Fig. 21.1
Details of atrial septation and venous return in the developing mouse heart are shown in a series of episcopic images. (a, b) Images of an E10.5 heart are shown in the frontal (a) and transverse (b) planes. The right and left sinus horns (RSH, LSH) drain into to the right atrium (RA). The left sinus horn, which gives rise to the coronary sinus, never shares a common wall with the left atrium (LA) during development. The pulmonary pit (*) is situated between the right and left pulmonary ridges (RPR, LPR). Second heart field progenitors migrate through the dorsal mesocardium (DM) into the right pulmonary ridge to form the vestibular spine. (c, d) In two E11.5 hearts, the relationships are shown between the primary atrial septum (PAS), its mesenchymal cap (MC), the developing vestibular spine (VS), and the inferior endocardial cushion (IEC). The primary and secondary atrial foramina are noted (PAF, SAF). (c) is cranial to (d). (e) In this E14.5 heart, fusion of the mesenchymal cap of the primary atrial septum, the vestibular spine, and the endocardial cushions has closed the primary atrial foramen. The primary atrial septum acts as a flap valve on the foramen ovale (FO). (f) An ostium primum defect (arrow) in this E18.5 heart results from persistence of the primary atrial foramen. (g, h) In this E18.5 heart, the atrial septum is shown (g) at the level of the pulmonary vein and (h) more cranially. Incorporation of the pulmonary vein (PV) into the left atrium causes infolding of the dorsal atrial wall (arrowhead, g). A muscular rim, not present in humans, exists at the site of breakdown at the atrial roof of the primary septum (arrowhead, h) (The episcopic image datasets were prepared by Dr. Timothy Mohun of the Francis Crick Institute, London)
The right superior and inferior vena cava will drain to the right sinus horn, while the left superior vena cava will drain through the left sinus horn, opening to the right atrium as the coronary sinus. The left superior vena cava persists in the mouse but normally regresses in humans. Rotation of the sinus horns ultimately places the coronary sinus in the dorsal atrioventricular groove. Although the coronary sinus runs adjacent to the left atrium, each has its own wall from the outset of development (Fig. 21.1a) [6].
In humans, the solitary pulmonary vein that initially opens through the pulmonary pit becomes incorporated into the roof of the left atrium, eventually achieving four discrete orifices [7]. This does not occur in the mouse: the right and left pulmonary veins connect to a common vein to the left atrium adjacent to the dorsal atrioventricular groove [8].
At E10, the primary atrial septum, which develops from second heart field progenitors, is first seen growing from the dorsal roof of the common atrium toward the endocardial cushions of the atrioventricular canal (Fig. 21.1c). Its leading edge has a mesenchymal cap, which is derived from the endocardium [9]. At the caudal end of the primary atrial septum, the mesenchymal cap is continuous with the developing vestibular spine (Fig. 21.1d). The primary atrial foramen, or ostium primum, is thus bounded by the edge of the primary atrial septum with its mesenchymal cap, the vestibular spine, and the atrial surface of the endocardial cushions. By E12, fusion of the mesenchymal cap, vestibular spine, and endocardial cushions closes the primary atrial foramen. Persistence of the primary atrial foramen, in other words an ostium primum defect, results from failure of the development of the vestibular spine (Fig. 21.1e, f) [9–11].
At E10.5, fenestrations appear in origin of the primary atrial septum at the atrial roof as the septum grows toward the atrioventricular canal. The fenestrations coalesce to form the secondary atrial foramen, which is essential during fetal life for right-to-left shunting of oxygenated blood after closure of the primary atrial foramen (Fig. 21.1c). The primary atrial septum thus becomes the flap valve of the foramen ovale (Fig. 21.1e). At E13, the dorsal rim of the foramen ovale forms by the infolding of the atrial wall between the pulmonary vein and left venous valve of the right atrium (Fig. 21.1g). The cranial rim is a muscular ridge that remains at the site of breakdown at the atrial roof of the primary septum (Fig. 21.1h). Infolding is a consequence of the incorporation of the pulmonary vein into the left atrium. The infolded rim of the foramen is commonly referred to as the secondary atrial septum or septum secundum, but it is not a true septum, since a hole through it will enter a potential space outside the heart. The ventral-caudal rim of the foramen, which buttresses the flap valve to the atrioventricular junctions, is formed by muscularization of the mesenchymal cap of the primary atrial septum and vestibular spine (Fig. 21.1e–h) [9–11]. This rim is a true septum, as illustrated by a rare vestibular defect in an Nkx2–5 +/− mouse (Fig. 21.2a) [12].
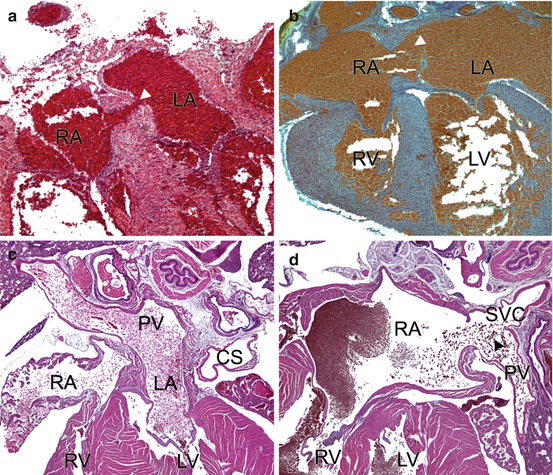
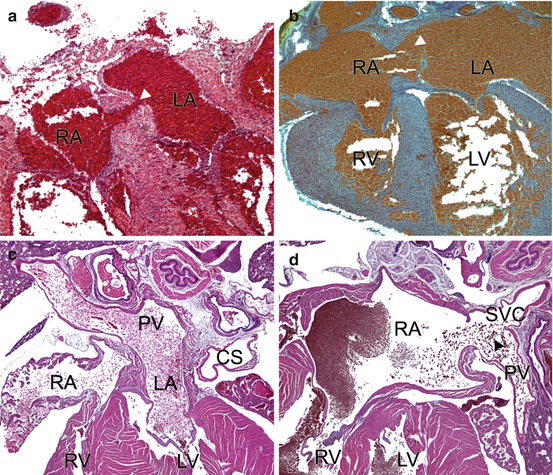
Fig. 21.2
Examples of atrial septal defects and an anomalous venovenous communication. (a) A vestibular defect and (b) a secundum ASD in newborn Nkx2–5 +/− mice. (c) Normal pulmonary venous drainage is shown into the left atrium of a mouse. (d) The pulmonary vein communicates anomalously with the superior vena cava in a Sema3d −/− mouse. Arrowheads indicate the defects (Mr. Suk Dev Regmi prepared the sections shown in a, b. Mr. Ehiole Akhirome discovered the vestibular defect. Both are at Washington University School of Medicine, St. Louis) CS coronary sinus, PV pulmonary vein, RA, LA right, left atrium, RV, LV right, left ventricle, SVC superior vena cava
The embryologic relationship of the systemic and pulmonary venous return was hotly debated in the past, but multiple studies in human and mouse now clearly establish their separate development. A recent analysis on the chick embryo demonstrates this elegantly with three-dimensional reconstructions. The pulmonary veins in the lungs develop from a plexus within the splanchnic mesoderm. They subsequently connect to the main pulmonary vein that develops at the pulmonary pit. The systemic venous sinus originates from the junction between the splanchnic and somatic mesoderm. The systemic veins develop in the splanchnic mesoderm caudal to the pulmonary venous plexus. There is no evidence for anastomoses between the two venous systems in the normal embryo [13]. Studies of developing human hearts also support the separate developmental origins of the systemic and pulmonary veins [14].
21.3 Pathways to a Defect within the Oval Fossa (Secundum ASD)
Most experimental studies of atrial septation to date pertain to the pathogenesis of the primum rather than secundum defect. Unfortunately, developmental biologists often do not distinguish the two defects, describing mutant phenotypes merely as “ASDs.” A secundum ASD is the consequence of deficiency of the primary atrial septum, such that it cannot cover the complete area of the foramen ovale (Fig. 21.2b). In the usual case, the primary atrial septum does not overlap the infolded rim of the foramen. Fenestrations in the primary atrial septum also occur but are less common. Of note, the vestibular spine and the mesenchymal cap, forming the buttress of the foramen, have developed and muscularized normally, which is not the case in the primum defect, which is an atrioventricular septal defect [9–11, 15].
An appreciation of normal development and the morphology of defects within the oval fossa can help to circumscribe the mechanisms of pathogenesis. Mutations that cause secundum defects likely relate to the development of the secondary atrial foramen. On the other hand, deficient growth of the primary atrial septum from the atrial roof to the atrioventricular canal is unlikely to explain the secundum defects. Experiments with Tbx5 (T-box 5) and Nos3 (nitric oxide synthase 3) mutants offer a glimpse into a specific cellular and molecular mechanism.
Human and mouse TBX5 mutations are commonly associated with secundum ASD, although other malformations, including atrioventricular septal defects, are also seen [16–19]. Independent functions of Tbx5 in the endocardium and the second heart field can explain the pathogenesis of both secundum and primum defects, respectively. These functions have been explored in a series of conditional deletion experiments, in which one or both copies of a gene are deleted in a specific cell type or developmental period. In the embryo, Tbx5 is expressed by the endothelial cells lining the primary atrial septum. Conditional knockout of Tbx5 in endothelial cells increased the number of apoptotic cells in the developing primary septum. The adult conditional knockout mice had near-complete absence of the primary atrial septum, while the conditional heterozygotes had a high incidence of patency of the foramen ovale. Endothelial knockout of Tbx5 did not affect the vestibular spine [20]. The diminished activity of Tbx5 in the endothelium thus causes defects within the oval fossa, or the forme fruste as seen in persistent patency of the foramen ovale. On the other hand, conditional deletion of Tbx5 in the second heart field prevented the development of the vestibular spine, resulting in primum defects [21].
The molecular pathways that lead to a secundum ASD are mostly undefined, but one has been suggested for Tbx5 and Gata4 (GATA binding protein 4). Mutations of the latter also cause secundum defects in both man and mouse [22–24]. In an endocardial cell culture model, TBX5 upregulates the expression of nitric oxide synthase 3 (Nos3, also known as eNOS or endothelial NOS). In the same cell culture system, TBX5 and GATA4 independently and cooperatively transactivate a Nos3 promoter construct [20]. Nos3 null mutant mice have a high incidence of defects within the oval fossa. The thinness of the primary atrial septum may make it more susceptible to apoptosis caused by the loss of Nos3 [25]. Furthermore, compound heterozygotes, in which one allele of Tbx5 is deleted in the endothelium and one allele of Nos3 in the germline, have a higher incidence of secundum defects than either single heterozygote. Nos3 is expressed in both the endocardial cells and myocytes, so its activity in one or both cell types may be necessary to prevent myocyte apoptosis. Taken together, the results suggest that Tbx5, Gata4, and Nos3 operate in a molecular pathway involving an endocardial signal that inhibits myocyte apoptosis.
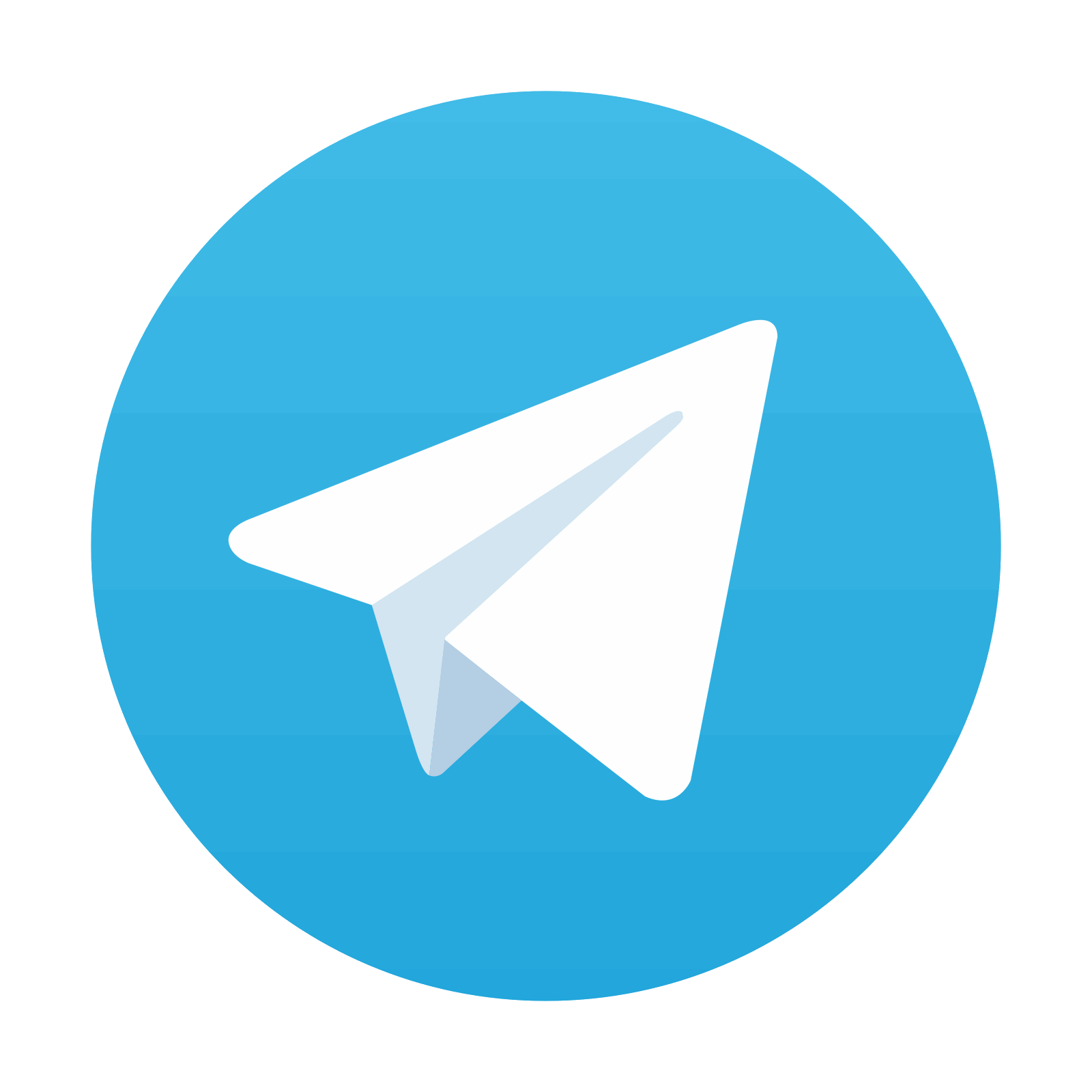
Stay updated, free articles. Join our Telegram channel

Full access? Get Clinical Tree
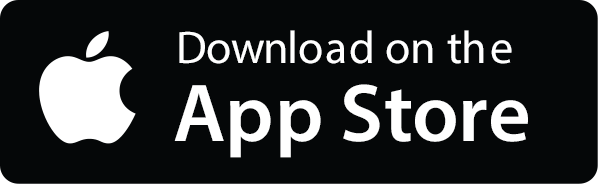
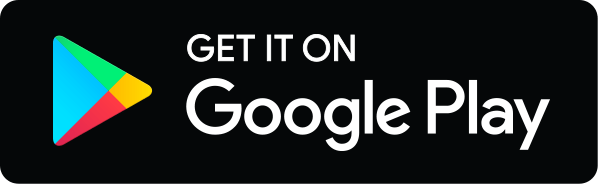