Abstract
Congenital mitral valve disease is rarely seen in isolation. It is more commonly associated with complex congenital defects, including Shone’s complex, hypoplastic left heart syndrome, transposition of the great arteries, and connective tissue diseases. Symptoms vary based on the degree of obstruction or regurgitation. Obstructive pathologies are treated with diuretics and rhythm management, whereas insufficient pathologies depend on afterload reduction, inotropes, and diuretics. Operative intervention is indicated when medical management fails. Valve repair, not replacement, is the primary goal for congenital mitral valve disease based on the dynamic advantages it confers.
Key Words
Parachute Mitral Valve, Mitral Ring, Mitral Arcade, Double-Orifice Mitral Valve, Mitral Valve Prolapse, Mitral Valve Repair, Mitral Valve Replacement
Isolated congenital mitral valve disease is rare. More commonly, it is associated with complex and challenging congenital cardiac conditions like Shone’s complex and connective tissue disorders. In these situations the abnormal mitral valve continues to serve as the systemic atrioventricular valve. In patients with conotruncal abnormalities a tricuspid valve or an Ebstein anomaly (positioned low in the ventricle with displaced and dysplastic valve leaflets) may be found in the systemic atrioventricular valve position. In the most severe cases, congenital mitral valve disease can occur with single-ventricle pathologies.
Hypoplastic left heart syndrome affects the mitral apparatus, left ventricle, aortic valve, and ascending aorta. The mitral valve is not the primary focus of this presentation, and the goals of care are focused on single-ventricle palliation. However, atrioventricular valve insufficiency may influence long-term morbidity and mortality. Similarly, complete atrioventricular septal defects involve the mitral valve, and complex repairs are often required to septate the ventricles and restore atrioventricular valve function.
Complex congenital cardiac presentations like these are discussed in greater detail elsewhere. This chapter will focus on isolated mitral valve diseases of the infant and child, in which the mitral valve is the primary concern.
History
Mitral valve stenosis was the first mitral valve lesion to be addressed surgically. Elliot Cutler performed the surgery on an 11-year-old girl in 1923 at the Brigham Hospital in Boston. Although this patient survived for 4 years, she was the only survivor of Cutler’s transapical valvotomy technique. In 1947 Dwight Harken, a protégé of Cutler’s at the Brigham, proposed a mitral valve repair with “selective insufficiency.” He believed performing serial resections of the commissures and preserving the anterior leaflet would prove successful. Unfortunately, 6 of his first 10 patients died.
At the same time Charles Bailey was working on a simple commissurotomy approach in Philadelphia. He successfully conducted trials of his technique in the laboratory before applying it clinically. His desire to advance the treatment for this dreaded condition was not without resistance, resulting in the nickname “The Butcher of Hahnemann Hospital,” following the deaths of his first two patients. Learning from his earlier mistakes, he developed an overlapping glove technique to safely slip a hooked knife into the heart. On June 10, 1948, Bailey booked three mitral valve procedures in three different hospitals across Philadelphia–knowing his surgical privileges would be revoked if another patient died. Following the death of the first patient that day, Bailey rushed to the second case and successfully performed the first hook-knife commissurotomy. His patient, a 24-year-old woman with a 2-year history of mitral stenosis, lived another 38 years.
In 1964 Young and Robinson performed the first mitral valve replacement in a 10-month-old child. Shortly thereafter, in 1976 Carpentier and colleagues proposed a systematic way of categorizing and describing congenital mitral disease, thereby uniting the field and laying the foundation for many of today’s surgical repairs.
Embryology
Mitral valve formation begins during the fourth week of gestation and continues through the sixth month. Endocardial cushions begin to advance from the anterior and posterior endomyocardium during the fourth week and merge during the fifth and sixth weeks—dividing the heart into a common atrium and ventricle, as well as two rudimentary atrioventricular canals. The cushions continue to expand laterally, forming early leaflets by the seventh week. Over the next 5 weeks the early leaflets undergo epithelial-to-mesenchymal transition—replacing early endomyocardial tissue with muscle. As the early leaflets transition into muscle, they merge inferiorly with a myocardial ridge that runs from the anterior wall of the left ventricle, through the apex, to the posterior atrioventricular canal. This ridge begins to delaminate from the ventricular wall at approximately 7 weeks’ gestation and can be recognizable as the anterolateral and posteromedial papillary muscles by 10 weeks. By 12 weeks the papillary muscles are only connected to the left ventricle inferiorly and the early leaflets superiorly. As the leaflets thin during the 14th week, chordae begin to appear, and the mitral apparatus is recognizable by the 15th week. Over the next 2 months, the muscular tissue of the leaflets and primitive chordae is slowly replaced by thin collagen, and the mitral apparatus is complete by 6 months.
Isolated malformations of the mitral valve may occur due to irregularities in development at any one of these steps.
- •
Endocardial cushions fail to migrate—mitral atresia
- •
Incomplete endocardial cushion differentiation—mitral valve stenosis
- •
Incomplete endocardial cushion thinning—supramitral or intramitral rings
- •
Union of the early papillary muscles—parachute mitral valve
- •
Incomplete myocardial ridge delamination—parachute-like asymmetric mitral valve
- •
Incomplete chordae development—hammock or arcade mitral valve
Although this list is not exhaustive, it depicts the common isolated mitral valve diseases seen in clinical practice. This chapter will describe each of these conditions, as well as others, in much greater detail. However, before discussing these pathologic findings, it is important to recognize normal mitral valve anatomy and physiology.
Anatomy
Diagnosis and management of mitral valve disease demands a thorough understanding of mitral valve anatomy and physiology ( Figs. 52.1 and 52.2A ). The mitral valve, or mitral apparatus, is made of four distinct parts—a fibromuscular annulus, two leaflets, multiple chordae, and two papillary muscles. The mitral valve separates the left atrium and ventricle. During diastole it acts as a funnel for blood entering the left ventricle, and during systole it acts as a barrier preventing retrograde flow from the left ventricle into the left atrium.


The anterior aspect of the mitral valve is located behind the left main coronary artery slightly below the level of the aortic valve. The anterior border is continuous with the left fibrous trigone, subaortic curtain at the junction of the left coronary and noncoronary sinuses, and the right fibrous trigone more medially. The atrioventricular node is located within the right fibrous trigone, adjacent to the posteromedial commissure of the mitral valve. The posteromedial commissure separates the anterior and posterior leaflets along their posterior margins, whereas the anterolateral commissure defines these leaflets along their anterior margins. The posterior leaflet and remaining annulus share a continuous border with the atrioventricular groove along the posterior and lateral aspects of the valve. The circumflex coronary artery and coronary sinus run within this groove and are intimately involved with the mitral annulus along these borders. Failure to acknowledge the proximity of these important structures may dramatically impact an otherwise successful mitral valve surgery, particularly during valve replacement.
The valvar portion of the mitral apparatus is composed of the annulus and leaflets. The annulus is a D -shaped fibromuscular structure to which the leaflets and surrounding myocardium attach. In three dimensions the annulus more closely resembles a saddle (see Fig. 52.2B ). The anterior third of the annulus is continuous with the left and right fibrous trigones and is attached to the fibrous skeleton of the heart. It supports the anterior leaflet and is the fixed portion of the annulus. The remaining annulus is a dynamic structure that contracts 20% to 40% during systole. It supports the posterior leaflet and is the portion of the annulus that dilates with valvar and ventricular dysfunction.
The anterior leaflet is triangular to trapezoid in appearance and is easily distinguishable from the crescent-shaped posterior leaflet. By definition the posterior leaflet contains three standard clefts, or scallops, but additional deviant clefts are observed in over a third of patients. The anterior leaflet does not contain standard clefts and carries deviant clefts in less than 10% of patients. Standard posterior scallops and their associated anterior leaflet regions are labeled numerically front to back (e.g., P1 : A1 being the anterior-most leaflets and P3 : A3 the posterior-most). The anterior leaflet occupies 150 degrees of the mitral annulus anteriorly, and the posterior leaflet occupies the remaining 210 degrees. The lateral borders of the leaflets are defined by the anterolateral and posteromedial commissures. Commissures are differentiated from clefts by the presence of fanning chordae that span both leaflets inferiorly. False commissures, or clefts, are supported by chordae that do not cross adjacent leaflet margins. Despite their differences, the anterior and posterior leaflets occupy the same surface area and together, in their entirety, measure more than twice that of the primary mitral orifice. Mitral coaptation occurs below the level of the annulus and is dependent on a balanced subvalvar apparatus to prevent incompetence.
The subvalvar apparatus is made of chordae and two papillary muscles. The anterolateral papillary muscle anchors chordae to both leaflets and the anterolateral commissure, and it receives its blood supply from the first diagonal branch of the left anterior descending coronary artery and the obtuse marginal branches of the circumflex coronary artery. The posteromedial papillary muscle anchors chordae to both leaflets and the posteromedial commissure, and it receives 85% of its blood supply from the right coronary artery and 15% from the posterolateral branch of the circumflex coronary artery. Chordae are fibrous thread-like bands that connect the papillary muscles to the leaflets. Approximately 9 chordae originate from the apical portion of each papillary muscle and branch to make over 30 distinct attachments on the leaflets. The area in which the chordae course from the papillary muscles to the leaflets represents the secondary mitral orifice. Chordae are classified into four broad categories based on their origin and insertion.
True chordae originate from the papillary muscle and insert onto the mitral valve leaflet. True chordae can be divided into first-order and second-order chordae. True first-order chordae insert onto the margin or free edge of the leaflet—they are responsible for preventing mitral valve prolapse during systole. A special subset of true first-order chordae are commissural chordae. Commissural chordae are straight chordae that fan distally and insert on the anterior- and posterior-most aspects of both mitral leaflets. True second-order chordae insert further back on the leaflet, closer to the annulus in the rough zone. They tend to be thicker and play a variable role in left ventricular function. False chordae are structural and do not follow the traditional papillary muscle–leaflet pattern. False chordae can lie between adjacent papillary muscles, papillary muscles and the ventricle, or the rough zone of the leaflet and the ventricle.
Classification of Mitral Valve Disease
Valvar disease is often divided into obstructive and regurgitant processes. Unfortunately, congenital mitral valve diseases affecting the infant and child are not always as clear-cut. These pathologies commonly present as pure obstructive or regurgitant processes, but a substantial subset can display a mixed presentation or even be completely asymptomatic.
Following convention, this chapter will divide mitral valve disease into obstructive and regurgitant processes. Diseases with variable presentations will be listed under their most common presentation.
Obstructive Mitral Valve Disease
Congenital Mitral Stenosis
Congenital mitral stenosis is largely a catch-all diagnosis for obstructive lesions involving the mitral valve. These lesions often involve multiple aspects of the mitral apparatus and include diagnoses such as mitral rings, parachute mitral valves, and mitral arcade.
Examples of less characteristic pathologies include tethered commissures and hypoplastic leaflets. These lesions are often associated with larger syndromes or infectious causes, although isolated cases have been reported. Transthoracic echocardiogram with Doppler demonstrates a triangular-to-trapezoid, or funnel-like, acceleration across the mitral valve. Three-dimensional echocardiogram has helped differentiate these obstructive pathologies preoperatively, but meticulous intraoperative investigation remains the gold standard.
Mitral Rings
Mitral rings are composed of redundant annular tissue, classified as either supramitral or intramitral, based on proximity to the mitral leaflets and involvement with the subvalvar apparatus. Mitral rings interrupt transvalvar flow to varying degrees based on their size. They commonly present with obstructive symptoms, but they can also present asymptomatically as an incidental murmur. The etiology of mitral rings is uncertain, although it is believed to involve inadequate separation or thinning of the endocardial cushions during development.
Intramitral rings are thin, fibroelastic rings in direct contact with the mitral leaflets. They restrict the ability of the leaflets to open and always affect the subvalvar apparatus. Supramitral rings are characterized by excess fibrous tissue between the leaflets and the left atrial appendage, sparing the leaflets and the subvalvar apparatus ( Fig. 52.3A and B ). These rings represent progressive lesions that can be completely or partially circumferential. The median age of diagnosis is 3 years, although the presentation is variable and often related to the size of the ring or the presence of additional cardiac conditions (e.g., Shone’s syndrome or ventricular septal defects). Diagnosis with transthoracic echocardiography remains difficult and is made in only 50% to 70% of cases preoperatively. Doppler echocardiography may show a convex acceleration above the mitral valve that arcs superiorly toward the atrium, as compared with the inferiorly orientated acceleration seen with classic congenital mitral stenosis. Supramitral rings should not be confused with cor triatriatum sinistrum.

Cor triatriatum sinister contains a fibromuscular ring located above the level of the left atrial appendage, thereby dividing the left atrium into two compartments (see Fig. 52.3C and D ). Cor triatriatum sinister forms when a left pulmonary vein fails to properly incorporate into the left atrium. Like supramitral rings, these rings can present as obstructive processes or asymptomatically, based on the extent of the disease and activity level of the individual. Often cor triatriatum sinister is associated with atrial septal defects.
Parachute Mitral Valve
The leaflets in parachute mitral valves are anchored to a single papillary muscle by short, thick, hypoplastic chordae—giving the mitral apparatus a parachute-like appearance ( Fig. 52.4 ). The single papillary muscle is often centrally located, as opposed to the anterolateral and posteromedial arrangement seen with normal mitral anatomy. As a consequence, the leaflets are restricted during diastole, taking on a funnel-like appearance and obstructing transvalvar flow. In the apical four-chamber view this funnel-like appearance has been described as pear shaped—a pathognomonic finding in this disease entity. Parachute mitral valves are believed to be the result of papillary muscle fusion between the 5th and 10th week of gestation. Diagnosis is made with three-dimensional echocardiography.

Parachute-like asymmetric mitral valve disease is a similar condition to parachute mitral valve disease but contains two asymmetric papillary muscles. Whereas the posteromedial papillary muscle appears normal, the anterolateral papillary muscle is often positioned higher in the ventricle, with fewer chordae, and in contact with the mitral annulus. Parachute-like asymmetric mitral valve disease arises between the 5th and 15th week of gestation when the early papillary muscle fails to delaminate from the ventricular wall and form appropriate chordae with the valvar apparatus.
Parachute mitral valve can often be managed medically throughout the first decade of life, delaying surgery until the child is substantially larger.
Mitral Valve Atresia
Mitral valve atresia involves the constellation of a small annulus, short chordae, and closely associated papillary muscles. In essence the mitral apparatus never finished growing. Eighty percent of cases are seen in association with larger ventricular anomalies, including hypoplastic left heart syndrome, left ventricular outflow tract obstruction, and double-outlet right ventricle. Infants with mitral valve atresia present with severe obstructive symptoms early in life, often requiring an immediate operation.
Mitral valve function is often inferred in the context of coexisting cardiac malformations. Large ventricular septal defects or single-ventricle pathologies may compensate for decreased forward flow across an atretic mitral valve. In these settings the mitral valve should be interrogated closely and appropriately addressed to minimize the need for subsequent operations.
Pathophysiology of Mitral Valve Obstruction
Mitral valve obstruction, from any cause, results in the restriction of blood flow from the left atrium to the left ventricle. As the left atrial filling pressure increases, the force of contraction increases, and a pressure gradient develops across the obstruction. When the impedance of the obstruction is greater than the contractile strength of the left atrium, left ventricle filling is compromised, and volume backs up into the pulmonary veins. Often in patients with pure mitral valve stenosis, this decrease in left ventricular filling is compounded by a decreased ejection fraction and abnormal contractility. Together, these findings result in diminished cardiac output.
The increase in left atrial, pulmonary venous, and pulmonary capillary wedge pressures results in an increase in interstitial and alveolar fluid. The increase in interstitial fluid along with the pulmonary venous congestion compresses the small airways, restricts ventilation, and contributes to the increased work of breathing that is associated with left atrioventricular obstruction. Simultaneously hypoxemia and hypercapnia develop secondary to alveolar shunting and impaired gas exchange. Late signs and symptoms of mitral valve obstruction include pulmonary artery hypertension, right ventricular hypertrophy, and ultimately right ventricular dysfunction with or without tricuspid insufficiency. At this point, left ventricular filling is affected by both the mitral valve obstruction and the increased pulmonary vascular resistance. In the setting of right-sided failure, cardiac output is further reduced, and peripheral signs of heart failure, including end-organ damage become evident.
Left ventricular filling across the obstructed mitral valve occurs during diastole. With an increasing heart rate the diastolic time decreases, and left atrial contractility normally increases to maintain cardiac output. Due to the increased demands on the left atrium at baseline in severe mitral obstruction, it fails to compensate for the decreased diastolic times during tachycardia, resulting in acute pulmonary congestion. Prolonged mitral valve obstruction increases the risk of atrial dilation and dysrhythmias. Atrial fibrillation is particularly bad for these patients due to the loss of atrial contractility and the decreased diastolic filling times that are seen with rapid ventricular response.
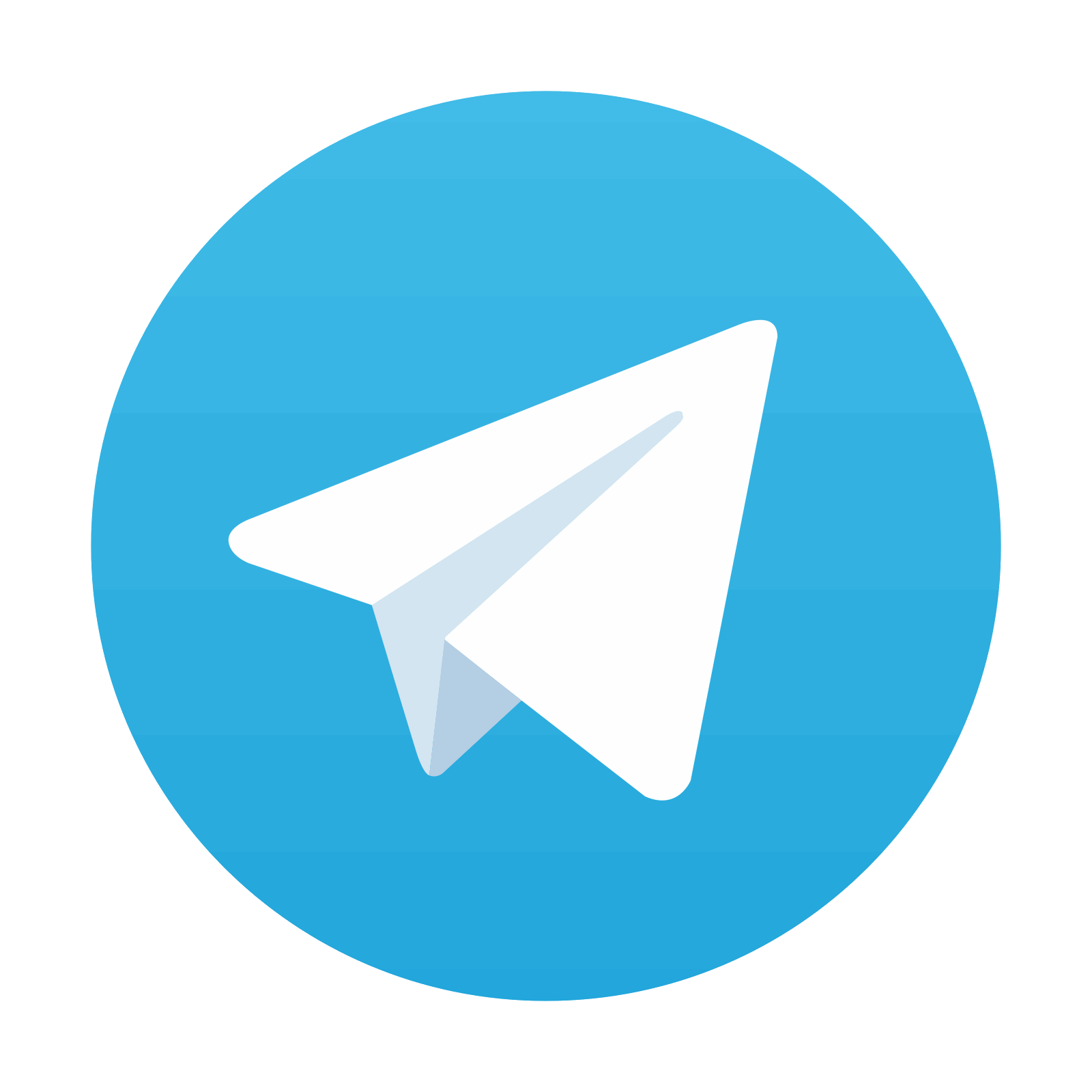
Stay updated, free articles. Join our Telegram channel

Full access? Get Clinical Tree
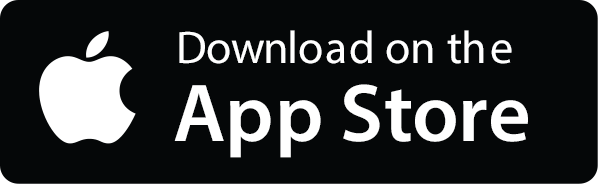
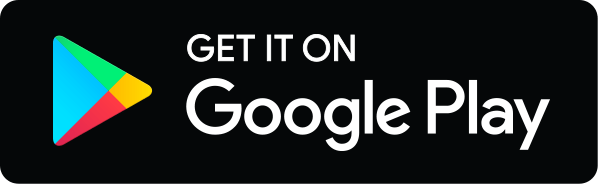