Fig. 13.1
MicroRNAs involved in the pathogenesis of cardiovascular disease. The figure illustrates the contribution of selected miRNAs to remodeling of the heart. Manipulation of these miRNAs may hinder the progression of cardiovascular pathology, with promising therapeutic prospects
Recently, Li et al. found that miR-328 induces cardiac hypertrophy by targeting SERCA2a. The overexpression of miR-328 led to severe hypertrophy accompanied by reduced SERCA2a level in both transgenic mice and in cultured neonatal rat cardiomyocytes. The subsequent normalization of miR-328 levels through the use of anti-miR-328 led to a reversal of both the structural and molecular changes [47].
The aforementioned studies suggest that miRNAs can function either as agonists, driving and enhancing the cardiac hypertrophic response (e.g., miR-208, miR-23a, miR-199b, miR-499, miR-21, miR-328, miR-212/132), or as antagonists, suppressing or attenuating cardiac hypertrophy (e.g., miR-1, miR-133, miR-26b, miR-29, miR-9, miR-98), by interfering with specific signaling cascades (Fig. 13.1). They exemplify how understanding the molecular mechanisms underlying certain pathologies can lead to innovative therapeutic approaches.
13.3.2 Heart Failure (HF)
As delineated above, a multitude of cardiovascular diseases (e.g., cardiomyopathies, coronary artery disease, cardiotoxic injury, valvular heart disease, cardiac hypertrophy, myocarditis) can result in cardiac hypertrophy and failure. Heart failure is defined as severe impairment in cardiac structure or function in a way that the heart may no longer maintain sufficient blood circulation to peripheral organs. Patients typically present with dyspnea, ankle swelling, fatigue, pulmonary crackles, or increased filling of the jugular veins. However, symptoms might not be typical in all cases, and especially patients in early disease stages are often asymptomatic. Clinical management of HF patients is thus challenging and would greatly benefit from novel molecular biomarkers that could not only aid in diagnosis but also facilitate individual risk assessment and allow estimation of prognosis.
Tijsen et al. investigated the potential of circulating plasma miRNAs as biomarkers in heart failure patients. In an initial screening phase, microarray analysis was performed on plasma samples collected from 12 healthy and 12 HF patients. The 16 candidate miRNAs were subsequently assessed by real-time PCR in an independent patient cohort, consisting of 50 dyspnea cases – 30 HF and 20 non-HF patients – and 39 healthy controls. Six miRNAs were significantly upregulated in HF patients compared to other dyspnea subjects and healthy controls. Moreover, the collected data indicated miR-423-5p as a good diagnostic predictor of HF with an area under the curve (AUC) of 0.91. Remarkably, the expression level of miR-423-5p correlated with disease severity (i.e., with NT-proBNP levels and left ventricular ejection fraction), as well as clinical symptoms (i.e., NYHA classification) [8]. The miRNA profiles in plasma and serum differ from those found in whole blood samples, because the latter contain miRNAs from all circulating cells. In a recent study conducted by Vogel et al., a set of dysregulated miRNAs were found in patients suffering from nonischemic systolic HF [9]. While selected miRNAs have already good diagnostic value as single markers (e.g., miR-558, miR-122*, miR-520d-5p), combining several miRNAs to a multivariate signature consistently improves the diagnostic power, yielding an AUC value of 0.81, being superior to natriuretic peptides in this cohort. Remarkably, the expression level of miR-519e* also correlated with cardiac events (i.e., rehospitalization due to HF, heart transplantation, myocardial infarction, cerebral ischemia, and cardiovascular death) and hence prognosis (Table 13.1).
Table 13.1
MiRNAs as biomarkers for cardiovascular disease
Cardiovascular disease | MiRNA biomarkers | Biosample | Reference |
---|---|---|---|
Heart failure | miR-423-5p | Plasma | [8] |
miR-519e*, miR-558, miR-122*, miR-520d-5p, miR-200b*, miR-622, miR-1231, miR-1228* | Whole blood | [9] | |
Coronary artery disease | miR-140-3p, miR-182, miR-92a, miR-92b | Whole blood | [11] |
miR-19a, miR-484, miR-155, miR-222, miR-145, miR-29a, miR-378, miR-342, miR-181d, miR-150, miR-30e-5p | Whole blood | [12] | |
miR-126, miR-17, miR-92a, miR-145, miR-155, miR-133, miR-208a | Plasma | [13] | |
miR-340*, miR-624*, miR-451, miR-454, miR545, miR615-5p, miR-1280 | Platelets | [14] | |
miR-134, miR-370, miR-198, miR-135, miR-147 | PBMCs | [15] | |
miR-146a, miR-146b | PBMCs | [16] | |
Acute myocardial infarction | miR-1, miR-133a, miR-133b, miR-499-5p, miR-122, miR-375 | Plasma | [48] |
miR-1, miR-133a | Serum | [49] | |
miR-208a, miR-1, miR-133a, miR-499 | Plasma | [50] | |
miR-208b, miR-1, miR-133a, miR-499-5p | Plasma | [51] | |
miR-208b, miR-499 | Plasma | [52] | |
miR-1, miR-133a, miR-208a | Serum | [53] | |
miR-1291, miR-663b, miR-145, miR-30c | Whole blood | [7] | |
miR-636, miR-7-1*, miR-380*, miR-1254, miR-455-3p, miR-566, miR-1291 | Whole blood | [54] | |
Essential arterial hypertension | miR-let7e, miR-296-5p, hcmv-miR-UL112 | Plasma | [17] |
13.3.3 Coronary Artery Disease (CAD) and Myocardial Infarction (MI)
Coronary artery disease refers to the manifestation of atherosclerosis in coronary vessels. The progression of atherosclerotic plaques narrows the lumen of coronary arteries, leading to an insufficient blood supply of the downstream myocardium (Chaps. 19 and 25). Independently, the rupture of any unstable plaque and the subsequent thrombus formation leads to complete or partial vessel occlusion, followed by ischemia and necrosis. Unfortunately, endogenous repair mechanisms are often insufficient for an effective regeneration of the entire ischemic myocardium at risk, resulting in loss of vital cardiac tissue and detrimental remodeling processes. This can result in cardiac fibrosis and impaired cardiac contractility, some of the key factors in the development of heart failure (Chap. 3).
The miRNAs identified in plasma/whole blood samples of patients with coronary artery disease originate from various cell types involved in the underlying pathogenesis. A miRNA-based biomarker that could differentiate unstable from stable atherosclerotic lesions may play a crucial role in detecting patients at risk for an acute myocardial infarction.
Contribution of various cell types (e.g., endothelial, immune, platelets, smooth muscle cells) is observed during the formation of atherosclerotic plaques. The activation of these cells might lead to the release of cell-specific miRNAs, which are then assessable in serum or plasma samples of CAD patients. Fichtlscherer and colleagues found a specific pattern of endothelial-originated (e.g., miR-17, miR-126, miR-92a), inflammatory (e.g., miR-155), and smooth muscle-derived (e.g., miR-145) miRNAs in the plasma of patients with stable CAD (Table 13.1). Counterintuitively, these miRNAs were downregulated in affected patients, although the activation of vascular and inflammatory cells during atherosclerotic changes is a well-known process. Speculations regarding this miRNA downregulation include the idea that circulating miRNAs derived from activated cells might be taken up into atherosclerotic lesions. Furthermore, cardiac miRNAs (e.g., miR-133, miR-208a) showed increased plasma levels, which might hint towards recurrent subclinical ischemia with cardiomyocyte death and subsequent miRNA release into the blood stream. Another cell type that is deeply involved in atherosclerotic plaque formation, as well as thrombus formation during AMI, are platelets. At least two miRNAs derived from platelets (miR-340*, miR-624*) were shown to be upregulated in CAD patients when compared to controls [14]. Furthermore, several studies revealed different miRNAs to be dysregulated in whole blood samples of CAD patients [11, 12]. However, these studies were conducted in relatively small patient cohorts and results therefore need to be interpreted with care.
In patients with prevalent CAD, unstable atherosclerotic plaques hold the risk of plaque rupture and subsequent acute myocardial infarction (Chaps. 19 and 25). Therefore, the identification of patients with such high-risk lesions may help prevent serious cardiovascular events. Specific miRNAs derived from peripheral blood mononuclear cells (PBMC) (e.g., miR-134, miR-370, miR-198) were found to be significantly increased in patients with unstable angina pectoris compared to those experiencing stable angina [15]. Moreover, the PBMC-derived miR-146a was shown to be an independent indicator for cardiac events [16].
Undoubtedly, a major interest of cardiovascular research lies in the discovery of novel biomarkers for MI. Cardiac troponins are today’s gold standard biomarkers for the detection of acute myocardial infarction. They can be measured in patients’ blood samples after being released from dying cardiomyocytes using antibody-dependent assays [55]. The development of high-sensitivity cardiac troponin T assays (hs-cTnT) allowed the detection of even very low amounts of cTnT, resulting in higher diagnostic sensitivity and improved risk prediction, but at the cost of reduced specificity [56, 57]. This is due to the fact that troponin levels may also be elevated in response to many other cardiovascular diseases, such as pulmonary thromboembolism, myocarditis, acute tachycardia, or heart failure, and hence, novel biomarkers could help increase specificity and add precision to the diagnosis of MI (Chaps. 10 and 11).
A set of studies suggest that circulating cardiac-specific and muscle-enriched miRNAs are dysregulated due to cardiac necrosis in the course of MI. The most frequently found candidates in plasma and/or serum are miR-1, miR-133a, miR-499, and miR-208b [48–52]. Meder and colleagues found 121 non-cardiac-specific miRNAs significantly dysregulated in peripheral blood samples of MI patients, with miR-663b and miR-1291 showing best statistical power. Remarkably, combining 20 different miRNAs into a multivariate signature could improve diagnostic power (AUC = 0.99) when compared to single markers. In addition, the levels of miR-145 and miR-30c highly correlated with TnT values and disease severity [7]. Differences between studies are due to the analytes used, the methods for miRNA detection, and most likely also due to the release kinetics during MI, since sample collection occurred at different time points after the initial cardiac event. In a kinetic study conducted on patients with hypertrophic obstructive cardiomyopathy undergoing transcoronary ablation of septal hypertrophy (TASH), Liebetrau et al. showed that the release pattern of different cardiac miRNAs was time dependent. miR-1 and miR-133a were already significantly increased 15 min after the procedure and showed peaks at 75 min and 75–480 min, respectively, whereas miR-208a showed a significant elevation 105 min post TASH with no further increase thereafter [53]. The authors hence concluded that miRNA expression patterns are altered already at a very early stage of MI, with different miRNAs showing different release kinetics. On a genome-wide level, our group identified several miRNAs that are early dysregulated during ST elevation MI. We relied on whole blood samples to also capture active mechanisms of cells involved in the pathogenesis of MI, such as vascular injury, inflammation, or plaque rupture. A subset of seven miRNAs (miR-636, miR-1291, miR-1254, miR-566, miR-455-3p, miR-380*, miR-7-1*) could reliably distinguish affected individuals from controls already very early during MI, even before observable changes in cTnT levels were noted.
The remodeling process following myocardial ischemia and necrosis is based on the formation of fibrotic scar tissue, which relies on the deposition of collagen components in response to the stimulation of extracellular matrix (ECM) genes. In this context, downregulation of the miR-29 family members is associated with cardiac fibrosis [58]. These specific miRNAs are able to simultaneously target multiple genes involved in fibrotic repair mechanism (i.e., elastin, fibrillins, collagens). Therefore, miR-29 mimics might be able to suppress the expression of ECM genes, hence inhibiting the development of excessive fibrosis that might result in dilatation of the ventricle. Furthermore, miR-24, also closely related to ECM remodeling, is able to improve heart function by reducing fibrosis in infarct border zones of murine hearts. The effects, which were evident 2 weeks after myocardial infarction in mice that underwent intramyocardial injection of lentivirus miR-24, presumably rely on the ability of miR-24 to target the furin-TGF-β signaling pathway [59].
The cardioprotective effect of miRNAs relies on their ability to influence angiogenesis, prevent calcium overload, and modulate apoptosis. Novel therapies may therefore lead to an increased reperfusion, a reduced infarct size, and an improved contractile function in post-myocardial infarction patients.
The expression of the miR-15 family, a group of six related miRNAs (miR-15a, miR-15b, miR-16-1, miR-16-2, miR-195, miR-497) with similar seed regions but different 3′-sequences, is known to be enhanced after myocardial infarction in both porcine and murine models [60]. Remarkably, silencing of the miR-15 family members through systemic delivery of small LNA-modified inhibitors (8 or 16 nucleotides in length) designed to target the shared seed region of the related miRNAs reduced infarct size and improved cardiac function. This illustrates how miRNA modulators can be used to target multiple, closely related miRNAs and hence might evoke a strong effect. Interestingly, the comparison of intravenous, subcutaneous, intraperitoneal, and gavage administration of the therapeutic agents within this study suggested a similar inhibition through all administration routes, except the oral delivery which was slightly less efficient than the others.
Rapid reperfusion of the ischemic myocardium is considered to be the most effective approach in limiting myocardial infarct size and improving patient’s clinical outcome. However, restoring the blood flow may paradoxically also lead to cardiomyocyte death and significant expansion of the infarct zone, a phenomenon known as myocardial reperfusion injury. Several miRNAs show dysregulated patterns in the advent of ischemia-reperfusion (I/R) injury, suggesting that a possible cardioprotective approach could derive from their manipulation. By generating a mouse model with cardiac overexpression of miR-494 and subjecting it to I/R injury, Wang and colleagues showed that transgenic hearts displayed an improved recovery of their contractile properties during reperfusion and a significantly reduced apoptosis level compared to wild-type hearts. Remarkably, myocardial infarction size was significantly smaller in miR-494-overexpressing hearts. The cardioprotective effects described here presumably rely on the activation of the Akt-mitochondrial signaling pathway as an endpoint of a very complex regulatory process involving both pro- and antiapoptotic targets of miR-494 [61]. Furthermore, heat shock proteins, which play an important role in the physiological recovery of the myocardium especially in uncomplicated cases of acute myocardial infarction, are also targeted by miRNAs. Ren et al. showed that the role of miR-320 as a cellular regulator during I/R injury is based on its ability to target heat shock protein 20. Knockdown of miR-320 using a cholesterol-linked antagomir significantly reduced cardiac infarct size in mice [62].
An important process during myocardial reperfusion is the rapid increase of the intracellular Ca2+ concentration after blood flow restoration. The cardioprotective role of miR-214 during I/R injury seems to rely on the translational repression of the sodium/calcium exchanger 1 (Ncx1), an important regulator of calcium influx and various other downstream factors involved in the calcium signaling pathway. Accordingly, miR-214 knockout mice experienced higher mortality rates and increased injury after MI [63]. Thus, the manipulation of miR-214 might inhibit calcium overload in reperfused cardiomyocytes, thereby preventing myocardial damage.
Angiogenesis is an important factor that triggers cell survival and myocardial regeneration after an acute myocardial infarction. Several miRNAs (e.g., miR-126, miR-92a, miR-24, miR-21, miR-503) regulate angiogenesis, and their manipulation might therefore offer the possibility to increase long-term cardiac perfusion, which in turn can lead to a functional recovery of the myocardium especially in the infarct border zone. For instance, miR-92a controls the growth of new blood vessels by targeting different proangiogenic proteins (e.g., the integrin subunit α5). Overexpression of miR-92a in endothelial cells suppressed angiogenesis in vivo, whereas a chemically modified inhibitor of miR-92a led to an increased growth of blood vessels upon systemic delivery in mice with limb ischemia and myocardial infarction [64]. Moreover, a recent study reported a similar beneficial effect of miR-92a antagonism in a porcine animal model of I/R injury. Besides systemic delivery, a site-specific application could also reduce infarct size and the loss of contractile function post ischemia [65]. Fiedler et al. assessed the role of miR-24 in endothelial cells and found a significantly increased level of this particular miRNA following cardiac ischemia. Remarkably, the inhibition of miR-24, which targets several transcription factors (GATA2, PAK4), stimulated angiogenesis and reduced infarct size in mice notably. Interestingly, low-dose treatment (5 mg/kg) with a specific antagomir resulted in a selective cellular uptake by endothelial cells, whereas high doses (80 mg/kg) led to a nonspecific enrichment of the antagomir in all cardiac cell types [66]. This example conveys an important message regarding the connection between cell-type selectivity of miRNA analogs and applied dosage.
Despite all the advanced treatment options, MI might still cause irreversible cardiac damage. Excessive scar formation leads to severe contractile dysfunction of the myocardium, which can result in heart failure. Advances in the field of regenerative medicine are thus of great interest, exploiting miRNAs as possible candidates to enhance myocardial regeneration. A broad range of studies conducted for this purpose focused on two main mechanisms: the reactivation of cardiomyocyte proliferation and the stimulation and differentiation of cardiac progenitor cells.
The manipulation of different miRNAs (e.g., miR-15 family, miR-195, miR-133, miR-17-92, miR-590, miR-199a), which are known to impact on the cell cycle, was shown to promote cardiomyocyte proliferation in various animal models [67]. The upregulation of the miR-15 family members, for instance, is associated with a definite withdrawal of cardiomyocytes from the cell cycle during neonatal development. Therefore, a postnatal knockdown of these miRNAs through anti-miRs administered in vivo increased the number of mitotic cardiomyocytes with promising regenerative potential [68]. However, excessive cell cycle progression may also result in cellular hypertrophy or apoptosis. Therefore, promitotic mechanisms must be assessed carefully, especially before they are considered for clinical applications.
Cardiac cells with stem cell properties can proliferate and differentiate into different cell types such as cardiomyocytes and endothelial or vascular smooth muscle cells. However, their regenerative potential is rather limited, especially after extensive cell loss like the one occurring post myocardial infarction. Since miRNAs act as regulators of both proliferation and differentiation, their manipulation might result in a regenerative potential of such cardiac progenitor cells [69]. Inline Jayawardena et al. could show that lentiviral-mediated delivery of several miRNAs (e.g., miR-1, miR-133, miR-208, miR-499) into infarct border zones of murine hearts induced the conversion of fibroblasts to cardiomyocytes in situ [70]. Using alternative strategies, a subset of miRNAs was shown to enhance viability of injected stem cells in infarcted hearts [71] or influence intercellular communication for the purpose of local and maybe even distant reprogramming of stem cells [72].
13.3.4 The Vascular System
Vascular injury and remodeling contribute to numerous vascular pathologies, including atherosclerosis, essential arterial hypertension, pulmonary arterial hypertension, aneurysm formation, and restenosis after angioplasty. miRNAs play an important role in the pathogenesis of several vascular-related diseases by orchestrating the molecular mechanisms underlying vascular remodeling and dysfunction.
Important contributors to vascular remodeling processes are vascular smooth muscle cells (VSMCs). Unlike most differentiated cells, VSMCs undergo phenotype switching, thus alternating between a differentiated and a dedifferentiated state in response to various extracellular stimuli [73]. This phenotypic modulation capacity is essential for vascular repair, since dedifferentiated VSMCs show increased proliferation rates, an enhanced production of extracellular matrix components, and the ability to migrate. Once the vascular damage is repaired, healthy VSMCs return to a differentiated, contractile phenotype [74]. While under physiological conditions the phenotypic plasticity of VSMCs contributes to normal homeostasis, its dysregulation may rapidly promote the development and progression of vascular pathology (e.g., atherosclerosis, pulmonary hypertension, restenosis after angioplasty). The VSMC-enriched miR-143/145 are important regulators of VSMC differentiation. Several animal models of vascular disease, including carotid artery-ligated mice, carotid balloon injury rats, or ApoE knockout mice, show significantly decreased expression profiles of miR-143/145 [75–77]. The functional modulation of these particular miRNAs in vivo prevented neointimal formation by reducing VSMC proliferation in animal models of vascular injury [78, 79]. Furthermore, Lovren et al. used a lentivirus-mediated overexpression of miR-145 in ApoE-deficient mice to limit atherosclerotic plaque development and to prevent plaque rupture with promising therapeutic prospects [80]. Moreover, low levels of miR-145 can be detected in the serum of patients suffering from coronary artery disease, suggesting the potential of miR-145 as a biomarker that may predict the severity or size of atherosclerotic lesions [13], while on the other hand, increased levels of miR-145 can be detected during AMI [7]. Other miRNAs involved in the phenotype switch of VSMCs include miR-1, miR-133, miR-21, miR-221, miR-146a, miR-24, and miR-26a [74, 81].
Angiogenesis is a finely tuned process that relies on the activation, proliferation, and migration of endothelial cells. Therefore, miRNAs that influence endothelial cell function (e.g., miR-126, miR-92a, miR-23/27/24, miR-503, miR-21) are of major interest in vascular pathologies. While it is widely recognized that diabetes mellitus interferes with endothelial cell function and with postischemic, compensatory angiogenesis, the exact molecular mechanisms are not fully understood. In a study conducted by Caporali and colleagues, miR-503 showed an increased expression level in endothelial cells of ischemic limb muscles of diabetic mice. Remarkably, the adenoviral delivery of an miR-503-targeting decoy ameliorated impaired angiogenesis in diabetic animals and restored blood flow in the ischemic limbs [82]. However, angiogenesis is not always helpful; under certain circumstances, it may have devastating effects. The hallmark of age-related macular degeneration (AMD), a major cause of blindness in elderly patients, is the ischemia-triggered choroidal neovascularization, a process that relies on angiogenesis. Zhou et al. showed that in vivo inhibition of two specific miRNAs (miR-23, miR-27) reduced choroidal neovascularization in mice with laser-induced choroidal injury, thus preventing the occurrence of neovascular macular degeneration in this animal model [83].
Essential arterial hypertension is a major risk factor for myocardial infarction, congestive heart failure, chronic renal failure, and stroke (Chaps. 30 and 31). Vascular remodeling is a major factor which contributes to an increased total peripheral resistance, which not only facilitates the progression of arterial hypertension but also leads to serious complications that may compromise the function of multiple organs. Although the mechanism underlying vascular remodeling is usually associated with cellular growth, other processes such as apoptosis, low-grade inflammation, and fibrosis also contribute to this pathology [84]. Furthermore, an abnormal activation of the renin-angiotensin-aldosterone system (RAAS) may also play an important role in the pathogenesis of essential arterial hypertension. In this context, Nossent et al. showed that SNPs located in miRNA binding sites of genes related to RAAS can influence not only blood pressure but also the risk of myocardial infarction [85]. In a recent study, Leung et al. evaluated transcriptomic responses to angiotensin II (Ang II) in VSMCs in an attempt to elucidate the gene regulatory networks targeted by Ang II, since these may contribute to the pathogenesis of arterial hypertension. A group of novel lncRNAs were discovered in the Ang II-induced rat VSMCs. One of these lncRNAs was lnc-Ang362, the host transcript of two miRNAs involved in VSMC proliferation (miR-221, miR-222). Remarkably, knockdown of this particular lncRNA by small interfering RNAs significantly reduced VCMC proliferation [86]. Although little is known about the effects of lncRNAs in vascular pathology, these newly identified noncoding transcripts may also be exploited as therapeutic targets in the future [87–89].
As far as biomarkers related to essential arterial hypertension are concerned, Li and colleagues identified a specific miRNA signature in the plasma of hypertensive patients by performing microarray-based miRNA expression profiling (miR-296-5p, let-7e, hcmv-miR-UL112) [17]. Interestingly, one of the miRNAs found to be significantly elevated in plasma samples of hypertensive patients was human cytomegalovirus (CMV) encoded (hcmv-miRUL112). Since the CMV titers were also significantly elevated in hypertensive patients compared to controls, the authors speculated a possible link between essential hypertension and CMV infection, with possible diagnostic and therapeutic consequences (Table 13.1).
13.3.5 Atrial Fibrillation (AF)
Atrial fibrillation is one of the most frequent cardiac arrhythmias encountered in daily practice. It can cause serious complications, including the exacerbation of a preexisting congestive heart failure or thromboembolic events. Recurrent or persistent AF is generally associated with both structural (e.g., fibrosis) and electrical remodeling processes in the atria that synergistically lead to an enhanced cardiac vulnerability (Chap. 50).
The increased rate of impulses during fibrillation causes a high influx of calcium ions, which threatens the integrity of cardiomyocytes. Therefore, an adaptive mechanism involving the decrease of the L-type Ca2+ channel density and the duration of the atrial action potential aims to prevent calcium overload. However, this electrical remodeling process facilitates AF recurrence and maintenance. In this context, Lu et al. [90] hypothesized that miRNAs might regulate calcium channel-encoding genes involved in the process of electric remodeling. Microarray screening and RT-PCR validation experiments showed a significant upregulation of miR-328 in a canine model with tachypacing-induced atrial fibrillation. Moreover, augmented expression of this specific miRNA by both viral delivery and genetic overexpression of precursor miR-328 induced atrial electric remodeling in different animal models. In contrast, loss of function of miR-328 reduced vulnerability for atrial fibrillation and suppressed detrimental remodeling processes. This presumably relies on the ability of miR-328 to target two important genes involved in calcium homeostasis (CACNA1C and CACNB1).
Fibrosis of atrial tissue is a hallmark of atrial fibrillation. miR-21 plays an important role in ventricular fibrotic remodeling by targeting Sprouty-1, a negative regulator of MAP kinase, thus stimulating fibroblast survival [35]. Therefore, miR-21 has been regarded as a possible candidate regulator of atrial fibrosis as well. In atrial tissue samples of myocardial infarction rats, increased levels of miR-21 were associated with a dysregulation of its cognate genes (e.g., Sprouty-1, collagen-1, collagen-3). Anti-miR-21 treatment not only reduced atrial miR-21 expression levels in vivo but also remarkably decreased the duration of atrial fibrillation as well as the amount of fibrotic tissue in the atria [91]. These results suggest that the manipulation of miR-21 expression levels may be beneficial in patients suffering from atrial fibrillation.
13.4 Concluding Remarks
At present, the development of diagnostic and therapeutic options constitutes a promising avenue of miRNA research in cardiovascular medicine. Over the last few years, genetic and pharmacological approaches relying on different chemistries have been developed, in order to enable miRNA manipulation in several small and large animal models that closely simulate human cardiovascular disease. The experience gathered from extensive work carried out on these animal models suggests that miRNA-targeting therapies may prove extremely useful especially with regard to remodeling processes, such as fibrosis, angiogenesis, apoptosis, hypertrophy, and atherogenesis, all initiated by distinct cardiovascular stressors. Such stressors include ischemia and I/R injury, pressure or volume overload, inflammation, and an abnormal electrical activity. The ability to positively influence detrimental remodeling processes may hinder the progression of cardiovascular disease (e.g., reduce scar size or stimulate angiogenesis in border zones in the case of myocardial infarction), thus preventing the occurrence of heart failure. Obviously, the clinical efficacy, long-term treatment effects, and safety of miRNA-based therapies need to be carefully assessed in the forthcoming years, to exclude toxic and other adverse effects. On the other hand, miRNAs hold great promise for the field of cardiovascular biomarker development as demonstrated by a wide range of studies on coronary artery disease, myocardial infarction, and heart failure. Multivariate miRNA signatures may surpass current gold standards or add to their diagnostic power. Moreover, clinical correlations between miRNA profiles and patients’ outcome and prognosis tend to be in the focus of recent studies. Although much remains to be learned about this class of regulatory RNAs, the considerable progress made in understanding miRNA biology over the past 10 years allows for quite some optimism.
< div class='tao-gold-member'>
Only gold members can continue reading. Log In or Register a > to continue
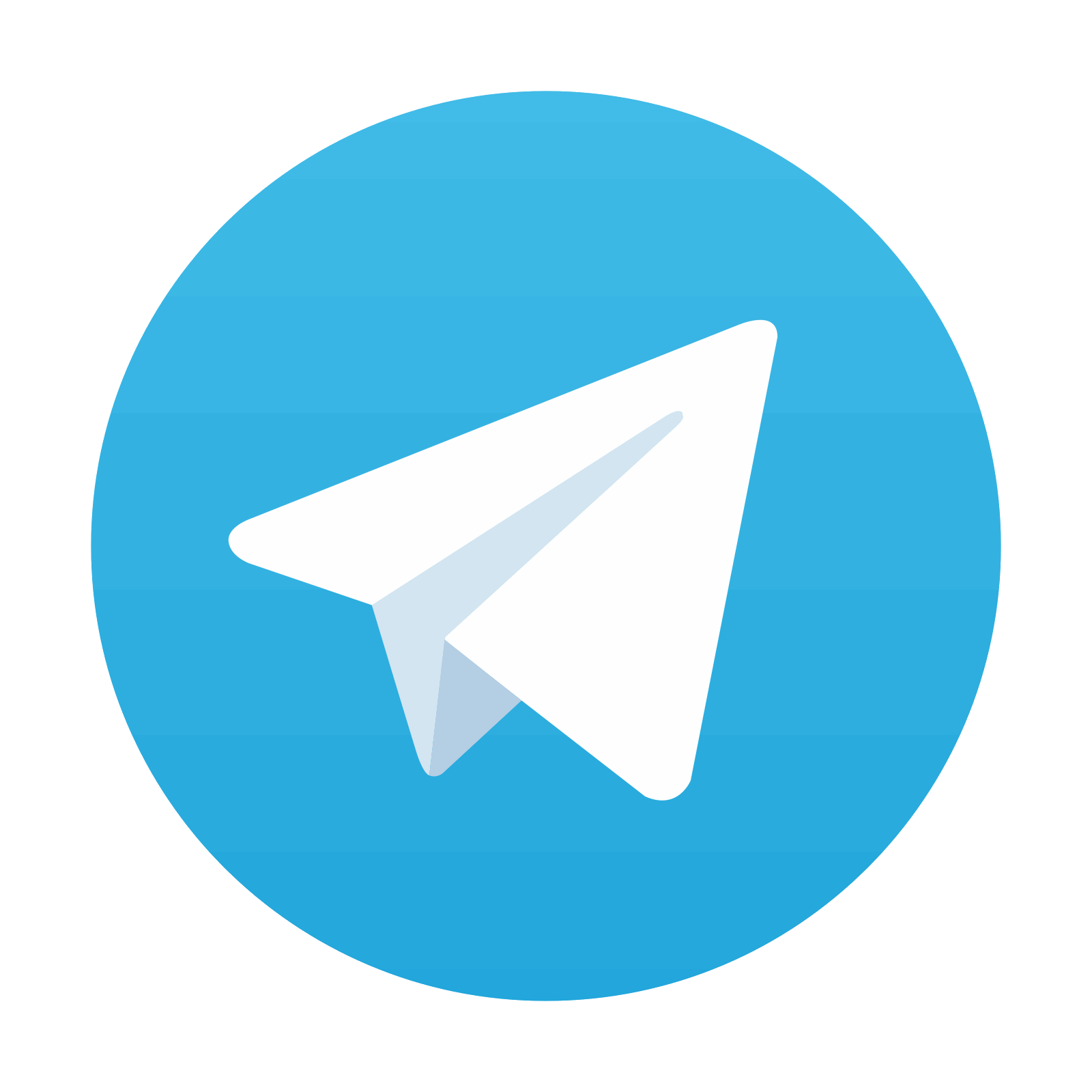
Stay updated, free articles. Join our Telegram channel

Full access? Get Clinical Tree
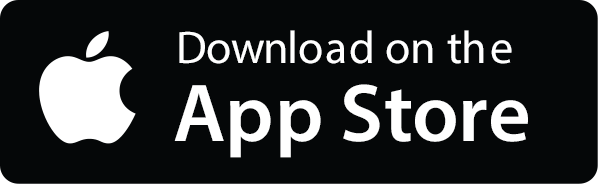
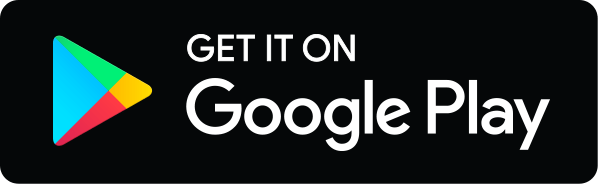