(1)
Institute of Pathology, Medical University Graz, Graz, Austria
19.1 Introduction
Within the last decade, many important discoveries were made in the regulation of growth, differentiation, apoptosis, and metastasis of lung cancers. These findings have dramatically changed the “ignorance” in the oncology community about the classification of lung carcinomas. A decade ago, oncologists were mainly interested to get the differentiation between small cell (SCLC) and non-small cell carcinomas (NSCLC) of the lung. With the findings of different responses for cisplatin and anti-angiogenic treatment in adenocarcinomas versus squamous cell carcinomas, this simple clinical lung carcinoma classification schema was abolished. Now oncologists want to know the differentiation within NSCLC, and the near future will even increase subtyping of the different NSCLC entities. In this review, we will first focus on general aspects of molecular pathology in lung carcinomas and then discuss different genetic abnormalities within the different entities. These abnormalities will be ordered according to their importance such as targeted therapy and impact on outcome.
19.2 Therapy Relevant Molecular Changes in Pulmonary Carcinomas
19.2.1 NSCLC and Angiogenesis
In the last decade, humanized antibodies have been developed to interfere with the neoangiogenesis in primary as well as metastatic carcinomas [1, 2]. However, anti-angiogenic drugs can cause severe bleeding, especially when administered in patients with centrally located squamous cell carcinomas [3, 4]. However, it is still not clear if the reported bleeding episodes in these patients are due to the squamous histology or more logically to the centrally located tumors, which are usually supported by arteries and veins arising from large branches. In addition it was reported that cavitation within the tumor is prone to hemorrhage, again something more common in central tumors located close to large blood vessels [5].
Angiogenesis, better neoangiogenesis, is a process by which primary tumors get access to nutrients and oxygen. The process of neoangiogenesis is still not fully understood. In some cases, the tumor cells themselves produce angiogenic factors such as vascular endothelial growth factors (VEGFs); in other cases, these growth factors are produced by macrophages present in the tumor microenvironment [6]. However, once new blood vessels (capillaries, small arteries, veins) are formed, this provides advantage for the tumor cells over their normal neighbor cells in getting better oxygen and nutrient supply. Nutrients and oxygen are not the only important factor for better growth; also purine and pyrimidine bases are essential for a dividing tumor cell [7–10]. A good example on how this can influence the progression from preinvasive to invasive lesions is the vascular variant of squamous cell dysplasia. It seems that the early access to blood vessels promotes rapid progression into squamous cell carcinoma [11]. In another preneoplasia, atypical adenomatous hyperplasia (AAH) vascularization is a late event, usually at the transition from in situ to invasive adenocarcinoma [12, 13]. This might explain why AAH can persist for several years without progression [14]. In addition there seems to exist a difference between mucinous and non-mucinous adenocarcinomas with respect to neoangiogenesis [15]. Increased angiogenesis itself in invasive adenocarcinomas has a negative impact on survival and progression of these patients [16].
Angiogenesis is essential for the primary tumor as well as for metastasis. The secretion of VEGFs facilitates most often neoangiogenesis. Tumor blood vessels are fragile and are prone to rupture. Using antibodies against VEGF (bevacizumab), the vascularization can be inhibited, and regression of the tumor is induced. In centrally located tumors, therapy can result in severe hemorrhage. Therefore, the use of bevacizumab is recommended for adenocarcinomas and large cell carcinomas, but squamous cell carcinomas are excluded – SCC is most often a centrally located tumor.
New developments are focusing on the inhibition of the VEGF receptors and also on the role of HIF and hypoxia in tumor development and metastasis. In several studies, the importance of VEGF and VEGFR axis was stated for vascular invasion and metastasis, mainly involving VEGF-C and VEGFR3 [16–19]. Studies aiming to target this axis showed positive results in experimental settings [20–23]. Bringing these targeted therapies into clinical trials is still in its infancy [24, 25]. A major problem in targeting VEGF-VEGFR is the fact that its regulation is under the major influence of the hypoxia pathway. Hypoxia is an important factor in invasion and angioinvasion, and HIF1α signaling will result in the upregulation of VEGF [26, 27]. So the hypoxia pathway might constantly overrule a blockade of VEGF-VEGFR unless also HIF1α production is also inhibited [28].
19.2.2 NSCLC and Cisplatin Drugs: The Effect of Antiapoptotic Signaling
In a large multi-institutional study, the effect of cisplatin chemotherapy was investigated. High expression of DNA repair enzymes, especially nucleotide excision repair enzyme (ERCC1), was found to be responsible for failure of cisplatin chemotherapy, and this expression correlated predominantly with squamous cell histology [29, 30]. ERCC1 is part of the excision repair machinery involved in the repair of damaged DNA. In NSCLC showing a high expression of this enzyme, the action of cisplatin-based chemotherapeutics is inefficient, most probably because DNA damage induced by the drug is immediately repaired. Therefore, ERCC1 should be investigated by immunohistochemistry to predict response to therapy especially in squamous cell carcinomas.
19.2.3 Thymidylate Synthase Blocker
Pemetrexed is an inhibitor of thymidylate synthase (TS) less for the other enzymes in the thymidine cycle. Thymidine uptake is essential for rapidly dividing carcinoma cells. In tumors with low expression of TS, pemetrexed can block the enzyme resulting in growth inhibition. TS expression most often is low in adenocarcinomas, but is highly expressed in many squamous cell carcinomas. Thus, pemetrexed is efficient in most adenocarcinomas and not in squamous cell carcinomas [31]. However, the action of pemetrexed is still not entirely clear: thymidylate metabolism does not only rely on enzymes of the thymidylate cycle but also needs active and passive uptake mechanisms; and thymidine uptake might also be influenced by pemetrexed [32, 33].
19.2.4 Receptor Tyrosine Kinases in Lung Carcinomas
Receptor tyrosine kinases (RTK) are membrane-bound protein receptor composed of an external receptor domain, a transmembrane spanning portion, and an internal domain, which at its C-terminal end contains the kinase domain. The external receptor domain has a specific configuration for the binding of growth factors, where usually two molecules form homo- or heterodimer with the receptor domain. This specific binding changes the configuration of the whole receptor and leads to the activation of the kinase domain. There are two ways of activation of receptor tyrosine kinases in lung cancer: overproduction of ligands either by the tumor cell or by cells within the microenvironment, such as macrophages, and activation by a mutation of the receptor gene, most often within the kinase domain. The receptor kinase itself can act also in two different ways: one is transfer of phosphorylation to transfer molecules [34–38], like GAB1 or Grb2, and the kinase splits into fragments, where one activated protein fragment translocates into the nucleus and binds to specific DNA elements and induces transcription of proteins [39–41]. In lung cancer, RTKs can be constantly activated by different mechanisms: amplification of the RTK gene, mutations of the RTK gene, and gene rearrangements (translocation/inversion) with constant activation or inactivation of regulatory proteins. Another mechanism is downregulation of regulatory proteins by miRNAs, so a tumor suppressor or a negative feedback protein is not synthesized because of mRNA inactivation by miRNA [42–48].
19.2.5 TP53: The Tumor Suppressor Gene
TP53 was one of the first tumor suppressor genes detected as being mutated in almost every cancer type. TP53 is located on chromosome 17p13-12, contains 11 exons, and has two promoters (one upstream of noncoding first exon and another within first intron) [49]. The protein p53 functions as a cell cycle control, which can send cells with defective DNA directly into apoptosis [50]. TP53 is either mutated or methylated in most lung carcinomas, especially in all tobacco smoke-associated variants. Analysis of these types of mutations highlighted some nonfunctional mutations most probably due to interaction with some tobacco genotoxic compounds [51–56], whereas other mutations resulted in truncation of the protein, defect of protein degradation, and loss of function [57–60]. Mutations and methylation-induced silencing are common in SCLC and squamous cell carcinomas and less frequently in adenocarcinomas [52, 61, 62]. There is still an ongoing debate if p53 inactivation is related to a metastatic phenotype [63–65].
Next we will focus more specifically on tumor entities and what molecular profiles are known in each entity.
19.3 Adenocarcinomas
Adenocarcinomas in highly industrialized countries are the most common lung carcinoma, with a percentage of 40 % of all lung carcinomas. In addition what was previously regarded as a single entity has become a huge diversity of carcinomas. Adenocarcinomas in never-smokers most probably represent a separate entity with different gene signatures and a slower progression rate compared to adenocarcinomas in smokers. Also gene signatures have contributed to a more heterogeneous picture. Morphologically adenocarcinomas can show a variety of patterns, which in part correlate with gene signatures, although our knowledge in this respect is still in its infancy.
Adenocarcinoma is defined by the formation of papillary, micropapillary, cribriform, acinar, and solid structures, the latter with mucin synthesis – mucin-containing vacuoles in at least 10 % of the tumor cells. Adenocarcinomas can be either mucinous or non-mucinous. Both will show the above mentioned patterns. Some rare variants are fetal, colloid, and enteric adenocarcinomas. Most often a mixed pattern is seen with a predominance of at least one component.
Tumor cells in adenocarcinomas can show differentiations along well-known cell types as Clara cells, pneumocyte type II, columnar cells, and goblet cells. Due to the importance of targeted therapy, the exact classification of adenocarcinomas and their differentiation from other NSCLCs have become a major task in pulmonary pathology. Differentiation factors are used to prove the nature of the carcinoma especially in less well-differentiated examples. A variety of useful markers for have been tested; the most important ones are TTF1 and Napsin A.
19.3.1 EGFR
In 2004 an epidermal growth factor receptor (EGFR) mutation was detected in a patient with lung adenocarcinoma and responded to tyrosine kinase inhibitor treatment – a new era of targeted therapy in NSCLC was invented [66, 67].
Mutation of EGFR has been detected in a small percentage of lung cancer patients in the Caucasian population. In Southeast Asians the percentage can rise to 60 %. These are activating mutations found in exons 18, 19, 20, and 21 of the EGFR gene (kinase domain) [68]. Mutations are most often found in never-smokers, females, and patients with adenocarcinoma histology. Mutations change the configuration of the kinase, which does not need anymore the ligand-based activation from the receptor domain. The receptor stays in an activated stage and constantly signals downstream. Carcinomas with this activating mutation can be growth inhibited by small receptor tyrosine kinase inhibitors (TKI) such as gefitinib, erlotinib, and afatinib. These TKIs bind either reversible or irreversible into the ATP pocket of the mutated EGFR kinase and thus inhibit phosphor-transfer to downstream molecules, thus blocking the signaling cascade [69]. The most common mutations are deletions within exon 19 with a variation of 9–18 nucleotides and a point mutation at exon 21. Other less common mutations are point mutations in exon 18 and insertions in exon 20.
However, mainly within exon 20, there are also resistance mutations; the best known is T790M. This type of mutation inhibits or reverses the binding of the TKIs gefitinib and erlotinib and prevents the receptor blockade. The irreversible TKI afatinib might overrule some of these resistance mutations, but more data are needed to prove this [70, 71].
For targeted therapy with TKIs, tissue samples of NSCLC have to be analyzed for these mutations. Within the different subtypes of adenocarcinomas, some will show a higher percentage of EGFR mutations, whereas others do not. In Caucasian population, adenocarcinomas with acinar or papillary pattern are up to 27 % mutated, whereas mucinous adenocarcinomas are constantly negative for EGFR mutations. Carcinomas with biphasic morphology such as adenosquamous carcinomas and mixed small cell and adenocarcinomas can show mutations but usually in a very small percentage.
Another therapy approach was tested with humanized monoclonal antibodies for EGF. By competitive binding to the receptor, this antibody replaces EGF and thus inhibits transactivation of the kinase. This type of therapy seems to be especially promising in EGFR-naïve (wild-type) adenocarcinomas and in addition also in squamous cell carcinomas [72, 73].
19.3.2 KRAS
KRAS was one of the early detected oncogenes in adenocarcinomas of the lung. KRAS belongs to the family of small GTPases located close to the inner cell membrane. They can be activated by tyrosine receptor kinases either membrane bound as EGFR or also by cytosolic kinases as SRC. Usually phosphorylated transfer molecules activate them as GRB2 [74–76]. Once activated, they can signal downstream into three major cascades: RAL-RAF, MEK-ERK, and PI3K-AKT. These different activation cascades have different effects on tumor cells; however, the exact interaction and the mechanisms, which select a specific signaling pathway, are not clear [77, 78].
Mutations of KRAS in lung adenocarcinomas are found in the codon 12, 13, and 61. These mutations result in constant activation of KRAS and consecutively activation of the downstream cascades. KRAS in this situation does not need an upstream activation.
KRAS mutations occur at an average of 30 % of all pulmonary adenocarcinomas, but this percentage rises to 50 % in mucinous adenocarcinomas [79]. In one study, KRAS mutations were more frequently seen in solid adenocarcinomas [80]. Whereas KRAS mutations are frequent in Caucasian, these are rare in Southeast Asian population, opposite to the situation of the frequency of EGFR mutations [81–84].
Targeted therapy this moment does not exist for patients with mutated adenocarcinomas, but there are trials going on, which aim to inhibit the downstream signaling pathway with MEK, ERK, and mTOR inhibitors [25].
19.3.3 EML4ALK1 and Additional Fusion Partners
Inversion (erroneously called translocation) of the ALK1 kinase gene and fusion with the EML4 gene have been recently shown in patients with NSCLC, especially in solid adenocarcinomas with focal differentiation into signet ring cells. Subsequently other patterns have been associated with this type of gene rearrangement, such as micropapillary. Both genes are on chromosome 2; the chromosomal break is inversely rearranged whereby the kinase domain of ALK and EML4 is fused together. The ALK kinase thus is under the control of EML4, which results in a constant activation of the kinase. ALK similar to EGFR stimulates proliferation and inhibits apoptosis [85]. Patients with this inversion respond excellently to crizotinib treatment, which is now the second example of targeted therapy in NSCLC [86]. Proof of EML4ALK1 inversion can be done with different methods: the most common is FISH where two probes (3′ and 5′) detecting the ALK gene on both sides of the breakpoint are used. In normal situation, this probes will detect the two portions close together or overlapping within the tumor nucleus. In cases of rearrangement, the probes will highlight each of the splitted portions of the ALK1 gene, so instead of two overlapping signals the signals split apart. In the Caucasian population, EML4ALK1 rearrangement is usually found in 4–6 % of NSCLC; in adenocarcinomas, this might be increased to 8 %.
Other genes joining the ALK1 gene in the same way can replace the EML4 gene. If KIF5B joins to ALK1, the overexpression of KIF5B-ALK [45] in mammalian cells led to the activation of signal transducer and activator of transcription 3 (STAT3) and protein kinase B and enhanced cell proliferation, migration, and invasion [45]. Another fusion partner recently described is ALK-KLC1 [87]. These other ALK1 fusions are rare; the incidence is about 1 %.
19.3.4 ROS1
ROS1 is another kinase involved as a driver gene in adenocarcinomas of the lung [92]. Usually the rearrangement of ROS1 is evaluated by two FISH probes for the 3′- and the 5′ ends. Only few fusion partners have been identified so far, CD74, SLC34A2, EZR, and GOPC/FIG [93–95]. This gene rearrangement has no influence on outcome, but similar to ALK1, this is usually a younger population of cancer patients [96]. The incidence of ROS1 rearrangement is in the range of 1 %. The function of one of the fusion genes EZR-ROS was studied in a mouse model and showed that in this experimental setting the fusion gene acted as an oncogene inducing multiple tumor nodules in mice [95]. Most important patients with this type of gene aberrations responded well to the ALK1 inhibitor crizotinib [97–99].
19.3.5 KIF5B and RET
KIF5B is one of the fusion partners for either ALK1 or RET. The KIF5B-RET fusion gene is caused by a pericentric inversion of 10p11.22-q11.21. This fusion gene overexpresses chimeric RET receptor tyrosine kinase, which can spontaneously induce cellular transformation [100]. Besides KIF5B, CCDC6, and NCOA4 can form fusion genes with RET. Patients with lung adenocarcinomas with RET fusion gene had more poorly differentiated tumors, are younger, and are more often never-smokers. Solid adenocarcinomas predominate, tumors are smaller, but lymph node incidence is higher. The incidence of RET fusion is about in 1 % of NSCLCs and almost 2 % of adenocarcinomas [100–102].
19.3.6 MET
MET is another receptor tyrosine kinase bound to cell membranes in NSCLC. The ligand for MET is HGF, originally found in hepatic carcinomas. This receptor came into consideration in NSCLC because amplification of MET or alternatively upregulation of HGF was identified as a mechanism of the resistance in EGFR-mutated adenocarcinomas [43, 103]. A search for the role of MET in other NSCLCs excluding EGFR-mutated adenocarcinomas showed that MET amplification was rare, but upregulation of MET is a common event: approximately 20 % of NSCLC including adenocarcinomas and squamous cell carcinomas showed high protein expression, but only 2 % MET amplification (Popper et al. in preparation). Clinical studies are in progress to evaluate the possibility to interfere with MET signaling using monoclonal antibodies. Other studies use small molecule inhibitors for MET. Since MET expression is common in EGFR-mutated adenocarcinomas, these studies aim to inhibit both EGFR and MET signaling pathways [104–106].
19.3.7 Others Genes
Histone acetylases and deacetylases (HAT, HDAC) regulate the access of the DNA by methylation and demethylation. Thus, these enzymes are important for DNA silencing [107–109]. In addition heavily methylated DNA tends to switch into a supercoiled form, which cannot be read by the transcription machinery. Histones themselves are in addition important for the correct positioning of the DNA, fixing the DNA to specific areas of the nuclear membrane [110–113]. Attempts to interfere with this system have been made quite a while ago, but the results were not convincing, probably because there are several types of HATs and HDACs with different functions. Recently treatment with HDAC inhibitors in combination with other drugs has shown promising results, resulting in apoptosis and tumor cell necrosis [114–116].
In a study focusing on molecular alterations in pulmonary adenocarcinomas, many additional genes were identified: well known are losses of one allele of the tumor suppressor PTEN in 9 %, often associated with upregulation of PIK3CA; however, PI3KCA mutations were also detected in 5 %. Two other genes mutated in 3 % and 2 % were identified as STK11 and BRAF, respectively [117]. Interestingly these gene alterations could be sorted by smoking habits to either smokers (STK11) or nonsmokers (PIK3CA). In addition squamous cell carcinoma morphology was associated with PTEN, STK11, and PIK3CA [117].
In a study analyzing Korean lung cancer patients by transcriptome sequencing, the authors identified the well-known candidates, EGFR, KRAS, NRAS, BRAF, PIK3CA, MET, and CTNNB1, but also new driver mutations such as LMTK2, ARID1A, NOTCH2, and SMARCA4 [65]. Besides these mutated genes, also fusion genes were detected as ALK, RET, and ROS1 and new ones as FGFR2, AXL, and PDGFRA. We also found an association between lymph node metastasis and somatic mutations in TP53 (mutations of TP53 will be discussed later on, since this is found in several lung cancer types).
Elevated levels of insulin-like growth factor (IGF)-II are associated with a poor prognosis in human pulmonary adenocarcinoma. Moorehead et al. succeeded to establish pulmonary adenocarcinoma in mice by transgenic overexpression of IGF-II in lung epithelium. These tumors expressed TTF-1, SP-B, and proSP-C. Activation of IGF-IR resulted in the downstream activation of either the Erk1/Erk2 or p38 MAPK pathways [118].
Within this IGF/IGFR system, also binding protein plays a prominent role: IGFBP3 expression resulted in upregulation of VEGF and HIF1, pointing to an important fact of neoangiogenesis, important for accelerated growth and invasion [119]. In contrast IGFBP1 seems to act like a tumor suppressor decreasing colony formation of cell cultures and increasing apoptosis. IGFBP1 has been found methylated in pulmonary adenocarcinomas [120]. The IGFR1 pathway is also involved in resistance mechanisms in EGFR-mutated adenocarcinomas [121]. Treatment with monoclonal antibodies for IGFR1 is also in clinical studies [122–124].
19.4 Squamous Cell Carcinomas
Squamous cell carcinoma (SCC) is defined by a platelike layering of cells, keratinization of at least single cells, intercellular gaps and bridges (represented by desmosomes and hemidesmosomes), and positive staining for high molecular weight cytokeratins (CK 3/5, 13/14). There are some morphologic variants as small cell and basaloid, but these have not been associated with gene signatures and therefore are only important in diagnostics.
The incidence of SCC has dropped in the last three decades from a major entity representing 35 % of lung carcinomas to around 17 %. One of the major reasons is the shift from filter-less to filter cigarettes. This has resulted in the reduction of particle-bound carcinogens and increase of vaporized carcinogens, which more easily reach the bronchioloalveolar terminal unit, inducing mainly adenocarcinomas.
In the past, SCC was mainly a diagnosis required to exclude several therapeutic options in the clinic: no pemetrexed therapy, no anti-angiogenic drugs, and less responsiveness to cisplatin treatment. However, this has changed within the last 3 years.
19.4.1 FGFR1
Fibroblast growth factor receptor 1 was identified being amplified in about 20 % of squamous cell carcinomas [125] (and personal unpublished data). In experimental studies as well as in ongoing clinical trials, it was found that only amplification, proven by in situ hybridization methods, identified patients, which respond to small molecule inhibitor treatment [126, 127] (and unpublished communication from R. Thomas, Cologne, Germany).
19.4.2 DDR2 and FGFR2
19.4.3 SOX2 Amplification
SOX2 gene located on chromosome 3q26.3 is a factor for the maintenance of stem cell-like properties in lung cancer cells [131]. SOX2 amplification has been reported to be specifically associated with SCC morphology [132–134]. However, other investigations have claimed also an importance for small cell carcinomas and adenocarcinomas [135, 136]. Amplification in SCC was associated with better prognosis [137] whereas with poor prognosis in adenocarcinomas and SCLC [135, 138]. So far no specific therapies do exist for patients with this genetic abnormality.
19.4.4 PTEN Mutation-Deletion
PTEN deletions are quite common in NSCLC, usually associated with the subsequent upregulation of PI3K and downstream activation of the AKT pathway [62, 139]. In the past, therapies were conducted with inhibitors of mTOR, but failed due to the subsequent upregulation of a negative feedback loop of mTOR, which in turn activates AKT and this time results in a circumvention of mTOR via other pathways [140–142]. Recent work, however, has shown a benefit of mTOR inhibition, when applied in the right context [143, 144]. PTEN mutation is more rare, but can be found more often in squamous cell carcinomas [117]. If patients with this genetic abnormality can also be treated by a combined modality is still an unsolved issue.
19.4.5 PDGFRA Amplification
19.4.6 CDKN2A (p16) Mutation, Deletion, and Methylation
Another uncommon genetic modification is found in the CDKN2A gene coding for the p16 protein. P16 is regarded as a tumor suppressor protein and is involved in cell cycle regulation in many pulmonary carcinomas including SCLC, SCC, and adenocarcinoma [148–150]. It closely interacts with Rb and p53 protein.
19.4.7 Notch1 Mutation
NOTCH-1 regulates cell specification and homeostasis of stem cell compartments, and it is counteracted by the cell fate determinant Numb. Notch signaling is altered in approximately one third of NSCLCs. Loss of Numb expression results in increased Notch activity; in a smaller fraction of cases, gain-of-function mutations of the NOTCH-1 gene are present. Inhibitors of Notch can selectively kill epithelial cell cultures harboring constitutive activation of the Notch pathway [151]. In a subsequent study, NOTCH-1 and NOTCH-2 frame shift and nonsense mutations were identified in pulmonary squamous cell carcinomas [152]. More importantly NOTCH-1 has an important role in carcinogenesis by suppressing p53-mediated apoptosis and regulating the stability of p53 protein. NOTCH-1 also plays an important role in KRAS-induced mouse adenocarcinoma models [153].
19.4.8 REL Amplification
Inducing lung adenocarcinoma in a mouse model, it was shown that different downstream activation of RAS pathways is necessary to induce an invasive and more important angioinvasive phenotype. Only the combined activation of the PI3K, RAS-MAPK-ERK, and the RAL and REL pathway could induce an aggressive phenotype [78]. Whereas REL-A seems to be exclusively expressed in SCLC, REL-B was shown to be processed in NSCLC cell lines. REL-B was shown to suppress the expression of ß1-integrin and thus prevented adherence of the carcinoma cell [154].
19.5 Large Cell Carcinoma
Large cell carcinoma (LC) is defined by large cells (>25 mμ) devoid of any cytoplasmic differentiation and large vesicular nuclei. They have a well-ordered solid structure. By electron microscopy, differentiation structures can be seen such as hemidesmosomes, tight junctions, intracytoplasmic vacuoles with microvilli, and ill-formed cilia. This fits clearly into the concept of a carcinoma, at the doorstep of adenocarcinoma and squamous cell carcinoma differentiation. LC numbers have dramatically decreased due to the use of immunohistochemistry for differentiation. Using TTF1 low molecular cytokeratins as well as p63 and cytokeratin 5/6, most cases of LC were either shifted into adenocarcinoma or squamous cell carcinoma, respectively [155]. These recent changes make an evaluation of genetic aberrations in LC quite difficult, since genetic studies were based on previous classifications.
Not surprisingly EGFR mutations, MET amplifications, and EML4ALK1 fusions have been reported in LCC [156, 157]. LKB1 a gene mutated in a small percentage of adenocarcinomas was also shown in squamous and large cell carcinomas [158]. LKB1, also known as STK11, is involved in the negative regulation of mTOR and closely cooperates with TSC1 and TSC2 genes [159].
19.6 Other Types of Large Cell Carcinomas
In contrast to LC, which is negatively defined by exclusion criteria, these are positively defined.
LC with rhabdoid phenotype is characterized by a solid growth pattern, often overlaid by a reactive proliferation of pneumocytes, which can give these tumors a pseudoalveolar pattern and a pseudo-composition of two cell populations. Within the cytoplasm of the tumor cells, eosinophilic inclusion bodies can be found, similar to those seen in rhabdomyosarcomas. These inclusion bodies are stained by eosin and are negative for striated muscle markers, but positive for vimentin. The production of vimentin filaments, which seem to have no function because of package into a cytoplasmic vacuole, is the only known abnormality for this tumor type. In a single study, KRAS mutations were found in some cases of this tumor type [155].
Sheets of undifferentiated tumor cells embedded in a lymphocyte-rich stroma characterize lymphoepithelial–like LC. The carcinoma cells are positive for cytokeratins 13/14; the lymphocytes in most cases are B cells. In cases from Southeast Asia, most lymphoepithelial-like LCs are positive for EBV, and EBV seems to play a role in carcinogenesis, whereas in Caucasians, these carcinomas are negative for EBV [160, 161]. Only one study looked up genetic changes in this tumor entity: they found unusual mutations in TP53 at exon 8 and EGFR mutations in few patients within their large series. These were exon 21 (not L858R) and exon 18 and 20 mutations [162].
Pulmonary clear cell carcinoma is another rare variant of LCC, defined by abundant glycogen in the cytoplasm of the tumor cells. Tissue processing usually dissolves glycogen, thus leaving the impression of a clear/empty cytoplasm. Only one study examined molecular changes in this tumor type and found predominantly KRAS mutations [155].
19.7 The Neuroendocrine Carcinomas
19.7.1 Small Cell Neuroendocrine Carcinoma
Small cell carcinoma is defined by nuclear size of 16–23 mμ (not so small!), dark stained nuclei (mainly composed of heterochromatin), inconspicuous or lacking nucleoli, small cytoplasmic rim, often invisible in light microscopy, and fragile nuclei.
SCLC is regularly positive for the neuroendocrine markers NCAM and synaptophysin, but most often negative for chromogranin A. The best marker is NCAM with a strong membranous staining. SCLC is positive for low molecular weight cytokeratins. SCLC produces hormones, such as adrenocorticotropin (ACTH), but also substances interfering with the blood coagulation system. In contrast to carcinoids, SCLC more often is positive for heterotopic hormones (i.e., hormones usually not found in adult lung). In our experience, a positive reaction for gastrin-releasing peptide (GRP) and ACTH is most often seen. The secretion of ACTH can cause Cushing syndrome. Some of the hormones especially GRP act as an autocrine loop: the peptide is produced by the cancer cells, is released, and binds back to their respective membrane-bound receptors, which themselves signal back into the nucleus with a growth stimulation [163].
Genetic abnormalities in SCLC are quite common; usually over 50 % of the SCLC chromosomes are affected [164–166]. These many genomic alterations made a search for driver gene mutations/alterations complicated, which consequently resulted that besides classical chemotherapy, no targeted therapy emerged yet.
However, some genetic alterations are known for a long time, not resulting in a therapeutic intervention strategy. Two genetic alterations have been long known, RB loss or mutation and TP53 mutation. Since both genes are involved in cell cycle checkpoint controls, this might explain the high numbers of genetic alterations [167–169]. Other genes involved in SCLC are the tumor suppressor FHIT and RASSF1, both on chromosome 3p, RARbeta, and Myc genes [169]. RASSF1 mRNA expression was lost in all SCLC cell lines tested, whereas its promoter was methylated in some NSCLC cell lines [170]. In SCLC also apoptotic and immunogenic mechanisms seem to be inactivated. In a study by Senderowicz, FasL was overexpressed in almost all SCLC cases examined. The ratio of Fas/FasL was decreased. The authors concluded that FasL overexpression in the context of Fas downregulation might allow tumor cells to induce paracrine killing of cytotoxic T cells [171]. Since the PI3K-Akt signaling pathway is activated in almost all cases of SCLC, this system might also be associated with inhibition of apoptosis via upregulation of TNFRSF4, DAD1, BCL2L1, and BCL2L2 and with chemoresistance [172, 173]. These data demonstrate that several systems are involved in SCLC growth, survival, and resistance to chemotherapy.
ASH1 was identified as the gene responsible for the neuroendocrine phenotype in both high-grade carcinomas [174]. Together with other genes (ATOH1, NEUROD1, and NEUROD4) involved in neurogenic differentiation, they are also expressed in NSCLC with neuroendocrine phenotype. As SCLC, these NSCLC cases expressed mRNA for dopa decarboxylase and stained positively for neuroendocrine markers [175]. ASH1 seems to be an early differentiation gene in the developing lung. In embryonic development, ASH1 was found in neuroepithelial bodies and solitary neuroendocrine cells, but vanishes with maturation of the lung. Therefore, ASH1 might be an early program for neuroendocrine cell differentiation [176]. If there is another function of ASH1 is not entirely clear. ASH1 seems to repress tumor suppressor such as DKK1 and 3, which are regulators of the Wnt-βcatenin pathway. ASH1 also inactivates E-cadherin and integrinß1 by de-acetylation and methylation of the DKK1 and E-cadherin promoters [177]. In an animal lung cancer model, the expression of ASH1 enhances the carcinogenic effect of SV40 large T antigen, suggesting that ASH1 might cooperate with pRB [178].
19.7.2 Large Cell Neuroendocrine Carcinoma
Large cell neuroendocrine carcinoma is defined by a neuroendocrine pattern, i.e., rosettes and trabecules. On low power, they look similar to carcinoids, but on higher magnification, abundant mitoses are obvious. The prognosis of LCNEC is similar to that of SCLC; both are grouped as the high-grade neuroendocrine carcinomas.
Genetic analysis of LCNEC showed similar alterations as found in SCLC. However, allelic losses at 5q and abnormalities in the p16 gene may differentiate LCNEC from SCLC [179]. Another difference between both high-grade neuroendocrine carcinomas is seen at chromosome 3q: gains of 3q are frequently seen in SCLC, whereas gains of 3q were absent in LCNEC. However, gains of 6p were frequent in LCNEC; deletions within 10q, 16q, and 17p were more common in SCLC [180]. ASH1 mRNA is found higher in SCLC, whereas its counteracting gene HES1 was more frequently expressed in LCNEC [181].
19.7.3 Carcinoids
Typical carcinoid is defined by neuroendocrine structures, such as rosettes, trabecules, and solid nests, 0 or 1 mitosis per 2 mm2, and absence of necrosis. Atypical carcinoid is defined by two to ten mitoses per 2 mm2 and/or presence of necrosis and again neuroendocrine structures. In both carcinoids, there is an invasive growth into the lung, and lymphatic and blood vessel invasion can be found in some cases. Some carcinoids can metastasize, but so far there are no predictive markers for the biological behavior.
Those carcinoids, which have more than two losses on distal chromosome 11q, and those with multiple chromosomal losses (<10), also show a worse outcome. Based on genetic studies, it can be speculated that the 140 kDa isoform precursor of NCAM, Zinc-finger protein-like 1, and sorting Nexin 15 might be involved in the genesis of carcinoids [182].
19.8 Salivary Gland Type Carcinomas
Salivary gland tumors can occur in the lung, usually in a central location. Within the spectrum are mucoepidermoid carcinomas, adenoid cystic carcinomas, and epithelial-myoepithelial carcinomas. These are rare carcinomas with a wide range of affected ages, from children as early as 3 years of age, as well as in old patients.
19.8.1 Mucoepidermoid Carcinoma
Due to their central location, they may grow as a polypoid mass occluding large bronchi and thus causing obstruction – this is often the cause for the main clinical symptoms (poststenotic bronchopneumonia).
In low-grade carcinomas, cystic and solid areas are found. In both, mucin-producing columnar cells form glands, tubules, and cysts. Within the glandular areas, squamous epithelium is interspersed; the tumor cells are usually non-keratinizing. In high-grade carcinomas, the distinction from adenosquamous carcinoma might sometimes be impossible, due to overlapping features. The proof is the mixture of squamous and mucin-producing columnar cells within a gland, endobronchial growth, and absence of keratin pearls and an in situ component. The prognosis of high-grade mucoepidermoid carcinomas is similar to other NSCLCs.
EGFR was studied in mucoepidermoid carcinomas of the lung. Protein expression was frequently found, whereas mutations in exon 18–21 were absent. Polysomy of the EGF receptor was seen in a small percentage of cases [183]. In another study, the authors reported EGFR mutations and also a response to tyrosine kinase inhibitor treatment. One of the patients in this study showed a response to treatment without alterations of the EGFR [184]. A third study probably presents the explanation for this behavior: the authors showed a translocation t(11;19) resulting in a fusion oncogene CRTC1-MAML2. CRTC1-MAML2 was shown to upregulate the EGFR ligand, amphiregulin, and thus activate the receptor [185].
19.8.2 Adenoid Cystic Carcinoma
Similar to the salivary counterpart, adenoid cystic carcinoma of the bronchus is a slowly growing tumor with late lymph node and distant organ involvement. However, recurrence is frequent. They occur in the trachea and the large bronchi as far as normal bronchial glands are found. The tumor forms pseudotubules filled with mucin-like material, which is composed of matrix proteins of the basal lamina as collagen type 4 and fibronectin.
In an experimental model of adenoid cystic carcinoma, it was shown that dual inhibition of EGFR and VEGFR2 resulted in apoptosis, decreased angiogenesis, and metastasis [186]. In an array-CGH study, a gain was found on chromosome 4q12, which contains a cluster of receptor tyrosine kinases such as KIT, PDGFRA [152]; however, in another study looking up this area, KIT mutations or amplifications were not found [187]. Also mutations of EGFR could not be demonstrated in adenoid cystic carcinomas, but upregulation of the protein was a constant feature [183, 188]. In another study, experimental inhibition of phospho-ERK1/2 resulted in inhibition of tumor cell growth and metastasis [189], and in a clinical study, growth of adenoid cystic carcinoma could be inhibited by a multikinase inhibitor [190]; however, in a subsequent clinical trial, this inhibition could not be confirmed [191]. Recently new investigations came up with genetic aberrations in these carcinomas. The MYB-NFIB fusion resulting in overexpression of MYB was found in the majority of these carcinomas [192, 193]. Copy number alterations including downregulated suppressor genes were in addition associated with behavior of these carcinomas [192]. Newly identified mutations in adenoid cystic carcinomas, some of which might lead to a targeted therapy, are FGF-IGF-PI3K mutations and mutations in NOTCH-1/NOTCH-2 signaling regulator SPEN, which might be a new cancer gene in this type of tumors, and finally within a subset of these carcinomas also FGFR2 mutations [193, 194].
Another rare salivary gland tumor is acinar cell carcinoma. Uniform tumor cells showing acidophilic/azurophilic granules in their cytoplasm characterize it. So far no specific genetic abnormality has been reported in pulmonary variants.
19.9 Sarcomatoid Carcinomas (SC)
The sarcomatoid carcinomas are a group of carcinomas with sarcomatoid features. This group is composed of pleomorphic carcinoma, spindle and giant cell carcinoma, pulmonary blastoma, and carcinosarcoma.
Pleomorphic carcinoma is defined as a carcinoma with either a spindle or giant cell component and any other NSCLC component. Pulmonary blastoma is composed of a fetal-type adenocarcinoma and an embryonic type of stroma. Most often this stroma component is benign; however, in rare cases, the stroma can exhibit atypia and even malignancy. Finally carcinosarcoma is composed of any type of NSCLC with a sarcoma with osseous, rhabdomyoblastic, or chondroblastic differentiation.
Few studies have focused on this tumor types. In the study by Pelosi, the epithelial components of SC showed expression of epithelial markers and cell cycle inhibitors p21Waf1 and p27Kip1 and the loss of FHIT, whereas the sarcomatoid components expressed mesenchymal markers and differently reacted for molecules involved in cell differentiation, cell cycle control, and tumor cell growth and motility [195]. In another study, the mechanisms of epithelial-mesenchymal transition were explored. The classical pathways NOTCH-1 and Snail and the Wnt pathway could be excluded, but a possible alternative pathway including Jun-Vimentin-Fascin was found [196]. Looking up EGFR and KRAS mutations in SC, no mutations were found for EGFR, but KRAS in almost half of the cases. However, EGFR was constantly overexpressed at the protein level, which might suggest the possibility of an EGF antibody treatment [197].
19.10 Preneoplastic Lesions
Actually our knowledge is very small on preneoplastic lung lesions: for a few carcinomas, the preneoplastic lesion is known; for most carcinomas discussed above, we know nothing. And most important even for those well-known lesions, we cannot predict if these lesions will progress into carcinoma or not. The factors responsible for progression and invasion are unknown. Research on preneoplastic lesions faces two major problems:
When the neoplastic process starts? And what to analyze?
From our current understanding, the neoplastic transformation has some important requirements, which usually precede morphologic changes: nutrition, nucleotides for DNA replication, and oxygen for metabolism. Preneoplastic cells need increased access to nutrients for building up an energy reserve for the increase of cell divisions, they need to get access to higher levels of purine and pyrimidine bases to facilitate their increased DNA replications during mitosis, and for an increased metabolism, also an increase of oxygen might be required [10, 198, 199]. Increased oxygen consumption, however, is controversially discussed: hypoxia seems to be critical for invasion and metastasis, so probably increased oxygen and oxidative metabolism might be required only in the early intraepithelial expansion of the preneoplastic cells, whereas glycolysis seems more common in invasive carcinomas [198, 200, 201].
19.10.1 Hyperplasia of Goblet Cells and Squamous Metaplasia/Dysplasia
For squamous cell carcinomas, the preneoplastic lesion is known since a while. In the large bronchi, there exists a protection program for toxin/carcinogen exposure, which starts with goblet cell hyperplasia and proceeds to squamous cell metaplasia and further on to squamous cell dysplasia or intraepithelial neoplasia. A vascular variant of dysplasia has been described characterized by an ingrowth of capillaries into the squamous epithelium. Atypia and mitosis might be mild or absent, respectively; however, this represents a rapid progressive lesions [11] most probably due to the expression and release of VEGF (unpublished personal observation).
A few factors influencing this progression have been identified, such as a p63 splice variant; amplification of PI3KCA; increased NFκB p65 nuclear expression; gradual increases of caspase-8, STAT 5, and p70s6K; or decrease of E-cadherin [202–205]. Angiogenic stimuli seem to play a role in progression, since it is upregulated in rapid progressive squamous cell dysplasia with prominent vascular intraepithelial growth pattern [11]. However, the interrelation of genes upregulated and downregulated has not been elucidated, so we are presently limited to single studies looking for different genetic abnormalities.
Atypical adenomatous hyperplasia and bronchiolar columnar cell dysplasia are two preneoplastic lesions confined to the alveolar and bronchiolar periphery, respectively. Atypical alveolar hyperplasia is visible already at low power magnification. The normal epithelium is replaced by pneumocyte type II-like atypical cells. Between the cells, lined up as a single row, there are usually gaps left. When these gaps are lost, and cell pile up close to each other, or form cell papillae without stroma, this already fulfills the criteria of adenocarcinoma in situ (AIS). AAH is regarded as the precursor for some non-mucinous adenocarcinomas, especially acinar and papillary types.
19.10.2 Genetic Aberrations in AAH
Genomic aberrations were found to increase from AAH to AIS and invasive adenocarcinoma; the chromosomes involved in the AAH lesions were still present in the high-grade lesions, suggesting an AAH to AIS to invasive adenocarcinoma sequence [206, 207]. KRAS mutations were found in one third of AAHs studied and seem to be an early event in carcinogenesis [208, 209]. A loss of either TSC1 or TSC2 genes was another finding in well-differentiated adenocarcinomas and their associated AAH lesions [210, 211]. EGFR mutations as well as amplifications could not be detected in AAH, but were found in adjacent well-differentiated adenocarcinomas and less frequently in less-differentiated ones [212, 213]. In another study comparing KRAS and EGFR mutations in AAH, AIS, and invasive adenocarcinomas, the percentage of KRAS mutations decreased from AAH to AIS to invasive adenocarcinomas, whereas EGFR mutations increased from AAH to AIS and to invasive adenocarcinomas. This study concluded that two different pathways of AAH exist, one driven by KRAS and the other by EGFR mutations, respectively [214].
In contrast to AAH, bronchiolar columnar cell dysplasia (BCCD) can only be identified at high magnification. In BCCD atypical cells gradually replace normal bronchiolar epithelium. In early stages of BCCD, a monomorphous proliferation of cells replaces these differentiated cells and gradually expands up to the surface. If completely replaced, more atypia is recognized.
In a study of genetic aberrations, Ullmann found an increase of genetic alterations from 2.6 in BCCD to 14.7 in the concomitant adenocarcinoma. Unbalanced numerical aberrations were losses of 3p, 9, 13, and 14 and gains of 1q, 17, 19q, and 20q [215]. Another study demonstrated loss of P16INK4a protein in 70 % of cases. P53 accumulation was found in 26 % of the cases [216].
Atypical goblet cell hyperplasia (AGCH) is much more difficult to recognize: the nuclei are compressed at the cell border, and the chromatin structure is invisible. Atypical, signet ringlike cells are replacing the normal epithelium resulting in a monotonous pattern. Atypical goblet cell hyperplasia might give rise to the different mucinous adenocarcinomas of the lung. AGCH was studied in cystic pulmonary adenomatous malformation (CPAM). In this study, chromosomal gains on chromosomes 2 and 4 were found as the major genetic alteration [217]. The same genetic alterations were demonstrated in three associated adenocarcinomas of children. So most probably oncogenes located on these chromosomes are involved in carcinogenesis.
19.10.3 Neuroendocrine Cell Hyperplasia
Neuroendocrine cell hyperplasia is divided into NE hyperplasia associated with fibrosis and bronchiectasis or in the vicinity of a carcinoid and diffuse NE hyperplasia without known cause; in addition a nodular proliferation is described, and finally there is tumorlet, which is different from carcinoid by size and separation of tumor cell groups by fibrous bands. Tumorlets and diffuse neuroendocrine hyperplasia are often found in the vicinity of carcinoids, and therefore it is speculated that they may represent preneoplastic lesions for carcinoids. A few studies focused on molecular changes in these lesions. Since neuroendocrine lesions and low-grade tumors are usually rich in capillaries, one study focused on angiogenetic factors. VEGF was found highly expressed in diffuse proliferations, but there were no correlations with progression, but rather an association with fibrosis [218]. N-cadherin was found in a high proportion of diffuse hyperplasia and carcinoids, but less in SCLC and LCNEC. In carcinoids N-cadherin expression was associated with negative lymph node status [219].
19.11 Selected Examples of Benign and Mesenchymal Lung Tumors
19.11.1 Benign Epithelial Tumors
Papillomas posses a stroma with thin-walled and fragile capillaries and veins, branching in a treelike manner, accompanied by few fibrocytes, and covered by metaplastic epithelium. The epithelium is most often composed of squamous cells, sometimes with certain degrees of dysplasia. In rare cases, a transitional and a columnar epithelium or mixtures of all three can occur. In squamous cell papilloma, koilocytes are seen, and by immunohistochemistry, in situ hybridization, or PCR, human papillomavirus can be detected [220, 221]. A bizarre outline of nuclei is usually detected in cases of HPV 16- or 18-positive cases. Many HPV types are known; in general they are divided into oncogenic and non-oncogenic types. They all have in common to promote growth of the epithelial cells when integrated into the cell genome. Also recurrence of papillomas is common. The most important function of HPV genome is its interference with the cell cycle control proteins p53 and RB protein. Specific parts of the HPV genome induce stabilization of the p53 and RB proteins preventing apoptosis induced by genetic aberrations. Therefore, the DNA repair machinery is ineffective, and mitosis progresses despite DNA defects and gene aberrations [222, 223]. Integration of oncogenic HPV types (16, 18) will induce a rapid progression of the papilloma into invasive squamous cell carcinoma [221, 224].
19.11.2 Sclerosing Pneumocytoma
Sclerosing pneumocytoma is a benign epithelial tumor. This is an epithelial neoplasm composed of immature and mature pneumocytes. A few genetic abnormalities have been described in sclerosing pneumocytoma. Aberrant nuclear and cytoplasmic expression of beta-catenin was found in both cellular elements of sclerosing pneumocytoma [225]. Overexpression of estrogen receptor beta was seen in almost 50 % of these tumors in another study [226]. One study looking for EGFR, HER2, and KRAS abnormalities did not reveal molecular alterations; however, allelic losses of p16 and Rb were observed [227].
19.12 Tumors Induced by Mutations of the TSC Genes (Related to Tuberous Sclerosis)
TSC genes code for the two proteins hamartin (TSC1) and tuberin (TSC2). Both are embedded into a signaling chain involving LKB1 (STK11), AMPKα, and both upstream of TSC and Rheb and mTOR and downstream of TSC1/TSC2. Normally LKB1 and cyclic AMP stimulate AMPKα, which in turn stimulates TSC1 and TSC2. The translated proteins hamartin and tuberin form a dimer and inhibit Rheb, which stimulates mTOR and mTORC formation. mTOR in sequence can either form the mTORC1 complex (with GβL and raptor) or the mTORC2 complex (with GβL and rictor) [228, 229]. Both TORC complexes signal into different downstream cascades, but altogether induce proliferation and influence cytoskeleton formation in cells and many more functions. In tuberous sclerosis, one of the genes is mutated and no functional protein is translated. This results in uncontrolled mTOR upregulation and tumor formation.
19.12.1 Multifocal Nodular Pneumocyte Hyperplasia (MNPH)
MNPH is characterized by an adenoma-like proliferation of immature and mature pneumocyte type II-like cells without atypia. The cells are positive for surfactant apoprotein A. These lesions form multiple micronodules. There is no progression into malignant tumors known and even no progression of these lesions into larger tumors [230]. Mutation of the TSC1 and TSC2 genes has been proven, so these lesions represent an adenomatous proliferation, induced by genetic instability, caused by mutations of TSC genes [231, 232].
19.12.2 Lymphangioleiomyomatosis (LAM)
LAM is characterized by an uncontrolled proliferation of mature and immature smooth muscle cells. The muscular proliferation is confined to bronchioles and alveoli and also occurs subpleurally and in the vicinity of vascular walls. By compression of lymphatics and airways, cystic spaces are formed, some are dilated lymphatics, and others are dilated bronchioles and alveoli [233]. The disease predominantly affects young woman and often starts after the first delivery. LAM can recur after lung transplantation and therefore present with some features of a systemic malignant disease (repopulation of transplanted lungs from outside) [234–237].
Genetically LAM is related to the tuberous sclerosis complex. Mutations of the TSC1 and TSC2 have been demonstrated in almost all cases evaluated. LAM is one of the many “benign” tumor lesions associated with somatic mutations found in this disease characterized by genetic instability [238–240]. Therapeutic approaches have been invented, first by an antiestrogen therapy including oophorectomy. Despite recurrences reported, lung transplantation has emerged as the best treatment. Recently a treatment with a mTOR inhibitor has shown promising results [241, 242].
19.12.3 Clear Cell Tumor (Sugar Tumor, PEComa = Perivascular Epithelioid Cell Tumor)
PEComa is composed of large polygonal tumor cells with inconspicuous nuclei and usually invisible nucleoli. On formalin-fixed paraffin-embedded material, the cytoplasm is clear. By PAS stain, abundant glycogen is seen. Another characteristic feature is the prominent vascular network. Most of these tumors will behave benign. However, there is a rare malignant variant with marked nuclear atypia [243, 244]. PEComas are part of the spectrum of the tuberous sclerosis complex. It can be associated with angiomyolipoma of the kidney and LAM and carries a similar spectrum of mutations [245, 246]. Recently another genetic abnormality was demonstrated in a subset of PEComas without tuberous sclerosis association, namely, a TFE gene rearrangement, resulting in an upregulation of the TFE3 protein [247].
19.13 Malignant Tumors of Childhood
19.13.1 Pleuropulmonary Blastoma
Pleuropulmonary blastoma (PPB) is a malignant mesenchymal tumor, which can arise in the pleura, the lung, or both. It can present as a predominant cystic, a mixed cystic and solid, or a pure solid lesion, which is also reflected by an increased aggressive behavior. The tumor cells form layers of immature small cells with relatively large nuclei with dense chromatin. These cells form a so-called germinal layer. In addition interspersed giant cells with rhabdomyoblast differentiation and areas of chondrosarcoma are usually seen [248, 249].
Genetically gains of large parts or whole chromosome 8 are often found in PPB [250–252]. A few other aberrations have been reported: losses on 6q13-qter, 10pter-p13, 10q22-qter, and 20p13 and gains of chromosomes 2 and 8p11-p12 [253]. In some reports, mutations of the TP53 gene were described [254, 255]. Recently DICER 1 syndrome has been linked to PPB: Dicer is a protein involved in processing of small inhibitory microRNAs. A mutation has been reported in several families, and this was linked to the development of PPB and other childhood tumors [256].
19.13.2 Congenital Myofibroblastic Tumor
Congenital myofibroblastic tumor is another rare childhood tumor composed of proliferating myofibroblastic cells, sometimes with marked cellularity and mitotic activity. The tumor cells proliferate within the interstitium and peribronchiolar and thus cause bronchiolar obstruction. They can behave biologically like malignant tumors [257, 258]. A single genetic analysis was reported, under the other name of the tumor, namely, leiomyosarcoma of childhood. The authors reported gains of chromosomes 2 and 11 and losses of chromosomes 9, 19, 20, and 22 [259].
19.14 Final Remarks
Due to the vast amount of knowledge in the epidemiology of lung cancer, we did not include these aspects. Several other topics, which are more general and not organ based in the development of cancer, are not discussed herein, such as the stem cell theory in cancer development and metastasis. Another aspect is invasion and metastasis of lung cancer. This is discussed in Chap. 20 on metastasis.
References
1.
Langer CJ, Natale RB. The emerging role of vascular endothelial growth factor receptor tyrosine kinase inhibitors. Semin Oncol. 2005;32:S23–9.PubMed
2.
Mae M, O’Connor TP, Crystal RG. Gene transfer of the vascular endothelial growth factor receptor flt-1 suppresses pulmonary metastasis associated with lung growth. Am J Respir Cell Mol Biol. 2005;33:629–35.PubMed
3.
Samadikuchaksaraei A, Cohen S, Isaac K, Rippon HJ, Polak JM, Bielby RC, Bishop AE. Derivation of distal airway epithelium from human embryonic stem cells. Tissue Eng. 2006;12:867–75.PubMed
4.
Kotton DN, Fabian AJ, Mulligan RC. Failure of bone marrow to reconstitute lung epithelium. Am J Respir Cell Mol Biol. 2005;33:328–34.PubMedPubMedCentral
5.
Kumar RK, Li W, O’Grady R. Maintenance of differentiated phenotype by mouse type 2 pneumocytes in serum-free primary culture. Exp Lung Res. 1995;21:79–94.PubMed
6.
Decaussin M, Sartelet H, Robert C, Moro D, Claraz C, Brambilla C, Brambilla E. Expression of vascular endothelial growth factor (VEGF) and its two receptors (VEGF-R1-Flt1 and VEGF-R2-Flk1/KDR) in non-small cell lung carcinomas (NSCLCs): correlation with angiogenesis and survival. J Pathol. 1999;188:369–77.PubMed
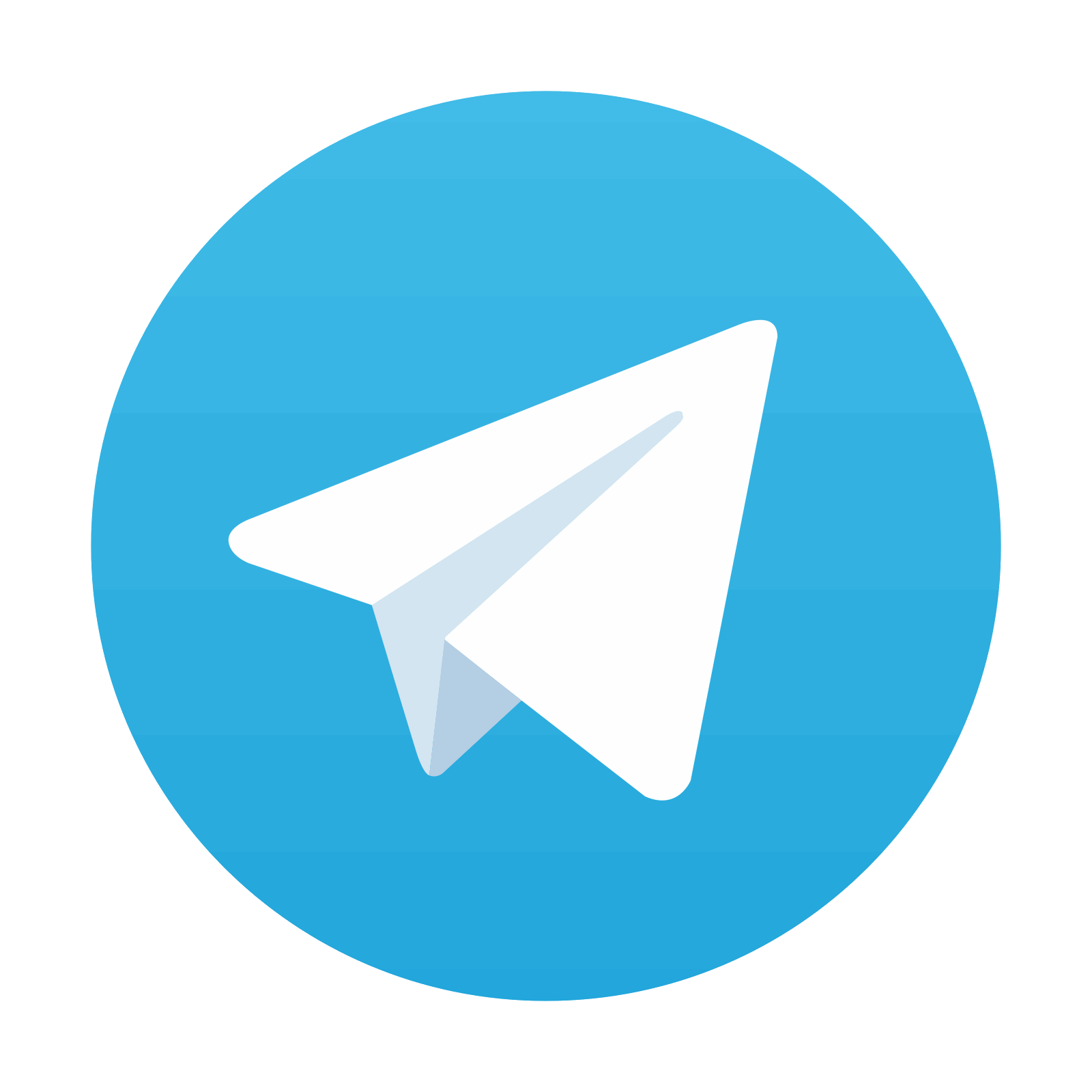
Stay updated, free articles. Join our Telegram channel

Full access? Get Clinical Tree
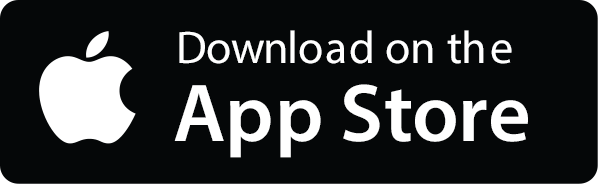
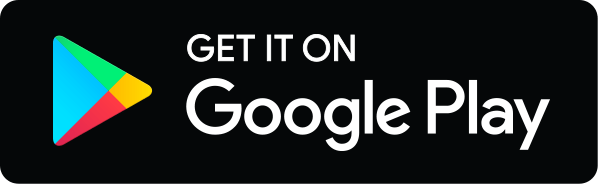
