Fig. 63.1
Polymorphic ventricular arrhythmia phenotypes in mouse model. Representative ECG recordings from wild-type and ankyrin-B± mice at baseline and following exercise and treatment with adrenergic agonist. Center recording for ankyrin-B± mouse illustrates typical example of arrhythmia lasting minutes from this mouse model (Reprinted from DeGrande et al. [18], with permission from Elsevier)
63.2.3 Short QT Syndrome
The majority of short QT syndrome (SQTS) variants result from a gain-of-function of potassium channel subunits; however, phenotypic plasticity has been noted in some forms of SQTS [3]. Penetrance in probands and murine models has demonstrated varying degrees of severity in QT shortening because of the variant’s effect on associated subunits of the potassium channel. While a host of variants have been identified (i.e., SQTS4 with CACNA1C), this section will focus on SQT1 and SQT3.
Gain-of-function variants in I Kr have been closely associated with SQTS1, namely, through the Asn588Lys missense variant. Under normal physiologic conditions, HERG allows timely repolarization of the ventricles and returns to resting membrane potential; however, Asn588Lys channels have been shown to increase the amplitude of I Kr and shorten the action potential duration resulting in arrhythmogenesis [10]. The introduction of a positive charge in the channel pore increases the energy requirement for channel inactivation, leading to greater repolarization earlier in the action potential and shorter refractory periods. This change leaves the ventricles susceptible to premature stimulation. Of note, changes in extracellular potassium concentrations do not produce a change in channel conductance, indicating a disruption in K+ permeability as the primary pathogenic mechanism. Corderio et al. produced a mouse model showing that arrhythmogenesis may begin in SQTS1 when the shorter ventricular refractory period produced by short action potential durations is combined with the longer Purkinje cell action potential duration in the ventricles, producing a heterogeneous repolarization and resultant polymorphic ventricular tachycardia [10].
Accelerated repolarization and shortened QT intervals also are associated with variants in KCNJ2, resulting in gain-of-function of the Kir2.1 channel protein. The Kir2.1 channel sustains resting membrane potentials, modulates cardiac excitability, and participates in determining the length of the QT interval by way of an inward rectifying current, I K1, during the repolarization phase of the action potential [11]. SQTS3 is characterized by an asymmetric T wave specific to an Asp172Asn Kir2.1 mutation demonstrating slow ascending and rapidly descending limbs [12]. The highly conserved 172 position is integral for normal channel blocking, but its function can be disrupted with an acidic to neutral residue change as seen in Asp172Asn, allowing an increased outward current shortening repolarization [12]. Guinea pig myocytes with overexpressed Kir2.1 channels produced an increased I K1 density, duplicating the gain-of-function pathogenic mechanism as seen in SQTS3. Moreover, increased outward current in mice overexpressing Kir2.1 showed an increased rate of change in membrane potential during the last phase of the action potential, producing a shortened repolarization and subsequent QT as seen in SQTS [12]. These changes were arrhythmogenic in both the ventricles and atria of transfected mice, inferring that the same mutation may increase risk for both ventricular and atrial fibrillations in an individual with SQTS3.
63.2.4 Catecholaminergic Polymorphic Ventricular Tachycardia
Catecholaminergic polymorphic ventricular tachycardia (CPVT) is found in autosomal dominant, recessive, and idiopathic forms. The most common genetic mechanism for CPVT is autosomal dominant gain-of-function variants in the RYR2 gene responsible for the assembly of the RyR2 or ryanodine receptor [3]. This protein controls the release of Ca2+ from the sarcoplasmic reticulum by way of L-type Ca2+channel signaling and thus is central to the timely stimulation of sarcomeres that produce heart muscle contraction. Mutations in RyR2 and associated proteins can cause premature channel opening and Ca2+ release leading to delayed afterdepolarizations, bidirectional ventricular tachycardia, and ventricular fibrillation upon β-adrenergic stimulation [1]. Although about 70 RyR2 variants have been identified, the mechanisms by which CPVT originates from these mutations can be largely attributed to the effects on RyR2 luminal Ca2+sensitivity, binding to association proteins, sarcoplasmic reticulum Ca2+ load, and lowered initiation threshold [1]. Several RyR2 Arg4496Cys knockin mouse models show that β-adrenergic stimulation induces CPVT Ca2+ waves by increasing store overload-induced Ca2+ release (SOICR), producing a higher Ca2+ and lower sarcoplasmic reticulum Ca2+ levels during resting state, which are susceptible to the Arg4496Cys lowered Ca2+ wave initiation threshold. Additionally, Purkinje cells in this model were more vulnerable to changes in intracellular Ca2+ leading to early and delayed afterdepolarizations, inferring a significant role in CPVT arrhythmogenesis [1]. The heterogeneity of a single RyR2 mutation was demonstrated by Loaiza et al. showing altered RyR2 cytosolic Ca2+ sensitivity, protein kinase A phosphorylation, and luminal Ca2+ modulation in Val2475Phe mice [11]. Similarly, Val2474Ser mice developed atrial fibrillation as a result of increased SOICR and lowered induction threshold in atrial cardiomyocytes. A final CPVT murine model involved a reduced binding affinity and decreased RyR2 association to FKBP12.6 that normally serves to stabilize the RyR2. Dissociation of these proteins in FKBP12.6 −/− mice was shown to destabilize the closed state of RyR2, allowing uncontrolled Ca2+ release and inducing ventricular tachycardia. While it is clear the pathogenic mechanisms of specific CPVT mutations are varied, Loaiza et al. asserted that the production of delayed afterdepolarizations may be a point of convergence for RyR2 mutations and the CPVT phenotype [11]. Finally, animal models of human CPVT arrhythmias have yielded new information on the mechanisms underlying disease. For example, human mutations in calsequestrin 2, a sarcoplasmic reticulum calcium-binding protein, result in CPVT. Unexpectedly, Casq2 −/− mice display defects in the sarcoplasmic reticulum size, as well as defects in calcium release and polymorphic arrhythmia [13].
63.2.5 Early Repolarization Syndrome
Increase of ventricular I to, I KATP, or I K,Ach current and decrease of I Ca,L and I Na current are suspected in the arrhythmogenesis of early repolarization syndrome (ERS); however, the exact mechanism is still unknown. ERS shares a close phenotype with Brugada syndrome as evidenced by its intermittent concomitant presentation in some patients, with diagnosis differentiating primarily on the area of the heart affected. Genotypes of ERS demonstrate heterogeneity and variants have been identified in six genes.
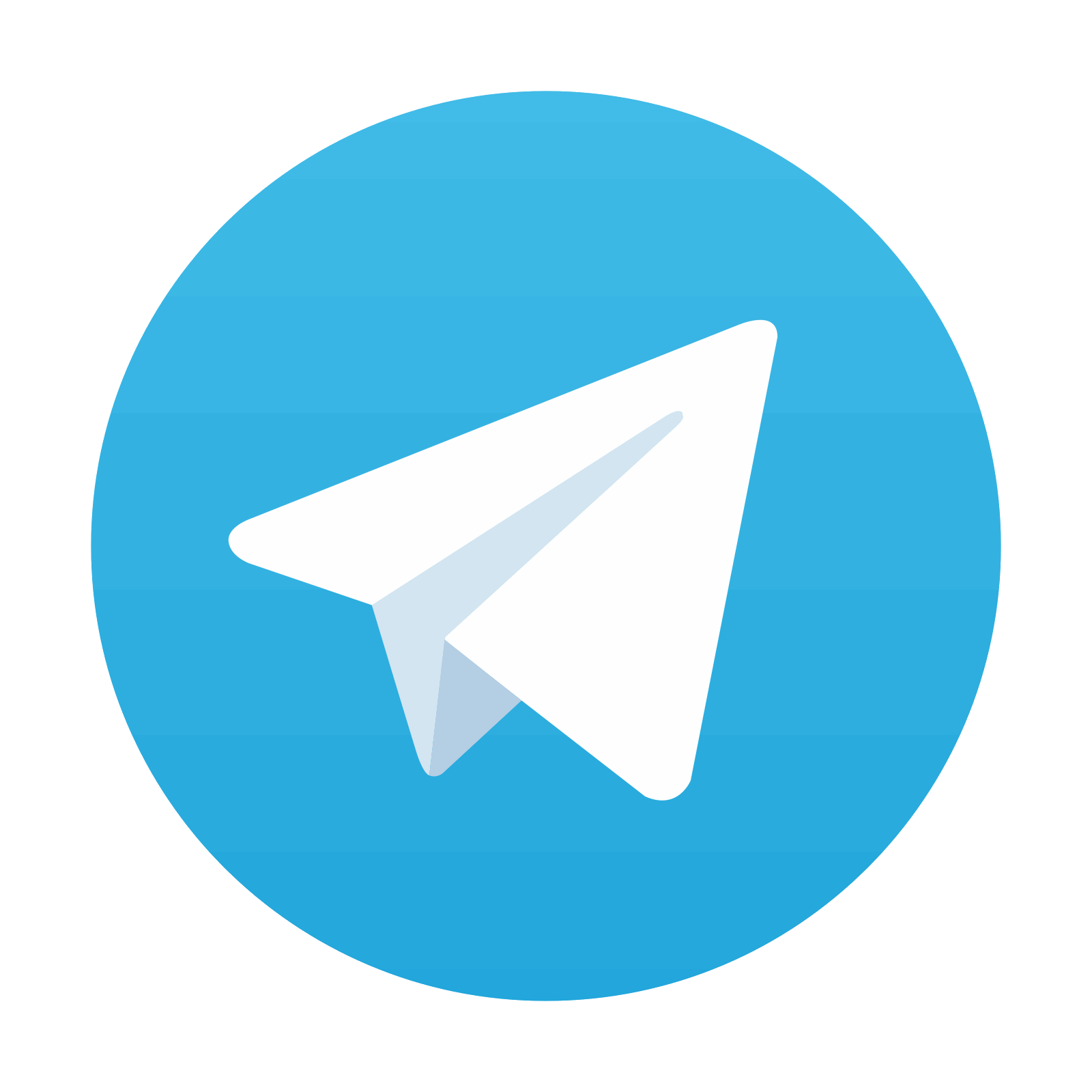
Stay updated, free articles. Join our Telegram channel

Full access? Get Clinical Tree
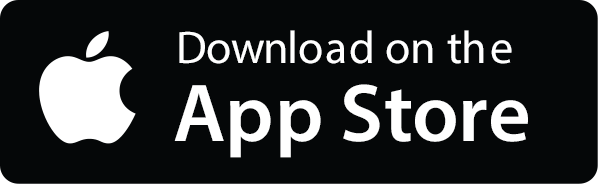
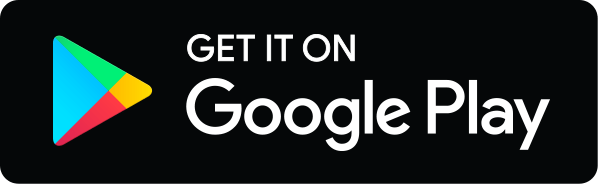