Multifunction Cardiogram, a.k.a. MCG
Raffi B. Shen, BA
Norbert W. Rainford, MD, FACC, FACP
Joseph T. Shen, MD
Introduction
Coronary artery heart disease (CAD) is a major cause of death and disability in developed countries. Although CAD mortality rates worldwide have decreased over the past four decades, CAD remains the cause of about one-third of all deaths among individuals over age 35 years.1,2,3 About half of all middle-aged men and one-third of middle-aged women in the United States will develop some manifestation of CAD.4 There are several unrecognized drivers or causes of the persistence of this morbidity despite our general knowledge of major risk factors based on population data such as the Framingham Heart Study. The lack of adaptable, inexpensive, noninvasive, and accurate modalities to detect CAD in its early stages as well as the lack of effective monitoring of the effects of diet and other lifestyle intervention has been a major factor.
Electrocardiogram (ECG) stress testing, nuclear scintigraphy, stress echocardiography, and other various types of cardiac stress imaging testing are considered the standard noninvasive techniques for evaluating cardiac ischemia. Although these are recognized as sensitive tests for the detection of CAD in two or more large epicardial vessels, it also has been widely acknowledged that they have poor specificity as shown by evidence of a high number of false-positive results. There is growing consensus that this lack of specificity results in a significant number of unnecessary coronary angiographies, thereby subjecting many patients to the potential risks involved with invasive procedures and radiation exposure without expected commensurate clinical benefit. For example, in 2010, Patel and colleagues published an analysis of the American College of Cardiology National Cardiovascular Data Registry, which included 397,954 patients without known CAD who were undergoing elective angiography.5 At catheterization, 149,739 patients (37.6%) had obstructive coronary artery disease (70% obstruction or greater), requiring an interventional procedure. Stated bluntly, up to 62.4% of those patients could have avoided coronary angiography if more accurate noninvasive testing modalities were available. Compounding the diagnostic inadequacy of conventional testing of obstructive CAD is the emerging consensus of the role of nonobstructive coronary disease and microvascular disease in the clinical manifestation of ischemic heart disease. A review in Circulation 1995 by Erlin Falk demonstrated that the progression to plaque rupture and myocardial infarction (MI) over time occurs most frequently in patients with obstruction of 50% or less.6
It is within this context of complex and evolving concepts that Premier Heart is proud to introduce the Multifunction Cardiogram (MCG), a noninvasive, physical-stress-free, and nonionizing diagnostic tool that can be used to quantitatively assess lesions across the very early nonobstructive to significantly obstructive spectrum and to monitor any form of therapeutic intervention.
The Purpose of This Chapter
This chapter provides an opportunity for the health professional to learn about a “new” diagnostic tool that has been more than 20 years in the making. It provides an outline as to why conventional cardiac testing is inadequate and why MCG is the ideal tool to fulfill this unmet need. There are several paradoxical or unexpected clinical developments in the management of CAD over the last several years. Although it is generally accepted that low-density lipoprotein (LDL) cholesterol plays a central role in the initiation of the coronary plaque, it is known that 50% of patients presenting with a MI have normal total cholesterol.7 Additionally, the data from several primary and secondary prevention trials have shown that the majority of the risk of CAD still remains even after LDL reduction.8 Perhaps the most glaring event of the inadequacy of conventional approach to the management of CAD is the 15-year outcome status report of the COURAGE trial (Clinical Outcomes Utilizing Revascularization and Aggressive Drug Evaluation).9 The 15-year status of this study essentially demonstrated that
percutaneous coronary intervention (stent placement) in patients with stable CAD did not improve in mortality outcome when compared with similar patients who were treated using only optimal medical management. Perhaps related is an older but interesting piece of data from the FAME (Fractional Flow Reserve versus Angiography for Multivessel Evaluation) study.10 The FAME study looked at 1-year outcomes (death, MI, major adverse cardiovascular event) in one group of patients whose management was guided by angiographic results versus a similar group guided by the fractional flow reserve (FFR) data. The FFR-guided group had significantly better outcome in all categories. A systems approach to address the functional aspects of this complex biological conundrum, known as the cardiovascular system, is desperately required.
percutaneous coronary intervention (stent placement) in patients with stable CAD did not improve in mortality outcome when compared with similar patients who were treated using only optimal medical management. Perhaps related is an older but interesting piece of data from the FAME (Fractional Flow Reserve versus Angiography for Multivessel Evaluation) study.10 The FAME study looked at 1-year outcomes (death, MI, major adverse cardiovascular event) in one group of patients whose management was guided by angiographic results versus a similar group guided by the fractional flow reserve (FFR) data. The FFR-guided group had significantly better outcome in all categories. A systems approach to address the functional aspects of this complex biological conundrum, known as the cardiovascular system, is desperately required.
What Is MCG and What Does It Measure?
The MCG is the first embodiment of a mathematical application of systems theory to a dynamic biological (cardiovascular) environment, which expresses the physiologic state of the heart, with a primary focus on the level of its ischemic burden. In other words, the MCG describes the dynamic functional sate of the heart, beyond relying upon anatomical status. Premier Heart’s greatest priority was always the measurement of the ischemic burden, but there were additional markers that needed to be recruited to describe the heart within a functional perspective. That expression was (is) the mathematical articulation of the communication between two standard ECG leads over multiple cycles, beginning with the conversion of the signals into a frequency domain via multiple nonlinear mathematical functions, thus the use of the term “multifunction cardiography.” The adaptation of systems analysis principles, combined with Lagrangian mechanics, empirical data mining, deep machine learning, and neural network development, created, to our knowledge, the first example of a commercially available information technology solution in the discipline of “Clinical Computational Electrophysiology.” Following decades of research and development, through the diligent work of two generations of dedicated scientists, clinicians, and engineers, MCG technology has evolved from conceptual mathematical designs to animal testing and finally to human application. Although x-ray, computed tomography,11 magnetic resonance (MR), or ultrasound technologies describe spatial anatomic separation, MCG describes frequency separation, whereby specific mathematical elements from the multiple functions in frequency domains via power spectra are ascribed to specific anatomical or physiologic functions of the whole cardiovascular system.12
It is beyond the scope of this chapter to delve into full explanation of the applied Lagrangian mechanics used by this technology, but it is helpful to outline a few basic principles. Blood flow is a non-Newtonian fluid that is optimally assessed and reported with Euler coordinates. Cardiac tissue and brain tissue are viscoelastic solids, and they are assessed and reported with Lagrange coordinates. Among the infinitely possible mathematical expressions within this system, we have empirically selected six dynamic and integrative mathematical functions that act as the backbone of our mathematical analysis, namely, auto power spectrum, phase angle shift, impulse response, cross correlation, coherence function, and transfer function. The Euler and Lagrange coordinates are then linked by a Laplace transformation application (see Figure 12.1).
The deeper questions as to what these frequency interrogations represent are issues such as:
Quantification of the abnormal electromechanical expression patterns of stress (physical) and strain and intracardiac blood flow.
Integration of all myocardial electrical power required to function under normal and abnormal conditions delivered by sodium (Na+), hydrogen (H+), potassium (K+), calcium (Ca2+), and magnesium (Mg2+) channels and ATPase activities of all myocardial cells through multiple cardiac cycles.
Oxidative stress caused by supply and demand imbalances, free radical formation, and lactic acidosis leading to ion channelopathies, particularly gradient dependent H+ channel (a subunit of F1-F0 ATPase) in addition to chronic damage/mutation of the mitochondrial gene transcription/translation mechanics, resulting in gradual myocardial power production.
All of this information and analyses are collected and collated by a bedside device within 10 minutes on the average patient, with the patient lying down (or sitting) quietly and remaining still for a few minutes. The device is the size of standard laptop. Electrocardiographic signals from two leads (II and V5) are recorded for 82 seconds per cycle and repeated for three to five times per session. Data are then uploaded and compared with the data patterns of hundreds of thousands of patients who have had the MCG test and who have had cardiac catheterization with angiogram. The database is equally male/female to eliminate gender bias, with an age range of 14 to 100 years. Every patient who has an MCG test in effect undergoes a virtual cardiac catheterization with an FFR assessment. How was this database built? The database is the end product of a laborious creation that is central to understanding MCG technology and may well be the most uniquely constructed database in all of medical data. The team, led by Dr Shen, embarked on a two-decade-long journey of research and development via digital signal processing, empirical clinical data collection, data mining, supervised machine learning, neural network development, artificial intelligence algorithm development, and countless iterations of optimization and improvement to create computer recognition of all forms of heart disease. In this endeavor, 2 million individuals were tested on MCG and more than 100,000 people with various heart diseases had their angiographic data strategically added to the system to build a production database for system software development. All the data sets used in analysis and the proposed statistical models had to satisfy the tests of
both null hypothesis and alternative hypothesis. The extremely carefully verified and thoroughly validated data sets were used in the discovery of over 200 mathematical elements from the six nonlinear functions. We ensured that the development of the machine learning algorithms for the quantitative automatic heart disease pattern classification and differentiation were based purely on thoroughly vetted and trusted empirical evidence. Based on well-defined frequency and separation, the aim was to systematically explore, define, express, measure, quantify, and differentiate the hidden NORMAL and ABNORMAL electromechanical, electrostructural, electrobiochemical, electrohematologic, electroneuroendocrine, neurohormonal, and electroimmunological expressions, as well as the highly elusive, yet vitally important diverse expressions of electromyocardial perfusion pattern of the cardiovascular system. It is this kind of unique analysis, coupled with the 24/7 accessibility and reliability, that positions MCG well beyond more expensive and more cumbersome imaging and perfusion techniques.
both null hypothesis and alternative hypothesis. The extremely carefully verified and thoroughly validated data sets were used in the discovery of over 200 mathematical elements from the six nonlinear functions. We ensured that the development of the machine learning algorithms for the quantitative automatic heart disease pattern classification and differentiation were based purely on thoroughly vetted and trusted empirical evidence. Based on well-defined frequency and separation, the aim was to systematically explore, define, express, measure, quantify, and differentiate the hidden NORMAL and ABNORMAL electromechanical, electrostructural, electrobiochemical, electrohematologic, electroneuroendocrine, neurohormonal, and electroimmunological expressions, as well as the highly elusive, yet vitally important diverse expressions of electromyocardial perfusion pattern of the cardiovascular system. It is this kind of unique analysis, coupled with the 24/7 accessibility and reliability, that positions MCG well beyond more expensive and more cumbersome imaging and perfusion techniques.
![]() Figure 12.1 Graphic representation of the linkages between the Euler coordinate and Lagrange coordinate. |
The final report gives an overall functional severity score between 0 (totally normal) and 22 (the most severe) (see Table 12.1). The report also gives an assessment of secondary pathological and physiopathological conditions. These secondary conditions or markers include the following: cardiomyopathy (derangements in myocardial wall motion and/or structure), hypertrophy, arrhythmias or precipitating substrates, rheumatic pattern (LV, aortic/mitral valve derangements), pulmonary pattern (right heart, pulmonic/tricuspid valve derangements), myocardial damage (MI, contusion, etc.), congenital abnormalities, compliance changes, remodeling, energy output, ejection fraction, and angle phase shift. Although there is a generally good correlation between the severity score and the level of obstruction, there is no specific correlation between the numerical severity score and the percentage of blockage. This fact underscores the contribution of the secondary factors to the functional assessment of the heart and highlights the difference between conventional anatomical imaging and MCG’s functional physiological assessment. It also underscores the importance of the FFR information alluded to earlier in the FAME study.
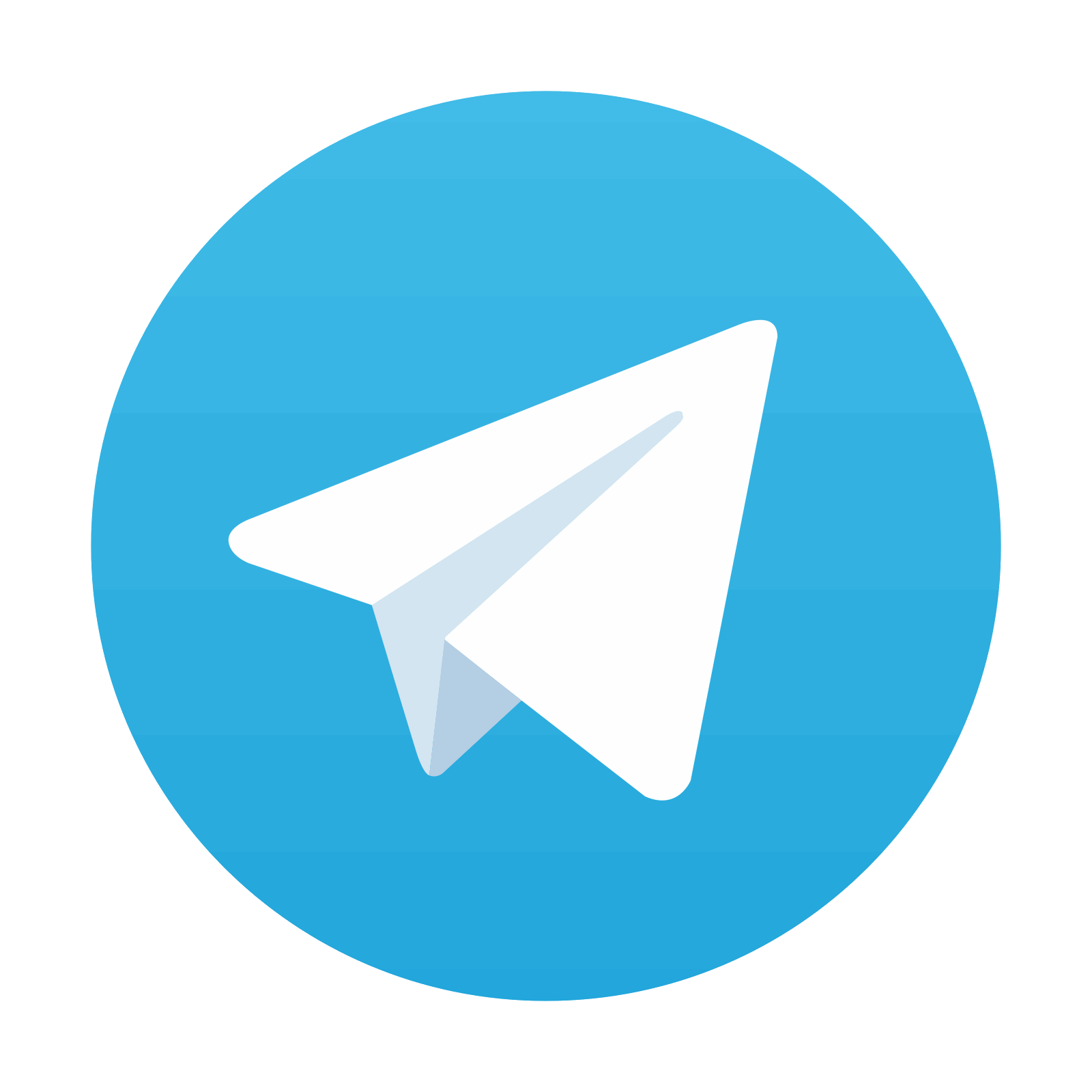
Stay updated, free articles. Join our Telegram channel

Full access? Get Clinical Tree
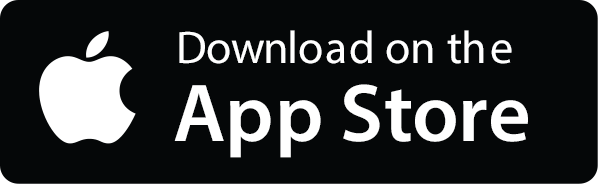
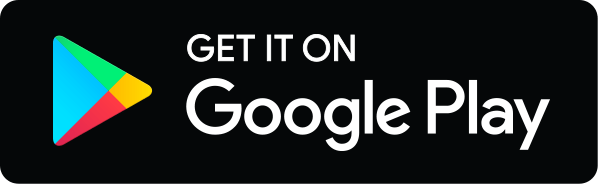