarding a protective effect of female gender. In a 2006 study by the Alabama group, female polytrauma victims younger than 50 years with an ISS >25 suffered significantly less MOF and sepsis and had lower plasma cytokines compared with age-matched males.50
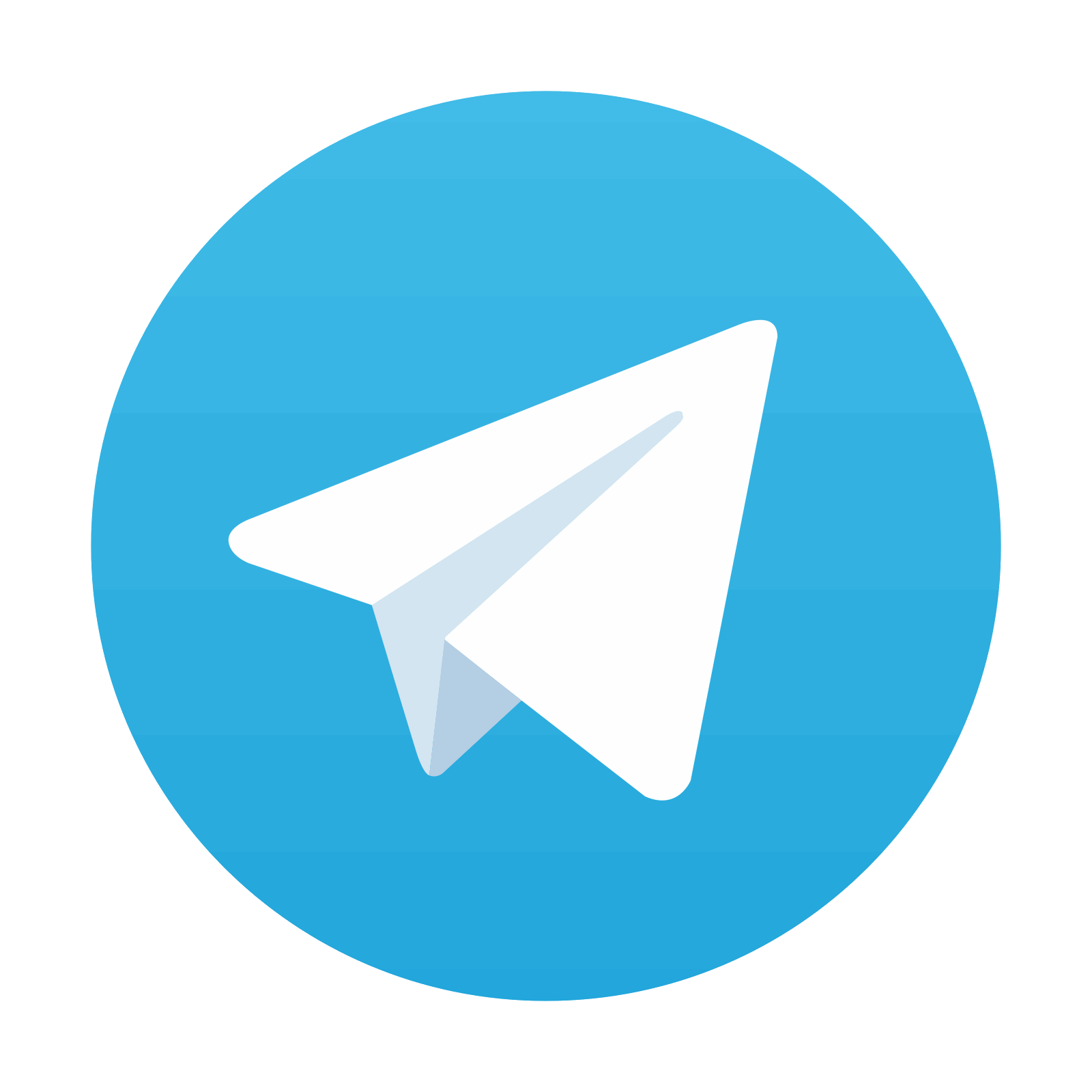
Stay updated, free articles. Join our Telegram channel

Full access? Get Clinical Tree
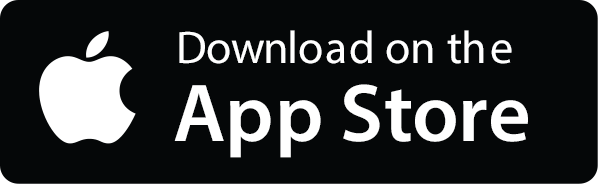
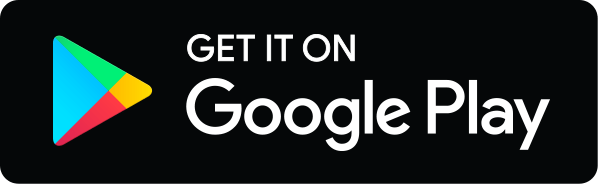