Fig. 19.1
Diagram outlining the considerable overlap between the coagulatory, fibrinolytic, and matrix metalloproteinase (MMP) systems
Table 19.1
MMP production and activation and substrates
MMP | Alternative name | Produced by | Activated by | Substrate – vessel wall | Substrate – blood | Other | References |
---|---|---|---|---|---|---|---|
MMP-1 | Collagenase-1, interstitial collagenase | Smooth muscle cells, endothelial cells, macrophages, monocytes, platelets | MMP-3, -7, -12, plasmin, activated platelets | Col1, col2, col3, col7, col8, col10, col11, gelatin, laminin, vitronectin, aggrecan, tenascin, fibronectin, perlican | TFPI, fibrin(ogen), a1-AT, a2-MG | (Pro-)IL-1b, pro-MMP-1, -2, pro-TNF-a, Complement 1q | |
MMP-2 | Gelatinase-A | Smooth muscle cells, endothelial cells, macrophages, monocytes, neutrophils, fibroblasts, platelets, cardiomyocytes | MMP-11, -14, -15, -16, ROS, thrombin, FXa, uPA, tPA, neutrophil elastase | Col1, col3 col4,col5, col7, col10, col11, laminin, elastin, gelatin, fibronectin, chondroitin sulphate, decorin, osteonectin, aggrecan, vitronectin | Fibrin(ogen), thrombin, a1-AT, plasminogen | (Pro)-IL-1b, IL-2 receptor-a, endothelin, adrenomedullin, platelet integrins, pro-TGF-b, pro-TNF-a, pro-MMP-1, -2, -13, Complement 1q, monocyte chemoattractant protein-3, fibroblast GF receptor | |
MMP-3 | Stromelysin-1 | Smooth muscle cells, endothelial cells, macrophages, monocytes, cardiomyocytes | Plasmin, cathepsins, mast cell proteases | Broad range | Fibrin(ogen), PAI-1, a2-AP, uPA, plasminogen, a1-AT | Pro-MMP-1, -3, -7, -8, -9, -13, E-cadherin, pro-IL-1b, pro-heparin-binding EGF-like GF, pro-TNF-a, Complement 1q | |
MMP-7 | Matrilysin-1, PUMP-1 | Macrophages, monocytes, fibroblasts epithelial cells | Plasmin, cathepsins, mast cell proteases | Broad range | Fibrin(ogen), TFPI, plasminogen, a1-AT | Pro-a-defensin, Fas ligand, pro-TNF-a, E-cadherin, RANK ligand, heparin-binding EGF-like GF | |
MMP-8 | Collaginase-2, neutrophil collagenase | Smooth muscle cells, endothelial cells, macrophages, monocytes, neutrophils | Col1, col2, col3, col7, col10, gelatin, tenascin, aggrecan | Fibrinogen, α1-AT, α2-MG | Pro-MMP-8, complement 1q | ||
MMP-9 | Gelatinase-B | Smooth muscle cells, endothelial cells, macrophages, monocytes, neutrophils, platelets | Plasmin MMP-3, -7, -11, -12, tissue kallikrein, nitric oxide, ROS, cathepsins, mast cell proteases, uPA, tPA | Col1, col4, col5, col7, col10, col11, col14, laminin, elastin, gelatin, osteonectin, galactin-3, fibronectin, aggrecan, vitronectin | TFPI, fibrin(ogen), α1-AT, plasminogen, other serpins, α2-MG | Angiogenic fragments, (pro)-IL-1β, IL-2 receptor-α, IL-10, vascular endothelial GF, (pro-)TGF-β, intercellular adhesion molecule-1, pro-TNF-α, Complement 1q, IL-2 receptor-α | |
MMP-10 | Stromelysin-2 | Endothelial cells, monocytes, fibroblasts, NK-cells, T-cells, chondrocytes | MMP-11, plasmin, plasma kallikrein, trypsin, neutrophil elastase | Broad range | Fibrinogen | Pro-MMP-1, -7, -8, -9 | |
MMP-11 | Stromelysin-3 | Smooth muscle cells, endothelial cells, macrophagesa, carcinomas, placenta, uterus | Activated intracellularly | Very weak | PAI, α2-AP, α2-MG, AT, other serpins | ||
MMP-12 | Macrophage metalloelastase | Macrophages, monocytes, chondrocytes, osteocytes | Plasmin, cathepsins, mast cell proteases | Col1, col4, elastin, fibronectin, laminin, aggrecan, gelatin | FXII, fibrinogen, TFPI, plasminogen, α2-MG, α1-AT | Pro-TNF-α | |
MMP-13 | Collagenase-3 | Smooth muscle cells, endothelial cells, macrophages, fibroblasts, cardiomyocytes | MMP-2, -14, -15, -16, plasmin, trypsin | Col1, col2, col3, col6, col7, col9, col10, col14, osteonectin, perlican, fibronectin, gelatin, aggrecan, tenascin | FXII, fibrinogen, α2-MG | Pro-MMP-9, -13, complement 1q | |
MMP-14 | MT1-MMP | Endothelial cells, macrophages, monocytes | Activated intracellularly | Col1, col2, col3, laminin, gelatin, fibronectin, vitronectin, aggrecan | FXII, fibrin(ogen), α2-MG, α1-AT | Pro-MMP-2, -13 | |
MMP-15 | MT2-MMP | Smooth muscle cells, endothelial cells, T-cells, NK-cells | Activated intracellularly | Gelatin, fibronectin, vitronectin, collagen, aggrecan, tenascin, perlican | Transglutaminase | Pro-MMP-2, (pro-)TNF-α, LRP | |
MMP-16 | MT3-MMP | Smooth muscle cells, endothelial cells, macrophages, T-cells | Activated intracellularly | Col3, gelatin, fibronectin, vitronectin, collagen, aggrecan | α2-MG, α1-AT, LRP | Pro-MMP-2 | |
MMP-17 | MT4-MMP | Endothelial cells, macrophages, monocytes, B-cells | Activated intracellularly | Gelatin | Fibrin(ogen), LRP | Pro-MMP-2 | [2] |
MMP-19 | RASI (rheumatoid arthritis synovial inflammation) | Smooth muscle cells, endothelial cells, macrophages, monocytes, activated lymphocytes, keratinocytes | Col1, col4, laminin, basement membrane, fibronectin, aggrecan, cartilage oligomeric matrix protein, gelatin | Fibrin(ogen) | |||
MMP-20 | Enamelysin | Tooth enamel | Amelogenin, aggrecan, cartilage oligomeric matrix protein | ||||
MMP-23 | Cysteine array (CA)-MMP | Monocytes, foam cells, fibroblasts, epithelial cells, chondrocytes | Laminin, gelatin | ||||
MMP-24 | MT5-MMP | Brain specific | Activated intracellularly | Gelatin, fibronectin, vitronectin, collagen, aggrecan | pro-MMP-2 | ||
MMP-25 | MT6-MMP | Peripheral blood leukocytes | Activated intracellularly | Col4, gelatin, laminin, fibronectin | Fibrin | Pro-MMP-2 | |
MMP-26 | Matrilysin-2, endometase | Endometrium | Col4, fibronectin, gelatin | α1-AT, fibrinogen | MMP-9 | ||
MMP-27 | C-MMP, MMP-22 | Fibroblasts | Gelatin, casein | Autolysis | [11] |
MMP activity is regulated at several levels: gene induction, vesicle trafficking, secretion (not all MMPs), and proform activation. MMPs are also regulated by their differential temporal and spatial expression. They can form complexes with their specific inhibitors, the tissue inhibitors of MMPs (TIMPs), but plasma proteases, including a2-macroglobulin (a2-MG), can also inhibit MMPs [2, 4, 11, 12]. While many MMPs are expressed as inactive pre-proforms, the membrane-type MMPs (MMP-14, -15, -16, -17) and MMP-11, -21, -23, and -27 are activated during processing along the endosomal pathway [2, 11]. Pro-MMPs can be activated by many processes, including chemical (via reactive oxygen species or nitric oxide, for example) and biological means (e.g., GFs, MMPs, or plasma proteinases) [2–4, 11].
MMPs in Peripheral Blood
Most of the components of blood and the vessel wall are capable of producing or interacting with MMPs. Culture studies suggest that MMP-1 expression may be increased during hyperglycemia, while diabetes is also associated with altered MMP gene expression [16, 17]. Patients with type 2 diabetes have elevated levels of MMP-2 and TIMP-1 protein, but not MMP-9, with MMP expression independent of age, duration of diabetes, blood pressure, or serum lipid concentration [16], while both MMP-2 and -9 are elevated in serum from patients with type 1 diabetes [17]. Several studies have measured MMP levels in plasma and serum, but since the absolute concentration of various MMPs is now known to be dependent on how the blood sample was collected and stored [18], the accuracy of many measurements is uncertain. Nevertheless, it seems that there are localized differences in MMP levels in plasma due to differences in the secretion of MMPs from the cells of the blood (predominantly platelets, neutrophils, monocytes) and the artery wall (endothelial cells, smooth muscle cells, macrophages, fibroblasts). These different concentrations of proteases, in conjunction with the differing numbers of platelets, neutrophils, and erythrocytes, result in differential lysis of the clot. Platelet-rich (“arterial” or “white”) clots contain fibrin fiber that are more resistant to fibrinolysis and retract more strongly than platelet-poor (“venous” or “red”) clots [19]. Both platelet-rich and platelet-poor clots can be found in veins [1]. Thus, fibrinolysis in venous thrombi may be heterogeneous, leaving channels in the clot (“recanalization”) through the platelet-poor regions [19, 20].
Origin of MMPs in the Blood and Vasculature
Platelets contain the gelatinases MMP-2 and -9 in their cytoplasm and within platelet granules [21], but these MMPs can exhibit contradictory effects in thrombus formation. MMP-2 stimulates platelet aggregation, with maximal release at maximum platelet aggregation. MMP-9, however, opposes the MMP-2 effect by inhibiting aggregation. Its release from platelets is maximal at 30 % aggregation [22]. MMP-1 and -3 have also been identified in platelets, as have TIMP-1 and -4; the presence of MMP-1 is thought to prime the platelets for aggregation, while MMP-3 has no effect on aggregation [21, 23]. Platelet adhesion results in the release of MMP-1 and -2, with possible activation of the pro-MMP-1 by the platelets [21, 23]. During the onset of thrombosis, platelets release tissue factor (TF) from their a-granules to complex with FVIIa and initiate coagulation. However, platelets also secrete TFPI, which antagonizes the initiation of coagulation (Fig. 19.1). MMP-1, -7, -9, -13, and neutrophil surface proteases are all capable of degrading TFPI, thus allowing coagulation progression [2, 8, 13, 19].
Neutrophils secrete MMP-2, -8, and -9 from various storage granules, as well as cathepsins and other matrix proteases [2, 13, 19, 24]. MMP-2 and -9 are released from gelatinase granules within neutrophils [19] to become activated and influence coagulation and fibrinolysis (Fig. 19.1). MMP-2, -3, -7, -9, and -12 can cleave plasminogen to produce angiostatin, an angiogenesis inhibitor, while further angiogenesis inhibitors are produced from cleaved collagens. Other matrix components, released from the ECM after protease activity, can contribute to the inhibition of coagulation [4, 25, 26]. Neutrophil elastase, a serine protease, is also important in thrombus formation and resolution. It has the capacity to control fibrin generation (altering clot stability by inactivating TFPI) and to degrade fibrin (both directly and indirectly via its effect on plasminogen activation) [19, 24]. Neutrophil elastase cleaves plasminogen to form mini-plasminogen, which is readily activated to form mini-plasmin, without need of any cofactors. Mini-plasmin is more efficient at degrading cross-linked fibrin than normal plasmin and is considerably less sensitive to a2-antiplasmin (a2-AP) inhibition [19], but many MMPs can degrade fibrinogen independently of plasmin [4]. Plasmin-degraded clots disassemble abruptly, releasing large particles into the circulation, possibly resulting in thromboemboli. Elastase-degraded clots release soluble (not particulate) products into the blood [19, 20]. These differences in thrombus stability and plasmin activity will have a significant influence on thrombus resolution in blood vessels.
Monocytes rapidly upregulate their production of MMP-9 on contact with endothelial cells, matrix components (including collagen), fibrinogen, or inflammatory mediators [such as tissue necrosis factor-a (TNF-a), lipopolysaccharide (LPS), or oxidised low-density lipoprotein (LDL)] [2, 27, 28]. MMP-12, -14, and -16 (but not MMP-1 or -3) production in monocytes is also upregulated by inflammatory cytokines, while contact with CD40 ligand upregulates MMP-1, -3, -8, -9, and -11 expression [2, 29]. Interestingly, monocytes treated with an MMP-9 inhibitor lose the ability to aggregate upon contact with fibrinogen (despite fibrinogen’s ability to activate proinflammatory pathways in monocytes), suggesting the presence of an autocrine mechanism in this process [27]. Monocytes also express MMP-10, -19, and -23 [2, 28]. These changes in monocyte MMP production are thought to facilitate entry of these cells into the vessel wall, where they can become macrophages. Tissue macrophages express MMP-13, -14, and -16, while foamy macrophages (macrophages that have migrated into the vessel wall and have become lipid-laden) have upregulated production of MMP-1, -3, -8, -9, -13, and -23. Both foamy and non-foamy macrophages constitutively secrete TIMP-1, -2, and -3 [2, 29–34] (also personal observations).
The vessel wall is lined by thrombo-resistant endothelial cells, which have constitutive MMP-2 expression. MMP-1, -3, -8, -9, and -11 levels are upregulated by inflammatory cytokines, or exposure to macrophages, oxidised LDL, or CD40 ligand [2, 29]. MMP-1 and -2 expression may also be upregulated by high glucose levels [2]. These results suggest that inflammation and immune mechanisms are associated with MMP production by endothelial cells. Endothelial cells also express MMP-13 and the inhibitors TIMP-1 and -2, which are not responsive to oxidised LDL. TIMP-2, however, may be upregulated in response to CD40 ligand [2, 35], while TIMP-1 is induced by prostaglandins and a wide variety of cytokines, including transforming GF (TGF)-β and platelet-derived growth factor (PDGF) [36].
Vascular smooth muscle cells constitutively express MMP-2, production of which is further upregulated by stretch and reactive oxygen species; MMP-9 expression can also be upregulated [2, 37, 38]. Smooth muscle cells appear to be subject to immune activation, as the presence of inflammatory cytokines such as interleukin-1 (IL-1), IL-4, and TNF-a, or contact with activated T-cell membranes (or CD40 ligand) induce the smooth muscle cells to produce MMP-1, -3, -8, and -9 [2, 29]. Smooth muscle cell expression of MMP-11 and TIMP-1, -2, and -3 appear to be upregulated by fibrogenic cytokines such as TGF-b and PDGF [2, 39].
Role of MMPs in Coagulation, Thrombosis Formation, and Fibrinolysis
Every member of the MMP family is capable of degrading components of the vascular ECM, but their substrates are not limited to matrix. Many MMPs are active in the propagation and resolution of thrombosis (Table 19.1).
MMPs in Haemorrhage
The gelatinases, MMP-2 and MMP-9, have been implicated in aneurysm formation, acute neurovascular events, and unstable atherosclerotic plaque structure [40]. Elevated MMP-9 levels in plasma have also been associated with cerebral haemorrhage and perihaematomal oedema, with a negative association of the MMP-9 inhibitor TIMP-1 [41]. Patients with acute intracerebral haemorrhage also have more MMP-3, which is associated with increased mortality [41]. This increase in MMP-3 production may occur through the interaction of tPA and low-density lipoprotein receptor-related protein (LRP) on endothelial cells [42]. Animal studies support these findings, as MMP-9 null mice also have increased incidence of cerebral haemorrhage and oedema, with increased mortality [43]. There is at least partial compensation for the missing MMP-9 in knockout animals by an increase in MMP-2 and MMP-3 levels in areas of cerebral haemorrhage. A similar compensatory increase in MMP-2 expression has also been observed in vein grafts in MMP-9 null mice [44].
MMPs in DVT Resolution
Venous thromboembolism [deep vein thrombosis (DVT) and pulmonary embolism] is a common cause of cardiovascular-associated mortality and is associated with thrombi that are rich in fibrin [1]. DVT resolution often involves re-canalisation (clot retraction, fibrinolysis, and angiogenesis) and infiltration by leukocytes and other cells [45, 46]. The first leukocytes to appear in the thrombi are neutrophils and monocytes, both which can express high levels of MMPs (particularly after activation) [12, 28]. Recently, a study was performed examining patients presenting with DVTs, with blood samples being taken immediately and 6 months later. Compared with healthy controls, patients with DVTs had four to fivefold more MMP in their blood, with an increase in inflammation (as indicated by increased Toll-like receptor-4 gene expression in leukocytes) [47]. Unsurprisingly, patients with thrombi also had more fibrin D-dimer (an indicator of ongoing thrombosis and fibrinolysis [46, 48]) and P-selectin (an indicator of endothelial cell activation [46]) in the initial blood sample [47]. In vivo studies suggest that DVT resolution involves the production of fresh matrix, requiring MMP production and activation and production of pro-fibrotic GF by invading leukocytes (from the blood) and smooth muscle cells and fibroblasts (from the vessel wall) [45, 46]. These results support two hypotheses: that thrombosis is linked to inflammation, and that DVT resolution, like wound healing, requires remodelling [12, 46]. While venous thrombolysis is predominantly mediated by plasminogen activation, at least part of the efficacy of plasmin is due to its ability to activate many MMPs [9, 12]. Thus, successful thrombus resolution is associated with active matrix remodelling consisting of early collagenolysis, elastolysis, and gelatinolysis (predominantly due to MMP-2, -8, -9, and -14 being produced by cells within the thrombus rather than from the circulation), followed by fibrosis [12, 45, 46].
< div class='tao-gold-member'>
Only gold members can continue reading. Log In or Register a > to continue
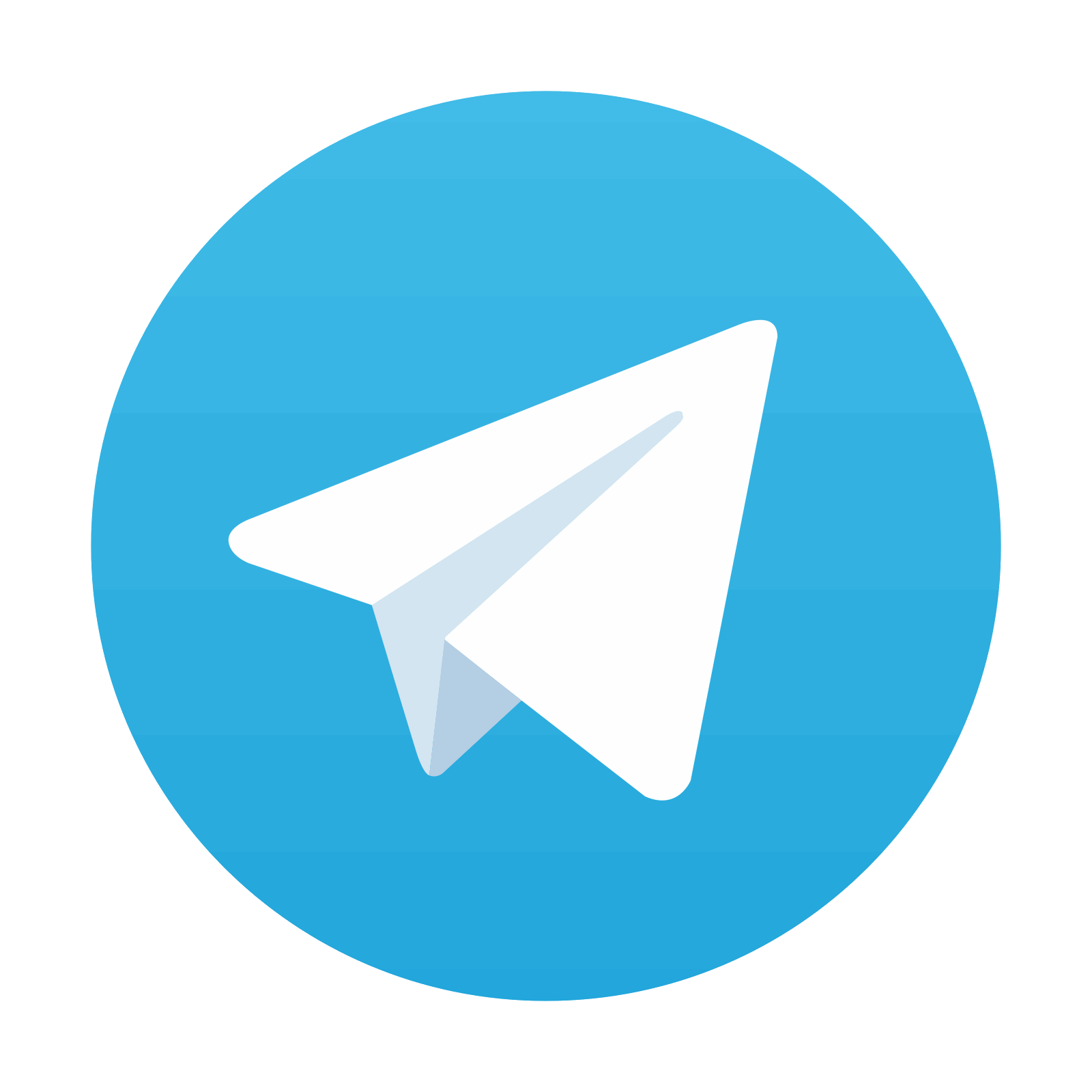
Stay updated, free articles. Join our Telegram channel

Full access? Get Clinical Tree
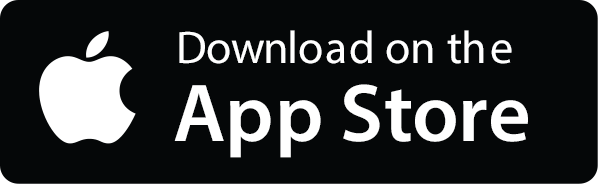
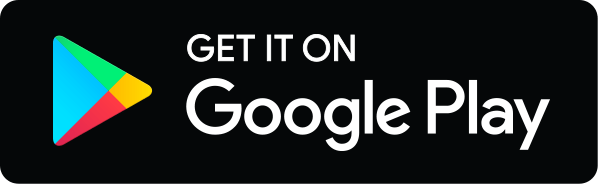