CHAPTER 2 Cells are the living inhabitants of our organs and tissues. The layout of our organs, tissues, and their respective inhabitant cells shows the cumulative processes of patterning during development, growth, and aging, as well as the stochastic processes of proliferation and cell death throughout life. In this context, the arrangement of cells in space and in time reflects their life history. In addition, the spatiotemporal arrangement of cells provides an indicator of current and future health of our tissues and organs at any given point in time. As a fundamental tenet of surgery, cell viability depends upon the presence of patent vascular networks in cells’ respective tissues and organs. Also, cells themselves provide intra- and peri-cellular communication networks across and between tissue compartments. The capacity for our tissues and organs to adapt and heal requires efficient communication between and through these cellular networks. Yet crosstalk between these networks is poorly understood. As the literally hardest tissue in the body, bone serves as the most technically challenging, exemplary model system to address this complex theme. Bone exhibits multiscale communication networks among osteocytes based upon the movement of pericellular fluid, ions, and nutrients through canaliculi and functional matrix pores that may serve regulatory functions to intracellular communication. In these networks, bone exhibits a molecular sieving function, effectively using different pathways to move fluid and solutes of various sizes and charge through the tissue. In a real sense, this represents an extravascular microcirculation of pericellular fluid in bone. Other tissues in and around joints participate in this fluid-based communication network. At organ, tissue, cell, and molecular length scales, bone provides more surface area for transport of fluid and exchange and filtering of solutes, to and from the vascular and lymphatic systems, than any other organ in the body. Furthermore, barrier function is intrinsic to the maintenance of distinct, yet juxtaposed physiological environments at surfaces separating the interior and exterior milieuof bone. Addressing novel, paired computational and experimental approaches to link fluid and solute flux between different length scales in bone underscores the key role of vascular and extravascular flow in bone cells’ dual role as tissue inhabitants and prototypers. After first exemplifying experimental and computational approaches to understand molecular transport in bone, analogous approaches are then applied to the more complex, higher length scale tissue system of the knee joint. Cutting edge imaging and modeling methods are used to understand the interplay of exercise and molecular transport in the various compartments of the knee joint. These insights are finally taken to the highest length scale to understand how exercise may modulate molecular transport and communication within the organism. Cells not only generate the extracellular matrix (ECM) comprising tissues but also are the living inhabitants of the tissues that make up the organs of the human body. In short, our cells are the living component of our physical structure. Their health state is reflected in the health status of our tissues and joints and vice versa.20, 22, 23 “Cells are [also] natural tissue builders,”22 giving our tissues and organs the capacity to heal. The integration of cell activity enables not only healing, but also tissue remodeling, and adaptation to changes in function such as mechanical loading or disuse. Although the cellular mechanisms underlying processes of healing and tissue remodeling are complex and not yet fully understood, the integration of cell activity depends on patent cellular machinery and thus cellular health. The integration also depends on cellular communication enabling navigation and coordinated activity, which in turn depend on tissue and organism health. The pericellular network provides an immediate conduit for transfer of information between cells and their matrix, as well as the vascular, lymphatic, and immune systems, which serve as bridges for further crosstalk between the tissues and organs comprising the integrated organism.14, 17, 19–25 Cellular connectivity derives from prenatal developmental processes where “everything is connected from day one.” Through pruning and honing of these connections, as well as through establishment and remodeling of cell-cell and cell-matrix adhesions, transport of information between cells, tissues, and organs, the musculoskeletal system takes on a higher level architecture, reflective of the boundaries of specific tissue types including muscle, bone, cartilage, meniscus, ligament, tendon, etc., all interacting with the vascular and lymphatic systems which provide major transport pathways for molecular communication.22 In each respective tissue of the musculoskeletal system, the pericellular fluid provides a medium through which environmental information, including mechanical forces, chemical gradients, and electrical impulses, are transmitted between and around cells, resulting in up- or down-regulation of gene expression.14, 17, 19–25 Delineated by the structural network of cells, which in themselves allow for direct cell-to-cell signal transmission across tissues via intracellular signaling,19 the contiguous pericellular fluid provides a powerful extracellular means to transfer information and modulate cellular activity. There is great impetus to elucidate the fluid environment of cells, as this knowledge could provide key insights into the mechanisms of cellular navigation and coordination during tissue remodeling and healing. At the same time, the pericellular fluid environment abuts and is thus interdependent on the state of the solid mechanical elements of tissues, as mechanical loading of tissue causes pericellular fluid to move, much like the fluid moves when a saturated sponge is squeezed.5, 17, 26 This interplay is particularly germane to the fluid environment and the structural elements of the respective tissues of the musculoskeletal system. Permeability through the micro- and nanoscale pericellular fluid network provides a quantitative measure of the tissue’s capacity for information transfer, e.g., transport of signals and molecules via the interstitial fluid network.16, 23, 31, 38 Experimental measurements as well as computational predictions of tissue permeability are challenging due to the remote location of cells and their networks. Nonetheless, the state of the ECM, and in particular the extracellular or pericellular space, is recognized as a common means by which different cells from tissues as diverse as brain,35 cartilage,1, 32, 34 meniscus,40 lymphatic tissue,46 and bone3 modulate and perceive their local environment. Permeability can be predicted computationally using virtual models of cellular networks. This approach is particularly helpful to predict cellular transport efficiency across tissues and organs. Alternatively, recently developed novel rapid prototyping methods allow for measurement of permeability based on application of classical fluid dynamics to rapid prototyped physical models of pericellular networks.23 Using state-of-the-art confocal microscopy, it is possible to get precise three-dimensional (3D), anatomical renderings of the nano-micro scale pericellular fluid space. These data sets are then input into rapid prototyping machines, like 3D printers, to create scaled-up (by 1000x) physical models of the respective cellular networks. According to classical fluid dynamics, measurements made on these scaled-up systems yield accurate measurements of the actual microscopic system if the fluid viscosity is scaled up analogously when carrying out the experimental measures. In this way, unprecedented experimental permeability measures can be made on physical models of actual pericellular networks. Bone tissue exhibits molecular sieving characteristics similar to the glomerulus of the kidney, where different calibers of porosity within bone, including macroscopic, vascular, pericellular, and intrinsic matrix porosity, allow for size- and charge-dependent penetration.43 The spatial distribution and density of blood vessels and different caliber porosities, as well as intrinsic matrix properties of bone tissue, modulate interstitial fluid flow direction and magnitude and thus spatiotemporal transport properties within the organ bone.6, 41, 43 Using in vivo fluorescent probes of specific size and charge, the molecular sieving characteristics of bone tissue have been characterized in unloaded and mechanically loaded states. Molecules larger than approximately 67 kDa, comparable to albumin, the most prevalent carrier protein in the blood, are not transported efficiently in unloaded bone. Furthermore, application of controlled mechanical loads using a mechanical testing machine to mimic loads incurred during physiological activities, augments molecular transport through bone via load-induced fluid displacement. Specifically, using a mechanical tester to compress the forelimb of the anaesthetized rat, a fluorescent tracer is shifted from the intramedullary canal to the outside surface of the bone, with a preponderance of tracer in the periosteum after loading.43 These studies demonstrate the effect of load-induced fluid displacements in bone from areas of higher to areas of lower pressure, much like squeezing of a saturated sponge. However, unlike a sponge that has rather permeable outside surfaces, periosteum bounds all non-articular bone surfaces.6
MOLECULAR TRANSPORT IN
MUSCULOSKELETAL HEALTH AND DISEASE
2.1 Introduction
2.2 Cells’ Dual Role as Tissue Inhabitants and Prototypers
2.3 Between Cells, Across Tissues and Organs: Molecular Transport is Central to Musculoskeletal Health and Disease
2.4 Approaching Molecular Transport in Musculoskeletal Health and Disease: Bone as a Case Study
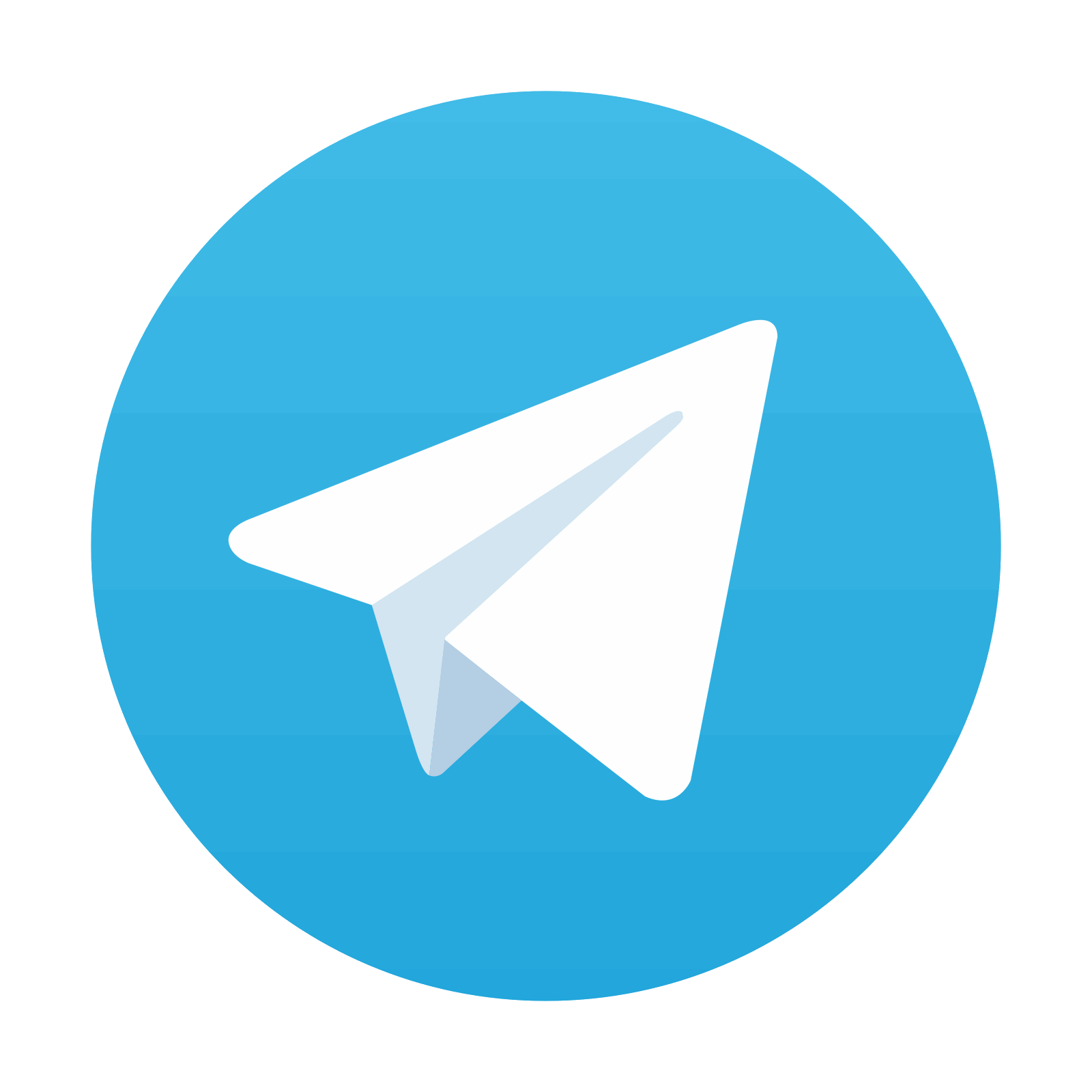
Stay updated, free articles. Join our Telegram channel

Full access? Get Clinical Tree
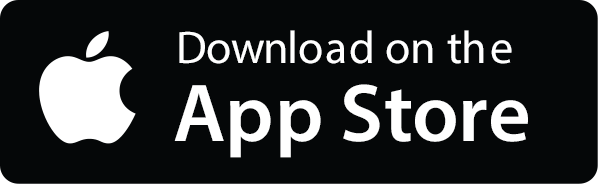
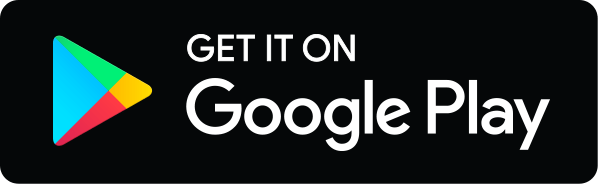