12 Multimodality Cardiac Imaging—When Is Echo Not Enough?
Basic Concepts
• The field of cardiovascular imaging has undergone tremendous improvements over the past decade, with the advent of advanced echo modalities (deformation, contrast, and three-dimensional imaging), advances in nuclear cardiology (single-photon emission computed tomography [SPECT], positron emission tomography [PET], and computed tomography hybrid systems) and the emergence of the new technologies of cardiac computed tomography (CCT) and cardiac magnetic resonance (CMR) and molecular imaging.
• Echocardiography maintains a central position in modern cardiac diagnosis and management, being the first choice of imaging method to assess cardiac anatomy and function. It provides portable, widely available, safe, and inexpensive imaging.
• For the majority of patients, a transthoracic echocardiogram alone is sufficient, providing all the imaging information required for making clinical decisions.
• In many clinical scenarios, the standard echo examination is not enough and clinicians need to identify when and how to combine it with other imaging tests to answer clinical questions (Tables 12-1 and 12-2).
• However, it is important not only to obtain the most complete and valid clinical information, but also to ensure that patients do not get inappropriate, unnecessary, and nondiagnostic imaging tests.
• It is critical to match rapid technological developments with a rigorous scientific evaluation to demonstrate the impact of multimodality tools on clinical decision making and patient outcome.
Cardiac Computed Tomography
• Advances in multidetector computed tomography with the introduction of multi-slice spiral computed tomography (MSCT) and dual-source CT have radically changed the role of CT in cardiac imaging.
• Electrocardiographic (ECG) gating is another important innovation that has allowed the correlation of anatomic information with the cardiac cycle phase.
• Retrospective ECG gating is a technique in which CT data are continuously acquired and the ECG signal is used during postprocessing analysis to correlate the information with the corresponding phases of cardiac contraction.
• Single-source MSCT (64-, 256-, or 320-slice systems) performs spiral data acquisition that is processed to generate a three-dimensional (3D) dataset. Images are usually presented as cross-sectional slices of the heart in any desired plane or as 3D reconstructions.
• CCT has excellent spatial resolution (maximal isotropic resolution of 0.5–0.8 mm3) and very good image quality.
• CCT offers excellent morphologic depiction of the heart anatomy, myocardium, heart valves, coronary arteries, and bypasses.
• CCT allows accurate 3D anatomic evaluation of associated extracardiac structures, including the great arteries, proximal branch pulmonary arteries, and pulmonary venous and systemic connections.
• CCT detects and quantifies calcification of cardiac structures, including the coronary arteries (the calcium score is used to stratify the risk for coronary atherosclerosis) and the pericardium (relevant in the diagnosis of constrictive pericarditis).
• Dual-source multidetector scanners have a maximum of 2 × 64 rows, providing 2 × 128 slices.
• With retrospective ECG gating, dual-source CT provides better temporal resolution (75–83 ms) than single-source CT (165 ms) but is still clearly inferior to both two-dimensional echocardiography (2DE) and CMR.
• Computed tomography coronary angiography (CTCA) has received a great deal of attention in recent years since technologic developments made possible the simultaneous visualization of the coronary lumen and vessel wall (Table 12-3).
• Contrast-enhanced CT is an emerging technology that examines myocardial perfusion. Using first-pass imaging (adenosine-augmented), it may detect perfusion defects. With a second scan a few minutes later, delayed enhancement may be analyzed for detection of myocardial infarction and scars.
TABLE 12-3 CURRENT AND EMERGING CLINICAL APPLICATIONS OF THE MOST COMMONLY USED CARDIAC IMAGING MODALITIES
CCT | CMR | Nuclear Imaging Modalities (SPECT and PET) |
---|---|---|
Current Clinical Applications | ||
• Exclusion of CAD in the setting of medium to high-risk but asymptomatic patients or in patients with atypical symptoms | ||
Emerging Applications | ||
TAVI, transcatheter valve implantation; CHD, congenital heart disease.
CT: Limitations and Disadvantages
• Relevant radiation exposure: between 7 and 21 mSv (equivalent to 100–600 X-rays) depending on the CCT modality and use of radiation-reducing algorithms. This limits use of CCT for longitudinal follow-up.
• Use of iodinated contrast agents: adverse reactions include nephropathy and potentially life-threatening allergic reactions.
• Need for breath-holding: respiratory motion causes artifacts and degradation of image quality. However, the breath-holding duration is nowadays very short (<15 s) due to faster gantry rotation times and more efficient detectors.
• Difficulties in case of tachycardia or unstable heart rhythm: to achieve the best image quality with ECG gating, the heart rate should be less than 65 beats/min. Thus, beta-blockers are usually given to patients with heart rates over 75 beats/min.
• Since data are acquired from several heartbeats, artifacts may be produced at the transition zone between gantry rotations.
• Retrospective ECG gating uses low-pitch acquisition, resulting in longer exposure time and higher radiation dose. Since acquisition takes longer, more respiratory artifacts may be produced.
Cardiovascular Magnetic Resonance
• CMR is a rapidly emerging noninvasive imaging modality that consists of several techniques that can be performed separately or in various combinations during a patient examination.
• Several pulse sequences may be used for CMR. A pulse sequence is a combination of transmitted radiofrequency pulses and magnetic gradients in the presence of a strong magnetic field, from which a series of received radiofrequency echoes are obtained and processed into an image.
• The radiofrequency signal emitted by hydrogen nuclei as it returns to baseline energy state has an amplitude (which depends on tissue properties) and a phase (which depends on the velocity and direction of flow of the water molecules).
• The most frequently used pulse sequences for CMR are:
• Spin echo and turbo spin echo sequences (T1, T2, with or without fat saturation) produce images with high tissue contrast and spatial resolution without the need for contrast agents. Flowing blood appears black, and more stationary tissues are displayed in shades of gray.
• Gradient echo sequence provides high temporal resolution (50 ms), allowing cine display. Flowing blood appears bright, and stationary tissue is displayed in gray. Flow turbulence generates signal void, allowing the detection of regurgitation.
• Steady-state in free precession (SSFP) pulse sequence: contrast is produced based on the ratio between T1 and T2, providing better signal-to-noise ratio and contrast between blood and myocardium. Similarly to conventional gradient echo sequences, flowing blood appears bright and flow turbulence produces signal void.
• Phase-contrast pulse sequence (or velocity mapping) is based on the accumulated phase of moving protons. Each pixel is displayed as a velocity rather than a signal magnitude. That allows flow calculations in vessels and cardiac valves by integration over time of the product of mean velocity with cross-sectional area.
• SSFP is currently the most widely used technique for CMR cine imaging. It provides high-quality information regarding heart anatomy, chamber volume, valve anatomy, and motion.
• SSFP produces 2D images in any desired plane having multiple phases (frames) throughout the cardiac cycle with high spatial resolution (in-plane resolution of 1.4 × 1.8 mm) and reasonably high temporal resolution (25–50 ms).
• Gadolinium-based magnetic contrast agents are large molecules that shorten T1 relaxation time (leading to an increase in signal intensity on T1-weighted images). After intravenous injection, they initially transit through the heart and vessels. Then, contrast agents rapidly diffuse from intravascular to interstitial space, being unable to enter cells provided that the tissue cell membranes are intact. Finally, if wash-in and wash-out kinetics are normal, contrast concentration in the tissue will decrease in minutes.
• Several CMR techniques are based on the evaluation of the dynamics of paramagnetic contrast agents in the heart:
• First-pass contrast CMR visualizes the initial transit of contrast media in the heart and is useful to evaluate myocardial perfusion.
• Delayed contrast enhancement CMR (DE-CMR) detects myocardial retention of contrast, which might result from abnormal vascular permeability (i.e., myocarditis), passive diffusion into the intracellular space due to the loss of membrane integrity (i.e., myocardial infarction), and increase of the interstitial space due to accumulation of collagen or reduction of the capillary density (i.e., myocardial fibrosis and scars).
• CMR can be combined with pharmacological stress for the detection of ischemia and assessment of myocardial viability. The excellent imaging quality provides accurate depiction of the regional contractility, which can be assessed qualitatively or precisely quantified in terms of regional shortening and thickening.
• Tagging is a technique for quantitative analysis of myocardial contraction. Late diastolic selective saturation pre-pulses are used to superimpose a grid across the field of view. Myocardial tissue with initial saturated magnetization remains hypointense throughout the cardiac cycle. Subsequent tracking of tag line deformation through time allows direct assessment of myocardial strain and torsion.
• Recently available single-shot fast planar sequences and parallel imaging techniques significantly reduced the acquisition time for CMR imaging.
• CMR does not expose patients to ionizing radiation and does not require the injection of potentially nephrotoxic contrast medium. Therefore, it can be repeated as often as necessary for follow-up.
• Real time CMR imaging has been introduced recently, allowing the acquisition of the image in one cardiac cycle. However, it still faces problems related to lower spatial resolution.
CMR: Limitations and Disadvantages
• Need for breath-holding sequences (10–20 s each) during acquisition: because respiratory movement has a major impact on image quality.
• Long acquisition time (10 to 20 min): conventional CMR imaging techniques require 10 to 12 breath-hold periods to obtain the complete data set. That may lead to patient restlessness and inaccuracy caused by slice misregistration between breath-holds.
• CMR may be unviable for patients with congestive heart failure and high-grade dyspnea, who often cannot withstand the long examination time in the supine position and repeated breath-holding.
• Difficulties in case of tachycardia or unstable heart rhythm: the ECG is used to synchronize the data, and the final image is averaged from data acquired across several cardiac cycles. ECG gating may be difficult if the heart rhythm is not regular but real-time sequences are already available for these patients.
• Metallic implants such as prosthetic valves, coronary stents, sternal wires, and hip and knee prostheses may produce local image artifacts (but pose no hazard at field strengths of 1.5 and probably 3 T).
• CMR is contraindicated in patients with neurostimulators, cochlear implants, metal fragments retained in the eye, and certain vascular clips.
• Gadolinium is contraindicated in patients with advanced kidney disease due to the risk of nephrogenic systemic fibrosis.
Nuclear Cardiology Imaging Modalities
• Nuclear cardiology imaging modalities differ from other imaging techniques since instead of providing anatomic information they evaluate physiological functions of the heart.
• Depending on the tracer used, nuclear techniques may assess myocardial perfusion, metabolism, contractility, or neural innervation.
• The integrated biological risk-benefit balance should be taken into account whenever SPECT or PET are considered since exposure to high levels of radiation occurs.
Radionuclide Ventriculography
• Red blood cells are labeled with technetium-99m (99mTc) pertechnate and reinjected into the patient.
• Equilibrium radionuclide ventriculography is a technique in which planar images in anterior and left anterior oblique projections are acquired once the radiotracer has equilibrated within the blood pool.
Single-photon Emission Computed Tomography
• Nuclear imaging modality in which gamma rays emitted by radionuclides (tracers) are directly detected using a rotating gamma camera.
• 2D data are acquired from multiple projections to reconstruct a three-dimensional (3D) dataset. The information is typically presented as cross-sectional slices of the heart but can be freely reformatted as required.
• SPECT combined with a cardiac stress test is used to assess myocardial perfusion and viability. Thallium-201 (201Th)- or 99mTc-labeled sestamibi and tetrofosmin are the available tracers for that purpose.
• ECG triggering of SPECT acquisition allows the assessment of the tracer distribution throughout the cardiac cycle and provides additional quantitative information about wall motion, wall thickening, and LV volumes.
• Recent advances include phase analysis of gated SPECT, which enables the evaluation of LV regional mechanical activation and promises to be useful in the assessment of LV dyssynchrony.
SPECT: Limitations and Disadvantages
• Use of radioactive material: the effective dose of a single nuclear stress imaging ranges from 10 to 27 mSv, which is equivalent to exposure to 500 chest X-rays (sestamibi-SPECT) or 1200 chest X-rays (thallium-SPECT).
• Low temporal resolution: with ECG-gated SPECT, 16 frames at most are assessed by the cardiac cycle.
• Difficulty to distinguish between true perfusion defects and fixed defects caused by soft tissue attenuation, particularly in women (photoelectric absorption in the breast) and obese patients. Several methods of nonuniform attenuation correction are available, including the use of parametric maps obtained from low-dose CT. Wall motion analysis by ECG-gated SPECT also aids in the distinction.
Positron Emission Tomography
• Nuclear imaging technique that detects pairs of gamma rays emitted indirectly by positron-emitting radionuclides (tracers), which are introduced into the heart on biologically active molecules.
• The high-energy photons released from the annihilation of positrons provide more radiation-event localization information and thus confer spatial resolution superior to SPECT.
• The major clinical applications of PET are:
• Assessment of myocardial perfusion at rest and during stress, using as tracers nitrogen-13 (13N)-ammonia, rubidium-82 (82Rb), or oxygen-15 (15O)-water.
• Promising applications of PET imaging but still restricted to research purposes are:
• Evaluation of endothelial dysfunction, by detecting low coronary flow reserve in the absence of epicardial coronary disease. The assessment of endothelial dysfunction promises to improve the long-term risk stratification by identifying early asymptomatic coronary artery disease (CAD).
• Identification of vulnerable plaque: preliminary studies have suggested that iodine-125 (125I) labeling of low-density lipoproteins and 99mTc-annexine V, which localizes the apoptotic cells, may depict the unstable atheromatous plaque.
• Assessment of ischemic memory: after a transitory ischemic event, the perfusion is restored but the myocardial metabolism remains abnormal during some days. Instead of obtaining energy through fatty acid metabolism, myocardial cells use glucose. This phenomenon can be demonstrated with labeled fatty acid scintigraphy or 18F-FDG SPECT.
• Major advantages of PET are:
• Less affected by soft tissue attenuation than SPECT (leading to better accuracy for the assessment of obese individuals and women with large breasts).
• ECG gating may be applied to PET, providing additional information regarding ventricular function.
Hybrid Imaging
• Hybrid imaging combines the high-resolution 3D anatomic depiction of the coronary arteries by CT-angiography with the functional evaluation of myocardial perfusion by SPECT or PET.
• Integration of CTCA data with SPECT/PET information can be achieved with hybrid scanners (which combine SPECT or PET devices with high-end CT scanners) or with software fusion of datasets obtained from separate stand-alone scanners.
• Hybrid imaging promises to offer a full comprehensive noninvasive assessment of CAD, yielding complementary information both on coronary stenosis anatomy and hemodynamic significance of relevant lesions.
• The clinical impact on treatment strategy and patient outcome of hybrid imaging remains to be determined in large-scale prospective studies.
Hybrid Imaging: Limitations and Disadvantages
Key Points
• Echocardiography may need to be supplemented by other imaging modalities for accurate diagnosis in some patient subgroups.
• CCT offers high spatial resolution. Prospective ECG gating allows lower radiation exposure compared with conventional CT imaging. Dual-source multidetector scanners reduce motion artifact.
• CMR allows evaluation of cardiac structure and intracardiac flow, using data acquired with pulse sequences specific to each clinical application.
Assessment of Ventricular Anatomy, Systolic Function, and Mass
• Echocardiography is the first choice of imaging test in the assessment of ventricular anatomy, systolic function, and mass. However, echocardiography has three relevant limitations:
• If the acoustic window is insufficient to provide a high degree of diagnostic certainty, ventricular opacification with echocardiographic contrast agents may be useful, improving the endocardial visualization.
• Quantification of the ventricular volumes by 2DE depends on geometric assumptions, which do not apply to the RV (due to its unique geometry) and are often incorrect even for the LV (especially when the ventricular shape deviates from assumed geometric models, as in ischemic or dilated cardiomyopathy).
• Evaluation of the ventricular geometry and systolic function using three-dimensional echocardiography (3DE) uses direct volumetric quantification, not relying on geometric modeling and preventing errors caused by foreshortened views (Fig. 12-1).
• Specific software designed for quantification of LV volumes (disk summation) and for volumetric analysis of the RV is currently available for 3DE.
• 3DE improves the accuracy of the ventricular quantification and reduces the unacceptable high level of variability of the 2DE evaluation.
• Disadvantages of 3DE include worse image quality, lower spatial resolution (because of lower line density than 2DE), suboptimal temporal resolution, and time-consuming analysis. Hopefully, further advances in transducer and computer software technology will progressively solve these problems.
• 3DE may be combined with ventricular opacification with contrast to improve the accuracy of the quantitative analysis and to expand 3DE use in patients with suboptimal endocardial visualization.
Left Ventricular Anatomy, Systolic Function, and Mass
• The accurate and reproducible assessment of LV volumes, function, and mass is crucial for the determination of appropriate therapeutic procedures, monitoring disease progression, timing of surgery, and prognostic stratification in patients with cardiac disease.
• Echocardiography is the primary modality used in routine clinical practice. 2DE techniques allow reasonably accurate measurements of LV mass, volume, and ejection fraction (EF).
• LV quantification by echocardiography is based on geometric assumptions, assuming an idealized cardiac shape that may not represent the patient being studied.
• Error due to geometric modeling is particularly significant in the case of remodeled ventricles, as in patients with dilated cardiomyopathy, wall motion abnormalities, and ventricular aneurysms.
• Echocardiography is significantly operator and acoustic window dependent, which contributes to high inter- and intraoperator variability and low interstudy reproducibility.
When to Consider Additional Imaging
• The assessment of LV anatomy and systolic function is crucial in every cardiac imaging examination, but it is especially relevant in the evaluation of patients with heart failure symptoms.
• High-quality imaging is crucial to confirm the clinical diagnosis of heart failure and to assess deterioration or response to treatment during follow-up.
• Imaging evaluation aims to quantify chamber dimensions and global systolic function, detect wall motion abnormalities, search for LV hypertrophy and diastolic dysfunction, look for valvular dysfunction, and assess pulmonary hemodynamic parameters.
• If the echocardiographic image quality is good, with full visualization of endocardial and pericardial borders in all segments, standard 2DE may provide adequate evaluation of LV anatomy and accurate estimation of LV volumes, EF, and mass.
• 3DE improves the accuracy of the evaluation of LV volumes and mass by eliminating the need for geometric modeling. Thus, it may be particularly useful whenever an abnormal LV geometry is suspected, such as in patients with dilated cardiomyopathy, wall motion abnormalities, ventricular aneurysms, or abnormally shaped ventricles secondary to congenital heart disease (CHD).
• However, 3DE still requires adequate acoustic access and depends on the ability to include the entire LV cavity within the 3D pyramidal scan volume. That is frequently impossible to achieve in patients with markedly dilated LV cavities.
• If the acoustic window is insufficient to provide a high degree of diagnostic certainty in the assessment of heart failure patients, evaluation should proceed to another imaging modality, such as contrast echocardiography, CMR, CCT, or nuclear imaging.
• The most appropriate added imaging modality depends on the suspected cause for heart failure: ischemic or nonischemic myocardial dysfunction, valvular abnormality, or pericardial disease.
Cardiac Magnetic Resonance
• CMR is the most precise technique for quantification of LV geometry, volumes, EF, and mass, so it is considered the gold standard for noninvasive volume measurements of LV.
• CMR has the highest reproducibility and lowest interstudy variability, which is essential for longitudinal follow-up studies of patients and especially in research. The excellent interstudy reproducibility minimizes the sample size for studies of the effects of drugs on cardiac function or mass, reducing time and cost for clinical trials.
• LV (and RV) volume and mass assessment is optimally performed with multislice 2D SSFP cine imaging covering the entirety of the ventricular chamber (Fig. 12-2).
• From contiguous LV short axis slices, each one 5 to 10 mm thick, LV volumes and mass are determined by planimetry of the blood-endocardium border (end-diastole and end-systole) and epicardial border (end-diastole) for each slice and summed for the entire ventricle (Fig. 12-3).
• Semi-automated analysis of LV mass, volume, and systolic function with intensity-based thresholding and long axis valve plane tracking is currently available, saving time.
• Besides quantifying regional and global LV systolic function, CMR may provide functional hemodynamic information by the assessment of LV stroke volume and concomitant valvular disease.
• CMR may be particularly useful in the work-up of patients with heart failure, providing detailed visualization of all areas of the heart, accurate quantification of LV and RV volumes and function, superior tissue characterization, assessment of cardiac dyssynchrony, and comprehensive assessment of valvular and hemodynamic function.
• Tissue characterization by CMR may help in the distinction between ischemic cardiomyopathy, dilated cardiomyopathy, and less common diseases, such as sarcoidosis and myocarditis. For that purpose, delayed enhancement should complement standard CMR evaluation, directly visualizing myocardial inflammation and fibrosis:
• Ischemic pattern: characterized by the presence of myocardial scar, visualized as an area of delayed hyperenhancement invariably involving the subendocardial regions in the distribution territory of a coronary artery.
Cardiac Computed Tomography
• CCT using retrospective gating provides reasonably accurate assessment of LV geometry, volumes, EF, and mass. CCT is more accurate than echocardiography, nuclear imaging, or contrast ventriculography as long as a minimum temporal resolution is guaranteed.
• Performance for assessment of LV function is limited by the relatively modest temporal resolution of CCT.
• New prospective gating protocols that reduce radiation exposure do not provide information on LV function.
• The major advantage of CCT in the assessment of LV anatomy and systolic function is the shorter examination time than for CMR (<15 s), which makes it more feasible in patients with heart failure symptoms.
• The two major limitations of CCT are (1) exposure to ionizing radiation, which limits its usefulness and precludes its use in follow-up studies, and (2) use of contrast media, which may transiently change the ventricular performance due to preload and negative inotropic effect.
• Given the predominant disadvantages of contrast medium application and radiation exposure, the assessment of LV function and morphology alone is not an indication for CCT, as these parameters can be assessed by echocardiography and CMR without radiation exposure and with higher temporal resolution.
• CCT assessment of LV volumes and mass should be considered a part of an examination required for other purposes, such as evaluation of coronary anatomy. Thus, CCT may be of value for patients with heart failure and distorted ventricles to elucidate the etiology, because information in terms of LV volumes, function, and mass is complemented by data concerning coronary anatomy without additional imaging time or contrast.
Nuclear Cardiology
• Radionuclide ventriculography and ECG-gated nuclear imaging techniques allow calculations of the LV end-systolic and end-diastolic volumes and thus EF.
• Measurements do not rely on geometric assumptions, being derived from end-systolic and end-diastolic count densities.
• 3D tomographic techniques (PET and 99mTc-SPECT) provide better accuracy than radionuclide ventriculography (planar acquisitions).
• Errors may occur in the case of small or large hearts, including patients with ischemic dilated cardiomyopathy due to the difficulty in assigning ventricular borders to areas with low counts, such as scars or areas of infarction.
• Spatial and temporal resolution of nuclear imaging modalities is insufficient to assess LV mass or geometry.
Right Ventricular Anatomy and Systolic Function
• Assessment of RV geometry and function using cardiovascular imaging techniques is considerably more challenging than the evaluation of LV function.
• These challenges are attributed to the complex geometry of the RV, which makes geometric modulation unviable.
• The RV is also difficult to visualize by echocardiography because of its crescentric shape around the LV and proximity to the sternum.
• Several 2DE approaches for quantification of RV volumes have been proposed, but none has shown to be reliable because:
• Geometric models used for calculations are inappropriate, due to the complex shape of the chamber, which is irregular, asymmetric, crescentric, and truncated.
• The RV has a unique geometry that makes it difficult to obtain orthogonal long axis views rotated around a common axis (required for biplane Simpson’s and area-length techniques).
• The complex geometry of the RV makes it ideally suited for 3D analytical models. Progressive development of 3DE has made it possible to evaluate the RV in the clinical setting and promises to overcome some limitations of 2DE.
• For RV quantification by 3DE, it has been shown that the disk summation technique offers no significant advantage in comparison with 2D methods. Conversely, recently developed software specific for volumetric analysis of the RV from 3DE datasets without using geometric assumptions does improve accuracy.
• Echocardiographic contrast agents and intravenous saline contrast can facilitate RV endocardial border definition, improving the accuracy and reproducibility of echocardiographic structure and function.
• However, 3DE strongly depends on adequate acoustic windows and relies heavily on the scanning abilities of the sonographer.
• Methods for quantitatively assessing RV function with 3DE have not been widely applied in clinical practice, and the expertise in their use is currently limited to a few centers.
• Despite extensive research and promising results, quantification by 3DE cannot be recommended as a standard for routine clinical practice.
When to Consider Additional Imaging
• Careful and comprehensive evaluation of RV systolic function is indispensable in multiple clinical settings, especially in patients with suspected cardiomyopathy, arrhythmogenic RV dysplasia, inferior wall myocardial infarction, valvular heart disease, pulmonary hypertension, and CHD.
• Patients with pulmonary hypertension require accurate serial evaluation of RV function to assess the progression of the disease and to determine the effects of treatment. In fact, the severity of pulmonary hypertension as determined by the pulmonary artery pressure does not reliably predict survival, whereas indices of RV performance are strong predictors of outcome.
• Patients with repaired tetralogy of Fallot, who commonly have a significant degree of pulmonary regurgitation, and individuals who have undergone valvuloplasty for congenital pulmonary stenosis have a high risk for long-term RV dysfunction, which negatively impacts their prognosis. Longitudinal assessment of RV function can guide the timing of reintervention.
• Since RV volume measurements are not interchangeable between imaging modalities, serial evaluations should preferably be performed using the same modality.
• In most clinical settings, the assessment of RV systolic function is performed qualitatively with 2DE techniques and relies on the interpretative ability of the operator.
Cardiac Magnetic Resonance
• CMR is considered the reference method to assess anatomy, geometry, as well as regional and global RV systolic function (Figs. 12-4 and 12-5).
• Even CMR may face problems since it provides suboptimal discrimination of the endocardial boundary, due to the presence of heavy trabeculation.
• Among the available imaging modalities, the volumetric analysis of CMR images yields the most accurate and reproducible measurements.
• So, 2DE is the first-choice technique when screening for RV dilation or dysfunction. In most patients, echocardiography provides sufficient information to complete the diagnostic evaluation. However, if accurate serial evaluation of the RV volumes is required (namely in patients with RV compromise due to CHD or pulmonary hypertension) or exhaustive tissue characterization of the RV myocardium is pretended (namely in patients with clinical suspicion of arrhythmogenic RV cardiomyopathy), CMR should be the preferred imaging modality.
Cardiac Computed Tomography
• MSCT provides the best spatial resolution to differentiate RV trabeculae from the myocardium and blood pool, allowing the best discrimination of the inward displacement of the endocardial boundary and the most reproducible assessment of RV parameters, as long as a minimum frame rate is guaranteed.
• CCT has a relatively low temporal resolution compared with CMR and echocardiography, a limited number of phases of the cardiac cycle being analyzed. Even so, retrospective gated CT has been shown to be accurate in the quantification of RV volumes, mass, and EF in adults.
• Because of its use of ionizing radiation, CCT may cause unwanted biological effects, particularly in the case of children or repeated examinations in adults.
• The large volume of contrast medium administered at a high flow rate during acquisition may negatively affect the RV volumetric parameters due to preload and negative inotropic effect.
• The accuracy of MSCT in assessing RV anatomy and EF in patients with depressed RV contractility, CHD, or valvular dysfunction needs to be further determined in large scale studies.
Cardiac Nuclear Imaging
• Nuclear imaging techniques are able to accurately and reproducibly derive RV EF from radiotracer count densities.
• Because global RV systolic function is derived from end-systolic and end-diastolic count densities, it is independent of the geometric assumptions.
• Nuclear techniques that have been used to assess RV function include first-pass radionuclide angiography, equilibrium radionuclide angiography, and SPECT equilibrium radionuclide angiography.
• First-pass radionuclide ventriculography is the preferred nuclear imaging approach to calculate RV EF. It involves the injection of a bolus of radionuclide tracer into a peripheral vein followed by imaging with a gamma camera with high count rate capabilities. Data are summed over a span of several cardiac cycles, and RV EF is derived from end-systolic and end-diastolic frames.
• Its success is largely dependent on the quality of the injected bolus, and its accuracy may be affected in patients with severe RV dysfunction or significant tricuspid regurgitation.
• SPECT equilibrium ventriculography is accomplished by blood pool labeling with 99mTc followed by tomographic ECG-gated data acquisition. Because of its 3D nature, it enables precise identification and isolation of RV counts, allowing accurate quantification of RV volumes.
Myocardial Tissue Characterization
• CMR is the better established imaging modality for tissue characterization, assessing tissue properties in T1- and T2-weighted sequences as well as detecting delayed hyperenhancement.
• Delayed contrast enhancement of the myocardium can also be assessed by CCT, but it has not been fully validated and requires exposition to substantial ionizing radiation.
• Late enhancement is analyzed in inversion recovery images acquired 5 to 20 min after the intravenous injection of paramagnetic contrast agent.
• The presence of delayed hyperenhancement reflects abnormal wash-in/wash-out dynamics of the contrast within myocardial tissue, which may be caused by:
• Abnormal vascular permeability, as in acute myocarditis or the acute phase of cardiac sarcoidosis.
• Passive diffusion of contrast into the intracellular space due to the loss of membrane integrity, as within necrotic areas of acute myocardial infarction. Some acute infarcts have a central dark zone in the area of hyperenhancement, representing microvascular obstruction.
• The pattern of distribution of delayed hyperenhancement provides relevant clues regarding the etiology of LV dysfunction since fibrosis due to CAD invariably involves the subendocardial areas.
• Thus, tissue characterization may direct or confirm the diagnosis of cardiac sarcoidosis, cardiac amyloidosis, siderotic cardiomyopathy, arrhythmogenic right ventricular cardiomyopathy, and myocarditis (Fig. 12-6).
Arrhythmogenic Right Ventricular Cardiomyopathy
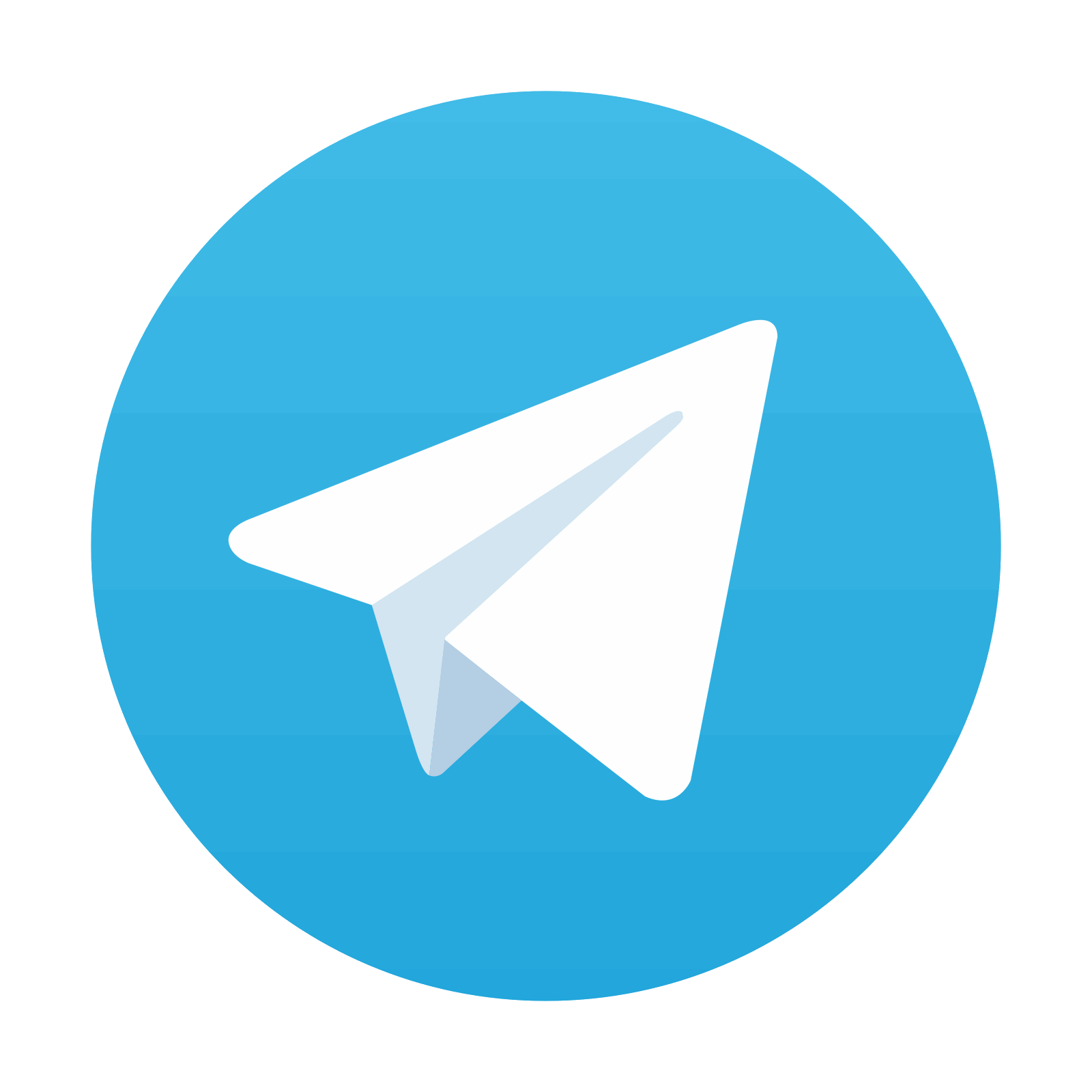
Stay updated, free articles. Join our Telegram channel

Full access? Get Clinical Tree
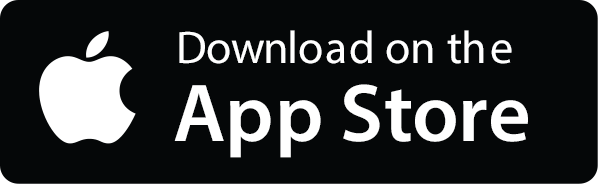
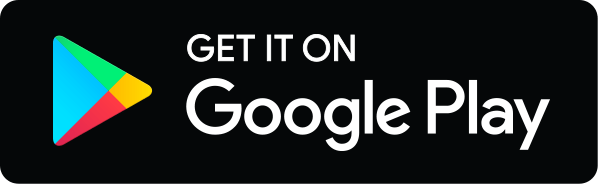