(18.1)
where VT = tidal volume, V (IL) corresponds to the flow or volume exiting through the leak port, V (NIL) is the flow/volume of unintentional leaks, and CV is the compressible volume.
In the laboratory environment, and with the help of a simulator, the morphology of the native graphics can be reproduced, placing a pneumotachograph at the exit of the ventilator. For example, in a system with single limb and leak port, and without unintentional leaks, the morphology can be similar to that represented in Fig. 18.1.


Fig. 18.1
Reproduction of the correction for the intentional estimated leaks in native flow-time graphics (see text for more details) in the laboratory with the help of a simulator. The abrupt “drop” in the uncorrected volume-time graphic (Channel 3) is related to the reset in the integral of the flow-time graphic (in the transition negative-positive of the flow-time graphic – Channel 2-). Usually, built-in software displaying volume-time graphics can present this drop in the presence of leaks
In fact, the native flow-time graphic contains, in the inspiratory phase (Fig. 18.1, Channel 2) the amount of gas delivered to the patient plus the gas escaping through the valve (calculated from the pressure-leak equation in Channel 4). When the estimated leaks are subtracted from native flow graphs, the typical morphology with equal positive and negative phases is obtained (Channel 5). Conversely, the expiratory phase in the native flow-time graph is smaller than the true exhaled tidal volume because there is an amount of gas escaping through the leak port and not directly monitored by the pneumotachograph inside the ventilator.
This is only an example of how distorted the flow signal monitored inside the ventilator can be. The function of mathematical algorithms applied to this signal should be to provide true information about the gas entering the patient, and the algorithms should take into account some physical phenomena that may occur during noninvasive home ventilation in the clinical practice.
18.2.2 Physical Phenomena
18.2.2.1 The Expiratory System
Today, due to its simplicity, not only for the patient but also for caregivers, the single limb with an expiratory leak port embedded in the mask or as an independent piece between the mask and the tubing is probably the preferred configuration in home noninvasive ventilation. This expiratory leak port acts as a continuous leak, with a higher resistance than the tubing itself. The behavior of this continuous leak can be easily determined in the laboratory by means of the so-called “leak test”: with the distal end of the mask or tubing occluded, the system is submitted to increasing pressure levels (e.g., in continuous positive airway pressure (CPAP) mode) and a pneumotachograph inside the ventilator captures only the flow leaks. Finally, the data are plotted on an X-Y graph and a second-degree equation can approximate with reasonable accuracy the amount of intentional leak for each level of pressure. These values can be subtracted from the native graphics to obtain the true gas flow entering and exiting from the patient.
This approach has two drawbacks, however. First, it is not always easy to perform a leak test when the port is built into the mask because the seal of the distal end might be incomplete. The second drawback appears in the presence of leaks. In this setting, and explained by Poiseuille’s law regarding the flow of fluids, with a higher flow exiting from the ventilator, the pressure difference between the true monitoring point (inside the ventilator) and the expiratory leak port also increases. This phenomenon can lead to an overestimation of leaks and progressive underestimation of tidal volume at increasing leak values [3]. The latter phenomenon, however, can be overcome by the introduction of a correction factor based on the resistance of the tubing and following Poiseuille’s law. This factor attempts to calculate the distal (in the leak port) pressure as a function of exiting flow, resistance, and proximal pressure values.
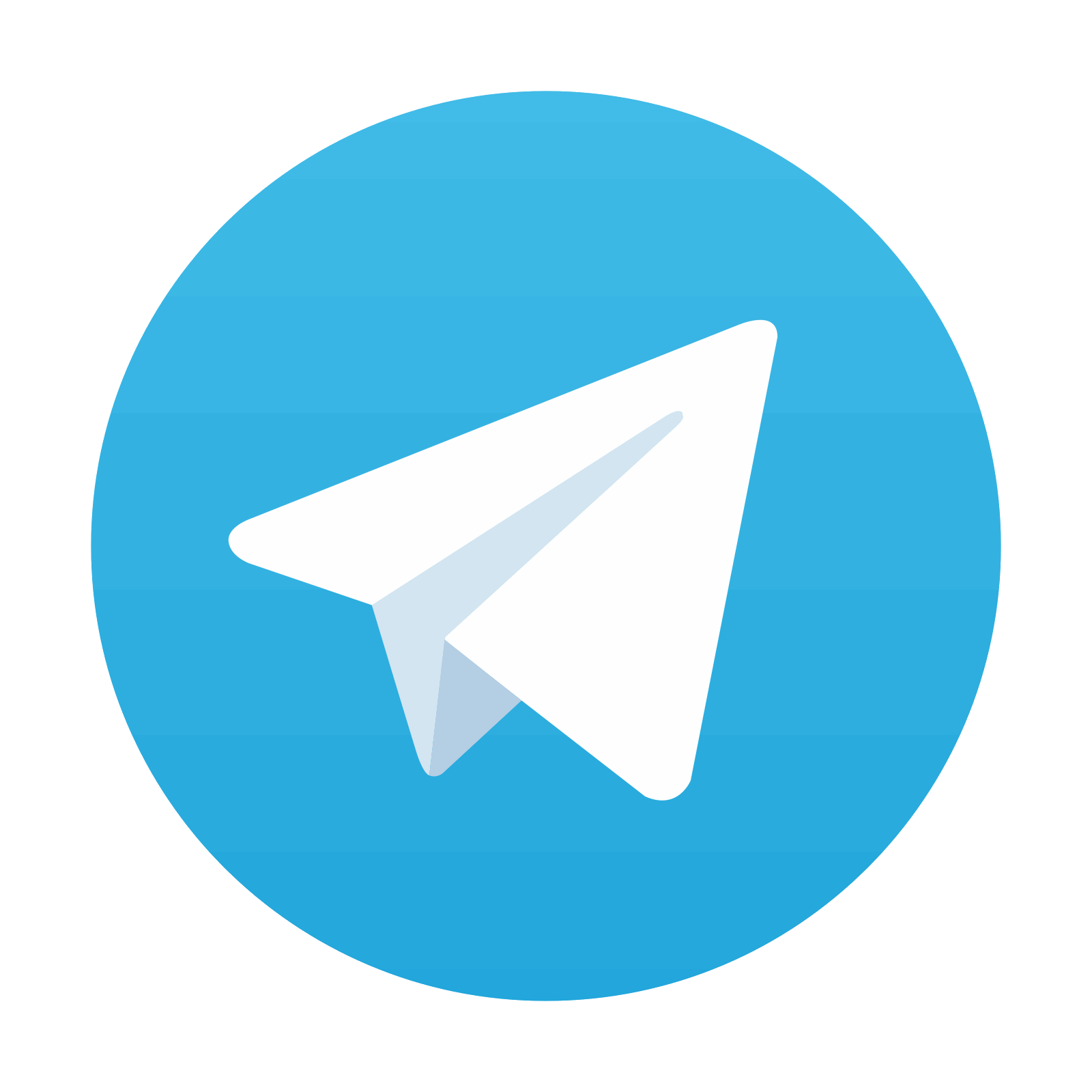
Stay updated, free articles. Join our Telegram channel

Full access? Get Clinical Tree
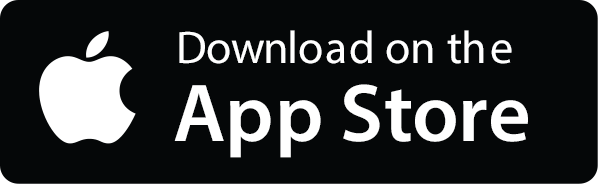
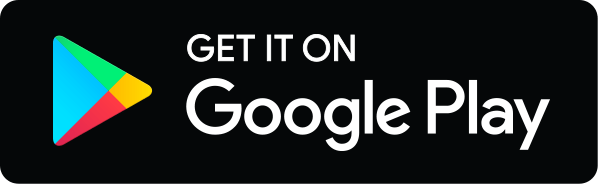