Marker
Expression
Function
Wilm’s tumor1 (WT1) is a zinc finger transcription factor
WT1 is expressed in visceral and parietal mesothelial cells, kidney podocytes, and glomerular capillaries
WT1 controls organ formation (Kreidberg et al. 1993). Loss of WT1 results in kidney and spleen agenesis as well as reduced cardiomyocyte mass and lung malformation (Scholz and Kirschner 2005; Moore et al. 1999). In human lungs, bi-allelic Wt1 mutations are associated with pulmonary dysplasia and mesothelioma (Dharnidharka et al. 2001; Loo et al. 2012)
Mesothelin is a 40 kDa membrane glycoprotein
Mesothelin is expressed in pleura, pericardium, and peritoneum
To date, the biological function of mesothelin is not known; however, the cleaved fragment that is released from the mesothelin transmembrane protein, called megakaryocyte-potentiating factor, is currently being evaluated as a marker for mesothelial malignancies (Chang and Pastan 1996; Hassan et al. 2004)
Leucine-rich repeat transmembrane neuronal–4 protein (LRRN4) is a 67 kDa transmembrane protein
LRRN4 is expressed in primary mesothelial cells and is significantly down-regulated in mesotheliomas
To date, its function is not known. LRRN4 is up-regulated in acute lung injury and in chronic obstructive pulmonary disease (Kanamori-Katayama et al. 2011)
Uroplakin 3B is a membrane glycoprotein
Uroplakin 3B is expressed in urothelial cells, retinal pigment cells, and mesothelial cells of the peritoneum, pleura, and pericardium
Sulfatase 1 (Sulf1) is a heparan sulfate 6-O-endosulfatase enzyme
Sulfatase1 is expressed in mesothelial cells lining the lung surface and Sertoli cells of testes
This enzyme removes 6-O sulfate groups from heparan sulfate. Sulfatase1 modulates heparan sulfate by regulating its binding partners. In Sertoli cells of the testes, Sulfatase1 is regulated by WT1. (Langsdorf et al. 2011)
(Raldh2) Retinaldehyde dehydrogenase 2 mRNA encodes for an enzyme that belongs to the aldehyde dehydrogenase family
Raldh2 mRNA is expressed in mesodermal and mesothelial cells
This enzyme is required for the production of retinoic acid. Loss of Raldh2 causes cardiovascular defects and embryonic lethality in mice. It plays a crucial role in lung bud formation (Desai et al. 2006)
Calretinin is a 29 kDa intracellular calcium-binding protein
Calretinin is expressed in the central and peripheral nervous system, retina, mesothelium, adipocytes, and Sertoli cells of testes
Calretinin is a calcium-binding protein involved in many signal transduction pathways. It is up-regulated in mesotheliomas and adenocarcinomas (Barberis et al. 1997)
10.4 Mesothelial Progenitor Cell Function
Evidence that the mesothelium gives rise to other cell lineages was originally derived from in vitro observation of cultured cells. In these studies, it was found that mesothelial cells “trans-differentiate” to distinct mesenchymal phenotypes such as fibroblasts and smooth muscle when exposed to defined growth factors (Yang et al. 2003). Dye labeling studies and quail-chick chimera experiments showed that the epicardial mesothelium differentiates into endothelium and smooth muscle through an EMT process during embryonic development (Pérez-Pomares et al. 2002). Examination of WT1 genetically deficient mice provided further evidence that mesothelial cells are progenitors required for organ formation (Kreidberg et al. 1993).
Mice expressing Cre-recombinase under mesothelial-specific promoters are powerful tools that have been utilized to study mesothelial progenitor function. Table 10.2 lists the currently available mesothelial-Cre mouse strains that can be used for lineage analysis. Using a mouse that expresses an inducible Cre-recombinase from the endogenous Wt1 locus (Zhou et al. 2008), mesothelial cells lining fetal heart, gut, and liver were shown to migrate inward and contribute to differentiated mesenchymal cells during organogenesis. To date, mesothelial-derived cell types identified by this methodology include cardiomyocytes, airway smooth muscle, vascular smooth muscle, endothelium, hepatic stellate cells, fibroblasts, perivascular mesenchyme, and gut tube anlage (Zhou et al. 2008; Que et al. 2008; Wilm et al. 2005; Asahina et al. 2011; Winters et al. 2012; Dixit et al. 2013). More recently, mesothelial cells were shown to give rise to visceral adipocytes (Chau et al. 2014).
Table 10.2
Mouse lines used to lineage trace mesothelial cells
Mouse strain | Mesothelium-derived lineages |
---|---|
WT280Cre YAC (Wt1 Cre ) Transgenic mouse line (Wilm et al. 2005) | Gut—vascular smooth muscle and endothelial cells (Wilm et al. 2005) |
Lung—vascular smooth muscle (Que et al. 2008) | |
Heart—smooth muscle cells and endothelial cells (Wilm et al. 2005) | |
Wt1 CreERT2/+ Loss-of-function WT1 allele resulting from a knock-in TAM-inducible Cre recombinase gene in exon 1 of WT1 (Zhou et al. 2008) | Heart—epicardium, cardiomyocytes, vascular smooth muscle cells, endothelium (Zhou et al. 2008) |
Liver—hepatic stellate cells, myofibroblasts, perivascular mesenchyme (Asahina et al. 2011) | |
Lung—vascular smooth muscle, bronchial smooth muscle, desmin+ fibroblasts (Dixit et al. 2013) | |
Wt1 GFP–Cre Loss-of-function Wt1 allele resulting from a knock-in eGFP–Cre fusion construct inserted in exon 1 of WT1 (Zhou et al. 2008) | Off-target Cre recombination throughout embryo, limits use as a tool for lineage analysis. However, fidelity of GFP supports use for cell sorting of mesothelial cells |
Wt1/IRES/GFP–Cre | Intestine and gut—endothelium, smooth muscle, visceral musculature, pericytes, Cajal cells (Carmona et al. 2013) |
Lung—pulmonary endothelial, smooth muscle cells, bronchial musculature, tracheal and bronchial cartilage, CD34+ fibroblast-like interstitial cells (Cano et al. 2013) | |
Msln CLN–CreERT2/+ TAM-inducible Cre recombinase–IRES–LacZ knocked into Mesothelin (Msln) locus (Rinkevich et al. 2012) | Msln+ cells give rise to fibroblasts and vascular smooth muscle in the lung, intestine, liver, gut, and thymus (Rinkevich et al. 2012) |
10.4.1 Mesothelial Progenitor Cells Lining the Lung
In the lung, independent studies using different Cre-lox lineage tracing system found conflicting results regarding the contribution of fetal lung mesothelial progenitors to differentiated lung cell compartments (Que et al. 2008; Greif et al. 2012, Rinkevich et al. 2012; Dixit et al. 2013; Cano et al. 2013). One study used a non-inducible Wt1–Cre (WT280Cre YAC) transgenic mouse line and showed that the mesothelium gives rise to intra-pulmonary artery smooth muscle cells (Que et al. 2008). These results were confounded, however, by uncertainties regarding the strength, timing, and specificity of the cellular marking in this transgenic Cre line. The second study, which focused on lineages in the main pulmonary artery, used an inducible knock-in Wt1 CreERT2/+ line and showed that the mesothelium is not a significant source of smooth muscle cells for this structure (Greif et al. 2012). Utilization of Wt1/IRES/GFP–Cre transgenic mouse line (Table 10.2) showed that mesothelial cells lining the lung surface were found to contribute to endothelial cells, smooth muscle, bronchial musculature, tracheal and bronchial cartilage, as well as CD34+ fibroblast-like interstitial cells (Cano et al. 2013). Like previous studies, these findings may be similarly limited by uncertainties in the fidelity of Cre activation. Finally, a study employing the Msln CLN mouse that contains an inducible CreERT2 knocked into the mesothelin locus showed that fibroblasts and smooth muscle are derived from mesothelin+ cells. One potential concern of this study is that mesothelin expression in the embryonic lung may not be mesothelial-specific (Rinkevich et al. 2012; Dixit et al. 2013).
In order to clarify these issues, we employed a Wt1 CreERT2/+ mouse line and conducted a detailed lineage analysis of the early fetal mesothelium during lung development (Table 10.2). The strength of this tool is that, Cre expression can be controlled temporally and parallels endogenous WT1 expression. Before embarking on these studies we first confirmed that WT1 is restricted to the mesothelium only in the developing lung (Dixit et al. 2013). The lineage data showed that mesothelium is a source of a distinct subpopulation of bronchial smooth muscle, vascular smooth muscle, and desmin+ fibroblasts during embryonic and early post-natal lung development. Further, using genetically modified mice and ex-vivo organ cultures, we mechanistically demonstrated that active hedgehog signaling is required for mesothelial cell entry into the fetal lung parenchyma. In contrast, hedgehog function had no apparent role in mesothelial cell entry into the developing heart. Notably, Wnt and retinoic acid signaling were found to control EMT and migration of epicardial cells (von Gise and Pu 2012). In addition, TGF-β was found to induce EMT and migration of mesothelial cells in the developing liver (Li et al. 2013). In culture, hepatocyte growth factor/scatter factor (HGF/SF) was found to regulate migration and proliferation of human mesothelial cells isolated from pleural effusions (Warn et al. 2001). Taken together, these findings further underscore a paradigm in which fetal mesothelial cells are an important source of progenitors for development of mesenchymal structures and show that key signals that underlay the entry and migration of mesothelial cells are organ-specific (Dixit et al. 2013).
10.5 Mesothelial Cells in Tissue Repair and Remodeling
Injury responses in mesothelial cells occur following direct injury to the mesothelium and during injury to the underlying sub-mesothelial tissue. During homeostasis, mesothelia are quiescent; however, they proliferate after direct damage from air, water, foreign substances, intra-coelomic movement of organs, surgical procedures, and peritoneal dialysis (Mutsaers et al. 2000). These injuries cause swelling and sloughing from the surface into the surrounding anatomical space (Herrick and Mutsaers 2004). Mutsaers et al. reported that within 48 h of injury, 30 % of mesothelial cells begin to proliferate and subsequently restore the damaged area completely by 7–10 days (Mutsaers et al. 2000; Ryan et al. 1973). It has also been suggested that free-floating mesothelial cells migrate from the surrounding fluid to the wound site to reestablish tissue integrity (Herrick and Mutsaers 2004). Notably, a prominent pro-inflammatory role for mesothelial cells has been postulated for acute and chronic peritonitis in humans undergoing peritoneal dialysis (Hekking and van den Born 2007; Nocoladi et al. 1994; Devuyst et al. 2010; Yung and Chan 2009).
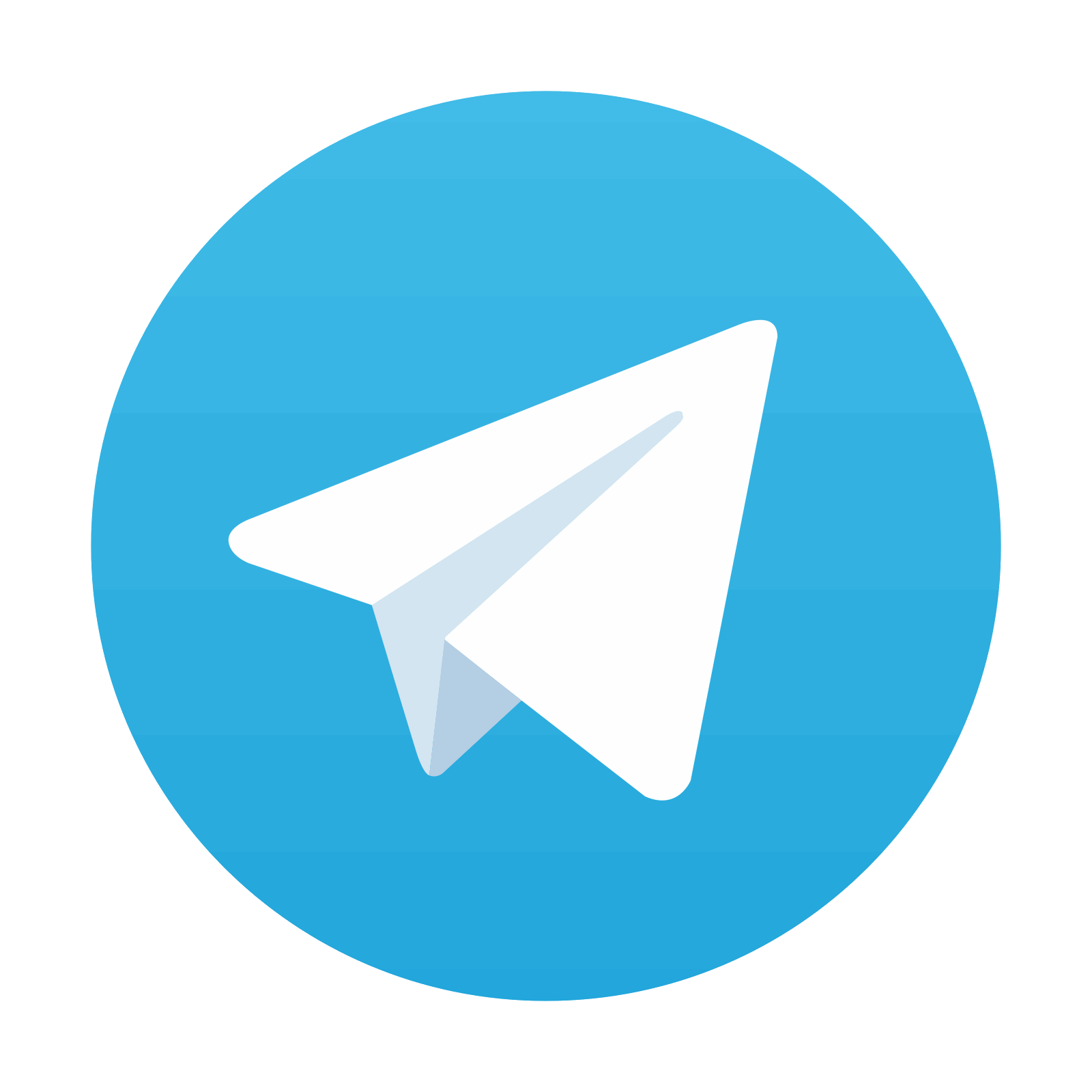
Stay updated, free articles. Join our Telegram channel

Full access? Get Clinical Tree
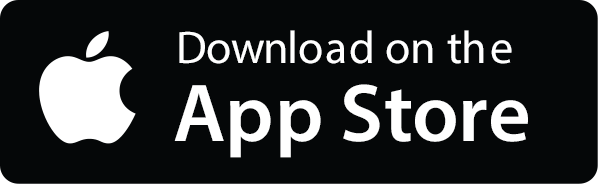
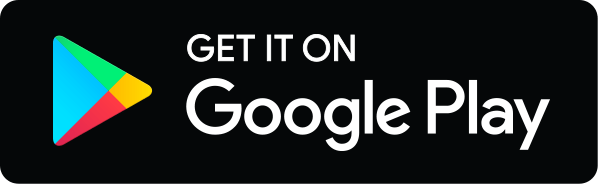