Background
MitraClip implantation reduces mitral regurgitation effectively but decreases mitral valve area, creating iatrogenic mitral stenosis. Evaluation with transesophageal echocardiography intraprocedurally is necessary to measure mitral regurgitation and mitral valve pressure gradient (MVPG) to determine whether it is necessary and safe to place more clips. The aim of this study was to investigate whether these intraprocedural hemodynamics represent postprocedural measurements and whether exercise is affected by the stenosis.
Methods
In this retrospective single-center study, 51 patients who underwent MitraClip implantation were included. Measurements were performed intraprocedurally using transesophageal echocardiography and postprocedurally using transthoracic echocardiography. In 23 of these patients, exercise echocardiography was performed at follow-up.
Results
Intraprocedural mean MVPG was 3.0 ± 1.6 mm Hg and increased to 4.3 ± 2.2 mm Hg postprocedurally ( P < .001). During exercise, mean MVPG increased significantly compared with rest conditions (from 3.6 ± 1.7 to 6.3 ± 2.7 mm Hg, P < .001). Six patients had mean resting MVPGs ≥ 5 mm Hg at follow-up and had higher systolic pulmonary artery pressure (sPAPs) than patients with mean MVPGs < 5 mm Hg (47 ± 7 vs 35 ± 12 mm Hg, P = .035). Higher MVPG and sPAP did not lead to more symptoms of heart failure. Receiver operating characteristic curve analysis showed an estimated cutoff point for intraprocedural pressure half-time of 91 msec to identify patients with mitral stenosis and sPAP ≥ 50 mm Hg postprocedurally.
Conclusions
Mean MVPG during MitraClip implantation measured by TEE underestimates the hemodynamics in daily life, of which operators should be aware when deciding on placing one or more clips. Pressure half-time seems to be the most robust parameter compared with mean and maximum MVPG and may contribute to this decision. Patients with higher mean MVPGs after MitraClip implantation have higher sPAPs at follow-up. However, more symptoms of heart failure were not detected at follow-up.
Patients with severe symptomatic mitral regurgitation (MR) have increased mortality and morbidity. In patients denied for surgical repair or replacement, MitraClip (Abbott Vascular, Santa Clara, CA) implantation may be a good option to reduce symptoms. However, MitraClip implantation decreases mitral valve area (MVA), creating iatrogenic mitral stenosis (MS). This decrease in MVA is without significant mitral obstruction, and surgery for left ventricular inflow obstruction has not been reported. During MitraClip implantation, mitral inflow patterns are measured using transesophageal echocardiography (TEE) to determine whether effective reduction of MR is achieved and whether the degree of MS is acceptable. Both the amount of MR reduction (MR grade > 2 is considered a suboptimal result) and the mitral valve pressure gradient (MVPG) are measured. MS is usually considered significant in case of a mean MVPG ≥ 5 mm Hg. Thus, intraprocedural TEE is necessary to determine whether it is effective and safe to place one or more clips. Which transesophageal echocardiographic measurements are capable of predicting hemodynamics in daily life and thereby able to guide decision making during the procedure are unknown.
In this single-center study, we aimed to investigate the effect of hemodynamic conditions of MitraClip implantation on the occurrence of iatrogenic MS both intraprocedurally and in daily life. Because data on changes in hemodynamic parameters at rest and during exercise echocardiography contribute to decision making during the procedure, a subgroup of our patients performed exercise echocardiography during follow-up to quantify the increase in mitral valve inflow patterns during exercise and to evaluate whether this would impair the functional status of the patients.
Methods
Study Population
Between May 2009 and December 2012, 76 consecutive patients underwent MitraClip implantation at our institution. Before MitraClip implantation, all patients had severe symptomatic MR and were declined for surgical treatment by a multidisciplinary heart team. The MitraClip device and the implantation procedure were described previously.
Of these 76 patients, 51 had echocardiographic measurements available of mitral inflow patterns both intraprocedurally and postprocedurally. MVPG was measured during the procedure using TEE, and in case of an acceptable reduction of MR or an intraprocedural mean MVPG ≥ 5 mm Hg measured at equal blood pressures, no second clip was implanted.
Between January and May 2012, 23 patients underwent additional exercise echocardiography, which was performed at a median follow-up period of 13 months after MitraClip implantation. All patients who underwent MitraClip implantation at our institution and were alive in January 2012 were informed about the exercise study. We selected 23 consecutive patients ( Supplementary Figure 1 ; available at www.onlinejase.com ) who provided written informed consent, were physically able to perform bicycle exercise echocardiography, and had good windows on echocardiography, according to the inclusion and exclusion criteria of our protocol. The exercise study was approved by the medical ethics committee.
Echocardiographic Protocols
All patients underwent TEE during MitraClip implantation and resting transthoracic echocardiography (TTE) preprocedurally and postprocedurally (1 or 2 days after implantation) using a Vivid 7 machine (GE Vingmed Ultrasound AS, Horten, Norway). Echocardiographic recordings were made using a 1.6-MHz to 3.2-MHz transducer (System 7; GE Healthcare, Milwaukee, WI), digitized, and analyzed offline. All views were obtained according to the recommendations of the American Society of Echocardiography. The mean value of three repetitive measurements was taken for every variable in patients in sinus rhythm and five measurements in those with atrial fibrillation. MR grade was based on qualitative and quantitative data from color Doppler and continuous-wave Doppler interrogation of the regurgitant jet in two orthogonal views; color-flow jet area in the left atrium, pulmonary vein flow (in case of no atrial fibrillation), vena contracta width, effective regurgitant orifice area (using the proximal isovelocity surface area method), regurgitant fraction, and regurgitant volume are used at our institution. MR severity was scored from 1 to 4 (1 = mild, 2 = mild to moderate, 3 = moderate to severe, 4 = severe). Vena contracta width could not be used after clip placement, because of the double-orifice valve. After clip implantation, we measured MR severity according to the MR score reported previously. Mean and maximum MVPG were determined using continuous-wave Doppler. When one or more MitraClips were placed (and a double orifice was created), we measured the highest gradient resulting in measurements of the smallest orifice. MVA was measured using the pressure half-time (PHT) method. Right ventricular systolic pressure was obtained from the systolic right ventricular–to–right atrial gradient, calculated from the peak velocity of the systolic transtricuspid regurgitant flow using the modified Bernoulli equation. Right atrial pressure was estimated from the dimension of the inferior vena cava and its collapsibility, measured in the subcostal view according to the guidelines of the American Society of Cardiology. In the absence of pulmonary valve or pulmonary artery stenosis, right ventricular systolic pressure corresponds well to systolic pulmonary artery pressure (sPAP) (none of the patients had pulmonary artery stenosis). Left ventricular ejection fraction and left ventricular end-systolic and end-diastolic volumes were measured using Simpson’s biplane method. Cardiac output was calculated using the left ventricular outflow tract diameter, pulsed-wave Doppler velocity-time integral measurements, and heart rate (HR).
TEE during MitraClip implantation was performed by three experienced cardiologists. Mean and maximum MVPG were determined using continuous-wave Doppler at 0° and 120°.
In a subgroup of patients, TTE at rest and during exercise was performed during follow-up. TTE was performed on a left lateral tilted semirecumbent bicycle ergometer. Patients on β-blockers were asked to discontinue them 24 hours before exercise. Baseline measurements of systolic blood pressure, HR, and peripheral blood oxygen saturation were performed before and at peak exercise. Initial workload started at 25 W and was increased every 2 min by 20 W until 70% of the age-predicted maximum HR (maximum HR = 220 − age) was reached or when symptoms of fatigue or dyspnea developed. All transthoracic echocardiographic examinations at rest and during exercise were performed by a single experienced sonographer.
Data Collection
Baseline characteristics including age, gender, and relevant medical and surgical histories were collected from medical records.
Both New York Heart Association (NYHA) functional class and N-terminal pro–brain natriuretic peptide values were determined preprocedurally and at follow-up according to standard patient care.
All echocardiographic analyzes were performed retrospectively and were reviewed by two experienced investigators.
Statistical Analysis
Data are expressed as mean ± SD for continuous variables and as counts with percentages for categorical data. Paired t tests were performed on continuous data to detect significant differences between periprocedural and postprocedural measurements and rest and exercise parameters. McNemar’s, χ 2 , or Fisher’s exact tests were performed for categorical data. Nonparametric tests were used for data that were not normally distributed.
Because moderate MS was defined as a mean MVPG ≥ 5 mm Hg at rest, the same cutoff value was used to divide the patients into two groups (mean MVPGs of <5 and ≥5 mm Hg at rest).
Hemodynamic parameters were compared between the groups. We performed receiver operating characteristic (ROC) curve analysis, and the area under the curve (with its 95% confidence interval) was determined using the bootstrap method. We used the value 50 mm Hg as a cutoff point for sPAP, because our study population already had a mean preprocedural sPAP of 34 ± 23 mm Hg. P values < .05 were considered statistically significant.
Results
Patient and Procedural Characteristics
Baseline characteristics are summarized in Table 1 . The overall mean age was 75 ± 10 years, and 51% of the patients were men. All patients had MR grades ≥ 3 before implantation, and the MR etiology was functional in 74%. NYHA functional classes were ≥III in 96% of the patients. MR was reduced to grade 1 in 28% of the patients, to grade 2 in 43%, and to grade 3 in 29% of the 51 patients.
Variable | Total group ( n = 51) | Follow-up group ( n = 23) |
---|---|---|
Age at procedure (y) | 75 ± 10 | 76 ± 8 |
BSA (m 2 ) | 1.88 ± 0.2 | 1.87 ± 0.24 |
Men | 26 (51%) | 10 (44%) |
Clinical history | ||
Hypertension | 15 (29%) | 6 (26%) |
Diabetes mellitus | 9 (18%) | 4 (17%) |
Previous stroke | 3 (6%) | 2 (9%) |
Coronary artery disease | 27 (53%) | 6 (26%) |
Previous PCI | 14 (28%) | 4 (17%) |
Previous CABG | 11 (22%) | 1 (4%) |
Atrial fibrillation | 27 (53%) | 16 (70%) |
COPD | 6 (12%) | 4 (17%) |
NYHA class ≥ III | 49 (96%) | 21 (91%) |
EuroSCORE | 15 ± 10 | 13 ± 10 |
STS score | 4.28 ± 3.62 | 3.4 ± 2.22 |
Echocardiographic variables | ||
MR grade ≥ 3 | 51 (100%) | 23 (100%) |
MR etiology | ||
Degenerative | 12 (24%) | 6 (26%) |
Functional | 39 (74%) | 17 (74%) |
Mixed | 1 (2%) | |
Mean MVPG (mm Hg) | 2.2 ± 1.5 | 2.2 ± 1.9 |
Maximum MVPG (mm Hg) | 6.3 ± 4.4 | 5.6 ± 4.3 |
PHT (msec) | 74 ± 27 | 67 ± 18 |
MVA by PHT (cm 2 ) | 3.3 ± 1.2 | 3.2 ± 1.1 |
Poor LV function (EF < 30%) | 16 (31%) | 6 (26%) |
sPAP (mm Hg) | 34 ± 23 | 31 ± 20 |
In the overall group of 51 patients, 16 (31%) had more than one MitraClip implanted (63% of these patients had functional MR). Of the 23 patients who also underwent exercise echocardiography, four (17%) had received more than one MitraClip.
Echocardiographic Data
Echocardiographic parameters intraprocedurally and postprocedurally are shown in Table 2 and Figure 1 A. In all included patients, the mean MVPG was lower intraprocedurally than postprocedurally (2.95 ± 1.6 vs 4.25 ± 2.20 mm Hg, P < .001). Maximum MVPG (7.9 ± 3.2 vs 10.2 ± 4.3 mm Hg, P < .001) and HR (69 ± 18 vs 81 ± 25 beats/min, P < .001) were also lower intraprocedurally compared with postprocedurally, while PHT (99 ± 40 vs 95 ± 30 msec, P = .30) did not differ significantly. Iatrogenic MS, defined as a mean MVPG ≥ 5 mm Hg measured postprocedurally at resting conditions, occurred in 18 of these patients (35%).
Variable | Intraprocedurally | Postprocedurally | P |
---|---|---|---|
Mean MVPG (mm Hg) | 2.95 ± 1.58 | 4.25 ± 2.2 | <.001 |
Max MVPG (mm Hg) | 7.90 ± 3.25 | 10.25 ± 4.32 | <.001 |
PHT (msec) | 99 ± 40 | 95 ± 30 | .30 |
HR (beats/min) | 69 ± 18 | 81 ± 25 | <.001 |

We divided the 23 patients who underwent exercise echocardiography into two groups according to the presence of iatrogenic MS in resting conditions. Patients with mean MVPGs ≥ 5 mm Hg at follow-up ( n = 6) had already shown higher intraprocedural mean MVPGs on TEE (3.56 ± 0.67 vs 2.39 ± 1.34 mm Hg, P = .054) and higher PHTs (130 ± 56 vs 86 ± 30 msec, P = .03) than patients with mean MVPGs < 5 mm Hg ( n = 17) ( Table 3 ).
Variable | Mean MVPG < 5 mm Hg ( n = 17) | Mean MVPG ≥ 5 mm Hg ( n = 6) | P |
---|---|---|---|
Mean intraprocedural MVPG | 2.39 ± 1.34 | 3.56 ± 0.67 | .05 |
Maximum intraprocedural MVPG | 6.55 ± 2.71 | 8.33 ± 1.3 | .07 |
Intraprocedural PHT | 86 ± 30 | 130 ± 56 | .03 |
Mean postprocedural MVPG | 4.00 ± 2.4 | 6.4 ± 2.4 | .08 |
Maximum postprocedural MVPG | 8.77 ± 3.2 | 14.4 ± 3.85 | .02 |
Postprocedural PHT | 89 ± 28 | 112 ± 44 | .07 |
Exercise Echocardiography
Table 4 shows the changes in hemodynamic and echocardiographic parameters between rest and exercise. Before exercise echocardiography, 48% of the patients were in sinus rhythm and 52% were in atrial fibrillation (4% had pacemakers). Most of the patients (74%) were in NYHA class II at the time of the investigation; 22% were in class I, and 4% were in class III.
Variable | Rest | Exercise | P |
---|---|---|---|
HR (beats/min) | 80 ± 15 | 100 ± 24 | .001 |
SBP (mm Hg) | 142 ± 22 | 155 ± 35 | .03 |
Oxygen saturation (%) | 97 ± 2 | 96 ± 3 | .01 |
Mean MVPG (mm Hg) | 3.6 ± 1.7 | 6.3 ± 2.7 | <.001 |
Maximum MVPG (mm Hg) | 8.9 ± 4.0 | 13.7 ± 4.6 | <.001 |
MR grade | .1 | ||
None | 1 (4%) | 1 (4%) | |
1 | 3 (13%) | 1 (4%) | |
2 | 12 (52%) | 11 (48%) | |
3 | 7 (31%) | 10 (44%) | |
PHT (msec) | 98 ± 30 | 81 ± 26 | <.001 |
MVA by PHT (cm 2 ) | 2.46 ± 0.73 | 3.04 ± 0.94 | <.001 |
LVEDV (mL) | 137 ± 71 | 134 ± 66 | .7 |
LVESV (mL) | 83 ± 56 | 80 ± 50 | .6 |
LVEF (%) | 43 ± 14 | 41 ± 15 | .4 |
sPAP (mm Hg) | 38.8 ± 12 | 51.9 ± 11 | <.001 |
Cardiac output | 4.2 ± 1.1 | 6.1 ± 2.0 | .001 |
Mean MVPG increased significantly during exercise (3.6 ± 1.7 vs 6.3 ± 2.7 mm Hg, P < .001; Figures 1 B and 2 ). In three patients, there was no increase in mean MVPG during exercise, because of accidental intake of a β-blocker the morning before exercise test (one patient) and atrial fibrillation with severely varying HR during exercise, which influenced the measurement of MVPG although measuring five cycles (two patients). In six patients (26%), MR grade increased during exercise. Also, systolic blood pressure (142 ± 22 vs 155 ± 35 mm Hg, P = .03) and estimated sPAP increased significantly during exercise (38.8 ± 12 vs 51.9 ± 11 mm Hg, P < .001).

The 23 patients were divided into two groups ( Table 5 ): patients with mean MVPGs < 5 mm Hg ( n = 17) and those with mean MVPGs ≥ 5 mm Hg ( n = 6) at rest. A higher mean MVPG resulted in a significantly higher sPAP ( P = .035) in resting conditions, but not during exercise. There were no differences in NYHA class or N-terminal pro–brain natriuretic peptide measurements between the two groups. The mean duration of exercise did not differ between the groups: in those with mean MVPGs < 5 mm Hg, 15.3 ± 2.3 min, and in those with mean MVPGs ≥ 5mm Hg, 14.3 ± 2.3 min ( P = .40).
Variable | Rest | Exercise | ||||
---|---|---|---|---|---|---|
Mean MVPG < 5 mm Hg ( n = 17) | Mean MVPG ≥ 5 mm Hg ( n = 6) | P | Mean MVPG < 5 mm Hg ( n = 17) | Mean MVPG ≥ 5 mm Hg ( n = 6) | P | |
NYHA class | 1.78 ± 0.55 | 2.00 ± 0.00 | .20 | |||
NT-proBNP (ng/L) | 2,276 ± 1,859 | 3,003 ± 1,687 | .60 | |||
HR (beats/min) | 70 ± 12 | 86 ± 18 | .42 | 95 ± 20 | 99 ± 28 | .60 |
SBP (mm Hg) | 140 ± 12 | 147 ± 20 | .50 | 153 ± 36 | 161 ± 35 | .80 |
Oxygen saturation (%) | 97 ± 2 | 98 ± 2 | .40 | 95 ± 3 | 96 ± 3 | .10 |
Mean MVPG (mm Hg) | 2.8 ± 1.0 | 5.9 ± 1.2 | <.001 | 5.4 ± 2.1 | 9.0 ± 2.7 | .90 |
Maximum MVPG (mm Hg) | 7.5 ± 3.2 | 13.0 ± 3.4 | .006 | 12.2 ± 3.6 | 18.0 ± 4.5 | .90 |
MR grade < 3 | 13 (76%) | 3 (50%) | .30 | 11 (65%) | 2 (33%) | .40 |
MR grade 3 | 4 (14%) | 3 (50%) | 6 (35%) | 4 (67%) | ||
PHT (msec) | 98 ± 28 | 99 ± 38 | .90 | 80 ± 28 | 85 ± 41 | .10 |
MVA by PHT (cm 2 ) | 2.46 ± 0.76 | 2.44 ± 0.70 | .80 | 3.01 ± 0.93 | 3.11 ± 1.12 | .20 |
LVEDV (mL) | 144 ± 71 | 107 ± 55 | .30 | 151 ± 72 | 103 ± 35 | .20 |
LVESV (mL) | 91 ± 58 | 54 ± 29 | .20 | 98 ± 54 | 48 ± 22 | .11 |
LVEF (%) | 41 ± 11 | 52 ± 16 | .08 | 36 ± 13 | 53 ± 14 | .031 |
sPAP (mm Hg) | 35 ± 12 | 47 ± 7 | .035 | 50 ± 12 | 55 ± 7 | .20 |
Cardiac output | 4.04 ± 0.8 | 4.67 ± 1.62 | .50 | 6.39 ± 1.87 | 5.45 ± 2.47 | .08 |
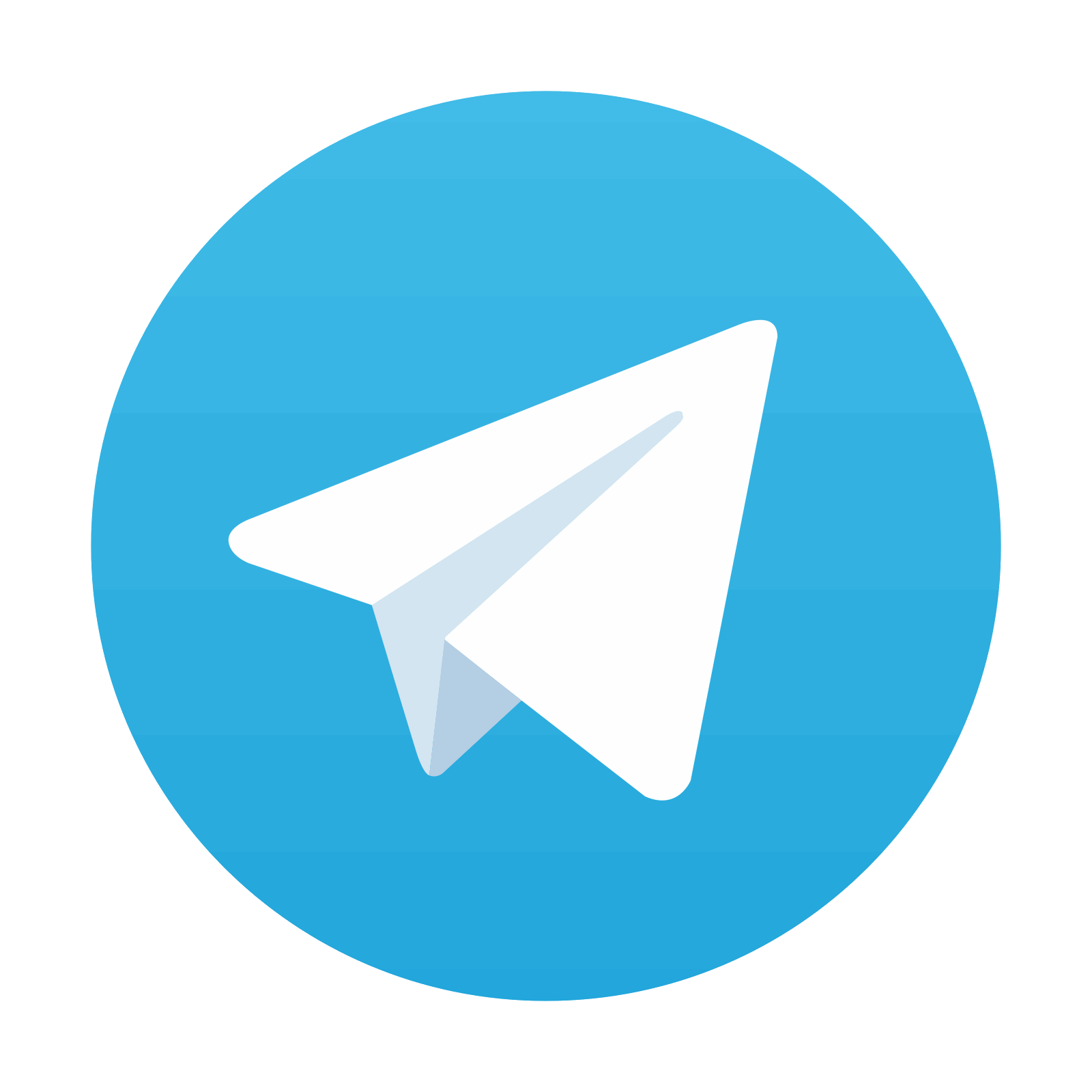
Stay updated, free articles. Join our Telegram channel

Full access? Get Clinical Tree
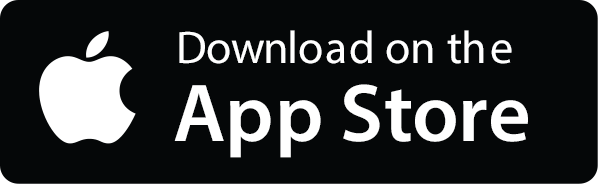
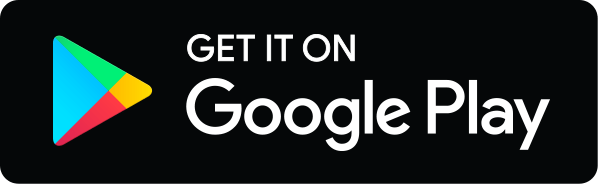
