© Springer-Verlag Wien 2016
Silke Rickert-Sperling, Robert G. Kelly and David J. Driscoll (eds.)Congenital Heart Diseases: The Broken Heart10.1007/978-3-7091-1883-2_2727. Molecular Pathways and Animal Models of Atrioventricular Septal Defect
(1)
Department of Regenerative Medicine and Cell Biology, Medical University of South Carolina, Charleston, SC, USA
Abstract
The development of a properly functioning 4-chambered heart relies on the correct formation of the septal structures that separate the atrial and ventricular chambers. Perturbation of this septation process results in a spectrum of cardiac malformations involving the atrial and ventricular septal structures. Atrioventricular septal defects (AVSDs) form a class of congenital heart defects that are characterized by the presence of a primary atrial septal defect, a common atrioventricular junction, and frequently also a ventricular septal defect. While AVSD were historically considered to result from failure of the endocardial atrioventricular cushions to properly develop and fuse, more recent studies have determined that inhibition of the development of the dorsal mesenchymal protrusion (DMP), a derivative of the second heart field, can lead to AVSDs as well. In this chapter, we review what is currently known about the molecular mechanisms and pathways that are involved in DMP development and the pathogenesis of AVSD.
Keywords
Atrioventricular septal defectAVSDMesenchymal protrusionDMPOstium primum defectInlet-type ventricular septal defectPosterior second heart fieldSmith-Lemli-Opitz syndromeEllis-van Creveld syndromeNoonan syndromeHeterotaxy syndromeAV cushionsBone morphogenetic proteinBMPPrimary foramenSonic hedgehogSHHSmoothenedSmoWntBeta-cateninLymphoid enhancer-binding factor 1Lef1Platelet-derived growth factorPDGFHaploinsufficiencyTBX5Primary ciliaMicrotubuleIntraflagellar transport proteinIft172PatchedPtc127.1 Introduction
Atrioventricular septal defects (AVSDs) are found in approximately 5 % of individuals with congenital heart disease (CHD). Two major AVSD subtypes can be distinguished based on the potential for shunting between the left and right side of the heart [1]. In “incomplete/partial” AVSDs, shunting is restricted to the atrial level via a primary atrial septal defect (pASD), also known as the ostium primum defect. In “complete” AVSDs, we find, in addition to a pASD, an inlet-type ventricular septal defect (VSD). In complete AVSDs, shunting can occur at both atrial and ventricular level. For many years, it was believed that perturbation of the development of the endocardial AV cushions (or failure of their fusion) was the only mechanism leading to AVSD (hence the term “endocardial cushion defect” as a synonym for AVSD). However, a number of observations in the human heart [2, 3] as well as a growing number of experimental studies have revealed that abnormal development of the posterior second heart field (pSHF)-derived dorsal mesenchymal protrusion (DMP) plays a critical role in the pathogenesis of AVSDs [4–6]. In this chapter, we will discuss several conditions and models in which AVSDs are observed. Unless we specifically mention “complete” or “incomplete”, the term AVSD refers to either form.
27.2 AVSDs and Genetic Syndromes
Down syndrome (trisomy 21), the most common genetic syndrome in humans, is strongly associated with AVSDs: approximately 1/4 of children with Down syndrome have an AVSD, and roughly 2/3 of cases of complete AVSD are found in patients with trisomy 21 [7]. The fact that not all individuals with Down syndrome have an AVSD indicates that the pathogenesis of AVSD in Down syndrome may involve multiple genetic modifiers and/or an environmental component. Other syndromes in which AVSDs are frequently observed include Smith-Lemli-Opitz syndrome (SLOS), Ellis-van Creveld syndrome, Noonan syndrome, and heterotaxy syndrome.
27.3 The Role of the Dorsal Mesenchymal Protrusion (DMP) in the Pathogenesis of AVSDs
27.3.1 The Contribution of the DMP to the AV Mesenchymal Complex
The DMP, sometimes referred to as the “vestibular spine” [2, 3, 8, 9] or “spina vestibuli” [10, 11], plays an essential role in atrial and atrioventricular septation [12–14]. The window in which the DMP develops is relatively narrow. The high proliferative activity of the pSHF cells located ventral to the foregut at around E9-9.5 (murine embryonic development) is responsible for a rapid expansion of this cell population. Around E10-10.5 the expanded pSHF-derived cell population protrudes into the common atrium as the DMP, using the dorsal mesocardium as its portal of entry [11, 13]. Here the DMP becomes a critical player in the septation process. As described in Chap. 5, an important step in atrial septation is the growth and descend of the myocardial primary atrial septum (or septum primum) from the atrial roof into the common atrium [15]. On the leading edge of this septum is located an endocardially derived mesenchymal ridge (also known as the mesenchymal cap). Although this ridge and the DMP have different origins, the two mesenchymal tissues are contiguous with each other. The space located under the primary atrial septum, through which the left and right atria are in open communication with each other, is called the primary foramen (or ostium primum). During normal development, the mesenchymal ridge, the DMP, and the major AV cushions will eventually fuse and close the primary foramen. The body of mesenchyme formed after the fusion of the mesenchymal ridge, DMP, and AV cushions is known as the AV mesenchymal complex [14]. Eventually, the mesenchyme of the DMP, but not the mesenchyme of the ridge and cushions, undergoes a myocardial differentiation [14]. In the formed heart, the muscularized DMP can be recognized as the inferior muscular rim at the base of the atrial septum [14–16].
27.3.2 Failure of the DMP to Properly Develop Results in AVSD
As described above, an important event in the development of the DMP is the proliferation-driven expansion of the pSHF cell population. When the pSHF cell proliferation is inhibited/impaired, the pSHF precursor population does not expand and the DMP fails to develop (or becomes severely truncated). In this case, the DMP will not be able to participate in atrioventricular septation; the AV mesenchymal complex will not form properly and the primary foramen will not be closed. This results in a common AV valve (cAVV) and a primary atrial septal defect (pASD). In addition to cell proliferation, the development of the DMP also involves cell migration [5]. Cell migration is important for organogenesis as well as numerous other developmental processes. Extracellular components, including growth factors, cytokines, and/or extracellular matrix (ECM) proteins, are typically required for the initiation and progression of cell migration [17]. These components may be produced by neighboring cells and/or are present in the extracellular matrix and can be involved in the regulation of intracellular signaling pathway factors, including Rho, Rac, and other members of the small GTPase family that facilitate migration by stimulating extension of lamellipodia, turnover of cell-substrate adhesion, and cell body contraction [18–20]. Perturbation of SHF cell migration has also been proposed to be a mechanism that can lead to inhibition of DMP formation, failure of formation of the AV mesenchymal complex, and eventually resulting in cAVV and pASD which are the common components of most AVSDs [5].
27.4 Emerging Insights into the Molecular Pathways That Are Involved in DMP Development
Insights into the cellular and molecular mechanisms that govern proper DMP development and its maturation are slowly emerging. Below we will discuss a number of studies, performed in recent years on mouse models with AVSDs, which have increased our understanding of how the development and growth of the pSHF and DMP are regulated and have provided insights into the etiology of AVSDs.
27.4.1 The BMP Signaling Pathway and AVSDs
Bone morphogenetic proteins (Bmps) play an important role in cardiovascular development. At least 6 Bmp isoforms are expressed in the heart (Bmp2,4,5,6,7,10). Of these isoforms, Bmp2 has been most frequently studied. Bmp2 is highly expressed in the myocardium of the AV junction at early stages of development [21] where it plays a crucial role in regulating the endocardial-to-mesenchymal transformation necessary for formation of the AV cushions [22]. Mice that do not express Bmp2 (Bmp2 knockout mice) die before E12.5. Embryos isolated at this stage show severe defects in AV cushion development [22, 23]. Bmp4 is also very important for heart formation [24–26]. Mice deficient for this isoform also die early in development [27]. Transgenic Bmp4 hypomorphic mice (i.e., mice that express a severely reduced amount of Bmp4) survive until after birth but die within 1 week. Histological examination of Bmp4 hypomorphic mice shows a 100 % penetrance of AVSD [28]. Furthermore, when Bmp4 is specifically deleted from the myocardium, the offspring also have AVSDs [28]. In this context, it is important to note that Bmp4 is abundantly expressed in the myocardial walls of the dorsal mesocardium (a.k.a. the mesocardial reflections) but that Bmp4 is not expressed in the myocardial AV junction [4]. The mesocardial reflections flank the space through which the expanding pSHF protrudes into the common atrial cavity [1, 12]. In a recent study, it was shown that the pSHF population that eventually will form the DMP expresses BmpR1A/Alk3, a Bmp receptor known to interact with BMP4. Furthermore, positive staining for activated pSmad1,5,8 in this cell population indicates ongoing BMP signaling. The importance of BmpR1A/Alk3-dependent Bmp signaling for the formation of the DMP and atrioventricular septation was demonstrated in experiments in which BmpR1A/Alk3 was conditionally deleted from the SHF using the myocyte enhancer factor 2C (Mef2C)-AHF-Cre mouse line. SHF-specific deletion of Alk3 resulted in impaired formation of the DMP and a completely penetrant incomplete AVSD (ostium primum defect) phenotype at stages ED13.5–15.5. Analysis of Mef2C-AHF-Cre;Alk3 fl/fl mutants at E10-10.5 revealed decreased proliferative index of SHF cells and, consequently, a reduced number of pSHF cells at the cardiac venous pole [4].
27.4.2 The (Sonic) Hedgehog Signaling Pathway and AVSDs
Sonic hedgehog (Shh) is a secreted morphogen that plays an important role in the development of the heart. Specifically, Shh is crucial for regulating SHF contributions to the cardiac inflow tract, to the cardiac outflow tract, and to cardiac septation [5, 29, 30]. Interestingly, while Shh knockout mice have severe cardiac defects [31, 32], Shh is not expressed within the heart itself. This indicates that extracardiac sources are involved in Shh-dependent regulation of heart development. One of the tissues secreting Shh is the ventral pharyngeal endoderm [30]. Conditional deletion of Shh with an NK2 homeobox 5 (Nkx2-5)-Cre mouse, driving expression in a number of tissues including the pharyngeal endoderm, leads to a fully penetrant AVSD phenotype [30]. Shh signaling involves two co-receptors. Smoothened (Smo) is a G-protein-coupled-like receptor acting downstream of Patched (Ptc1) to confer Shh signaling; when secreted Shh binds to Ptc1, it abolishes an inhibitory effect on Smo, allowing Smo to transduce signals via Gli transcription factors. Shh expressing ventral pharyngeal endoderm is juxtaposed to the SHF/DMP-precursor cell population located between the foregut and the heart. Conditionally deleting Smo from the SHF/DMP-precursor cell with the Mef2c-AHF-Cre mouse (Mef2c-AHF-Cre;Smo fl/- ) inhibits Shh signaling in these cells, perturbs DMP formation, and results in a fully penetrant AVSD phenotype [5]. Thus, interaction between Shh (expressed in and secreted from pharyngeal endoderm) and the Shh receptor Smo (expressed in the SHF/DMP-precursor cell population) is essential for the development of the DMP and, hence, for proper AV septation.
27.4.3 The Wnt/β-Catenin Signaling Pathway and AVSDs
The wingless-type MMTV integration site family Wnt/β-catenin signaling pathway is involved in many developmental events. In the heart, Wnt/β-catenin signaling has been shown to regulate the development of the outflow tract and right ventricle, components of the heart that are derived from the SHF [33, 34]. In addition, the Wnt/β-catenin signaling pathway is also import for the development of the venous pole of the heart. Deletion of β-catenin with the islet 1 (Isl1)-Cre mouse (like the Mef2c-AHF-Cre mouse driving Cre-expression in the SHF) results in embryonic lethality, pharyngeal arch defects, and cardiac malformations including AVSD (pASD) [35]. Conditional deletion of β-catenin results in reduction of proliferation of SHF-derived cardiac progenitor cells and significant downregulation of a number of downstream genes including Isl1, T-box (Tbx) 3, Wnt11, and Shh [35]. One specific Wnt variant found to be directly associated with the development of the posterior SHF and the DMP, and therefore with atrioventricular septation, is Wnt2 [6]. At the critical stages of DMP development (ED9.5–10.5), Wnt2 and lymphoid enhancer-binding factor 1 (Lef-1), a central transcription mediator of Wnt/β-catenin signaling, are expressed in the SHF mesodermal cell population and the DMP. Wnt2 and Lef-1 are, however, not expressed in the ventricles, AV canal, or AV cushions [6, 36]. Mice that do not express Wnt2 (Wnt2 null mutants) show reduced expression of axin2, Isl1, Lef1, and fibroblast growth factor 10 (Fgf10), a direct target of Wnt/β-catenin signaling [6, 34]. Staining for Ki67 shows a decrease in proliferative activity of the SHF/DMP-precursor population, and histological analysis reveals severe inhibition of DMP development. The proliferation defect and associated failure of the DMP to properly develop resemble what is observed in the conditional SHF-Alk3 knockout mouse [4] (see also above). Approximately 85 % of Wnt2 null mutants die at birth, and histological analysis of Wnt2 null specimens reveals the presence of AVSDs. LiCl is a pharmacological inhibitor of Gsk-3b and a strong activator of Wnt/β-catenin signaling. Administration of LiCl to pregnant female mice carrying ED8-10 mutant Wnt2 embryos leads to an elevation of Lef1 expression in the posterior SHF, restores SHF cell proliferation, rescues the AVSD phenotype, and increases the survival rate of Wnt2 null mutant offspring.
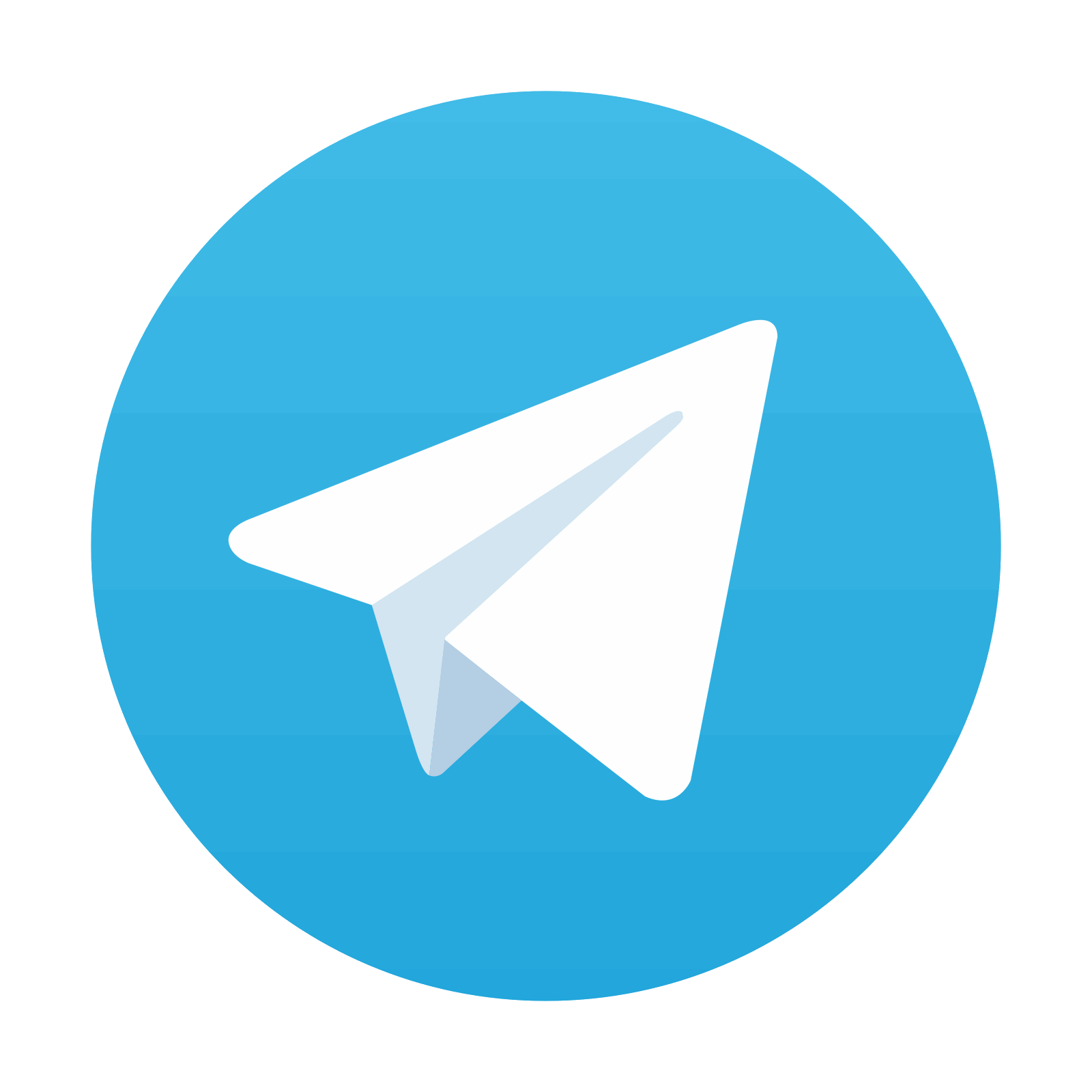
Stay updated, free articles. Join our Telegram channel

Full access? Get Clinical Tree
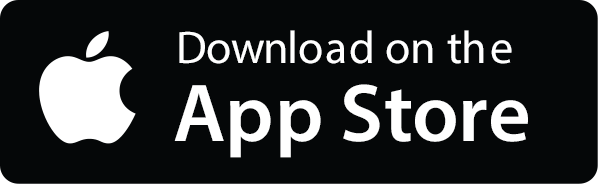
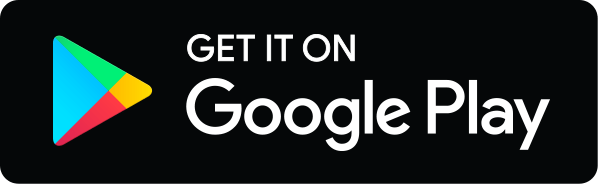