Key Points
- •
Fundamental scientific concepts and pioneering technologic developments have made safe and effective percutaneous cryoablation possible.
- •
Scientific concepts regarding thermal energy, heat transfer, and refrigeration techniques, and their application to the development of cryoablation technology, are reviewed in this chapter.
- •
This chapter includes a historical overview of cryoablation developments at Medtronic CryoCath, including a detailed description of the company’s catheter portfolio.
- •
Future directions in cryotechnology at Medtronic include enhancements, upgrades, and future developments.
Cryo: The Scientific Basis of Cryoablation and Design of Cryoablation Systems
What Is Cryoablation?
Cryoablation is a technique that uses refrigerant to remove heat from living tissue. To understand the process of heat removal, we must review a few basic concepts from physics and define terms such as cold, heat, temperature, and energy.
The Concepts of Heat and Cold
In everyday life, we use words such as cold and heat in various contexts and with various meanings. Generally, cold designates a relative absence of heat. When we talk about temperature, we refer to the relative presence or absence of heat. Three scales are widely used to measure temperature: The Celsius and Fahrenheit scales are both based on the boiling and freezing points of water, whereas the Kelvin scale is based on the absolute zero, that is, the point at which atomic and molecular motion comes to a stop.
Regardless of the phase in which a body exists (solid, liquid, or gas), its particles are in perpetual motion. For solids, motion is mainly vibration at the atomic level; in liquids and gases, motion also occurs at the molecular level. Molecules have potential and kinetic energy. In any given substance, the potential energy depends on the distance between its molecules, whereas the kinetic energy depends on the velocity of the motion of its constituent particles. Heat is a measure of these forms of energy; therefore, the greater the distance between molecules and the greater the velocity of their motion in a given substance, the more heat (or thermal energy) the substance contains.
Thermal Conduction and Convection
Heat transfer is the process by which energy in one body is communicated to another body with which it is in contact. The transfer of heat always occurs from a body with greater potential and kinetic energy to a body of lower potential and kinetic energy, in other words, from high to low temperature. This process can be understood through a simple example of thermal conduction: When you touch an object that is colder than your hand, heat flows from your hand to the object, warming the object up and creating a sensation of cold in your hand.
The phenomenon described earlier (heat flow from high-energy to low-energy molecules) is consistent with the second law of thermodynamics. Heat does not spontaneously flow from a body at lower temperature to a body at higher temperature. Thermal conduction occurs in all forms of media, including solids, liquids, and gases.
Although the principles of heat conduction are based on the transfer of energy in materials by vibrations at the molecular level, heat convection is a macroscopic and observable mechanism of thermal transfer that involves bulk motion of fluids. Heat convection occurs only in liquids and gases, or in mixtures of both. In forced convection, the heat transport is generated by means of an external source. For example, it is well-known that a hot plate of metal will cool faster when exposed to a blowing fan than when exposed to still air. Another mechanism of heat convection is natural convection, which occurs when temperature gradients across a fluid create density gradients.
Refrigeration
Refrigeration is the process of purposefully removing heat from one body and transferring it to another. The classic example is a household refrigerator, where energy is continuously transferred from the inside of the refrigerator to the surrounding environment.
In the refrigeration cycle (illustrated in Figure 7–1 ), a refrigerant enters the compressor as a vapor. It is compressed and then exits the compressor superheated. The superheated vapor travels through the condenser, which cools and condenses the vapor into a liquid. The liquid refrigerant goes through the expansion valve, where its pressure abruptly decreases, causing sudden evaporation and cooling of the refrigerant. Generally, about half of the liquid is vaporized through the valve, resulting in a mixture of liquid and vapor at a lower temperature. The cold liquid–vapor mixture travels farther through the evaporator and is completely vaporized, absorbing heat from the warm air in the space being refrigerated. The resulting refrigerant vapor returns to the compressor and the cycle is repeated.

Phase Change and the Joule–Thomson Effect
Two common refrigeration techniques in the medical technology industry are phase change and the Joule–Thomson effect. In phase-change systems, a cold, saturated liquid, generally nitrogen, is directed to the targeted area of treatment. As the fluid absorbs heat, it undergoes phase change from liquid to vapor and expands more than 400-fold. Given the considerable volume of vapor generated, a large cross-sectional area is required to recover the expanded gas and prevent pressure buildup. Because refrigerant in phase-change systems travels at very low temperature, significant insulation is needed to prevent the freezing of adjacent tissue. These considerations make phase-change systems unsuitable for medical applications where miniaturization is critical.
In Joule–Thomson systems, a fluid (either liquid or gas) is forced to pass through a valve that consists of a small capillary tube, where it loses pressure. This loss of pressure results in a temperature decline of the fluid. Joule–Thomson systems that use liquefied gases, such as nitrous oxide (N 2 O), are able to sustain minimal temperatures less than −80°C. These systems can operate at lower pressures than gas systems (760 vs. 3000 psig [per square inch gauge]), which is a significant advantage when applied to the design of cryoablation catheters. With this lower injection pressure, catheters can be designed with materials that provide greater steerability and flexibility. Finally, because hospitals are already equipped to scavenge N 2 O, these systems are appropriate for widespread use.
Application to Catheter Ablation Therapy
Given their small luminal size, cryoablation catheters can easily be directed through blood vessels into the heart for the minimally invasive treatment of arrhythmias. Cryogenic fluid is delivered into the catheter tip. When the refrigerant used is N 2 O, the fluid undergoes a Joule–Thomson expansion process to achieve the desired low-temperature environment. The creation of a cold environment inside the catheter tip initiates a process of heat extraction from the cardiac tissue to the device, selectively freezing the target tissue with clinical effect.
Refrigeration Cycle in Cryocatheters
A cryoablation catheter system performs the same refrigeration function as a household refrigerator, except it is not a closed-loop system. In the cryocatheter system, the refrigerant is not recompressed and recirculated. The simplified schematic in Figure 7–2 shows the similarities and differences from the closed-loop system outlined in Figure 7–1 .

Thermal Convection in Cryocatheters
In intracardiac cryocatheters, both natural and forced convection play a role in the overall effectiveness of the lesion created at the interface of the catheter tip and target tissue. The blood circulating around the tip of the catheter is a significant source of convection, warming the tip and reducing the amount of heat being removed from the tissue—thus the importance of good contact between the catheter tip and tissue. If the tip of the catheter can be shielded from circulating blood during the cryogenic process, reducing forced convection, more effective lesions can be created. Some natural convection effects are also present because of temperature differences within the pool of blood circulating near the tip.
Design Considerations and Materials
The process of heat flow from tissue to device can be made more effective by applying certain design considerations. For example, a catheter tip material with high thermal conductivity will increase the net transfer of heat to the device. The tip geometry or curvature must be appropriate to ensure steady contact with the tissue; loss of efficiency to a layer of blood that may be present between the tissue and catheter tip may occur. It is also important to establish the cooling power required to promote the effective removal of heat, given the dimensional constraints of the device and convective effects of blood circulating around the tip.
The measure of the ability of a material to conduct heat is defined by its thermal conductivity, k . In general, metals conduct heat better than polymeric materials. Copper is one of the most thermally conductive materials available and is typically selected as the tip material of choice in focal cryocatheters. Gold plating encapsulates the entire tip to ensure biocompatibility for use in the bloodstream. With their low thermal conductivity, polymeric materials are regarded as insulators, not conductors. In cryo devices, they are widely used for catheter parts that are not designed to transfer heat; they are also used in the production of cryoablation balloons, in which their weak ability to transfer heat is compensated by the very thin wall thickness resulting from the blow-molding process.
Additional cryocatheter design considerations include miniaturization and maneuverability to permit navigation of the catheter in and around the heart. Steering and feedback mechanisms must be integrated with the refrigerant delivery and recovery system in a catheter shaft that is flexible and can be pushed and torqued.
In summary, an effective cryoablation system is one that quickly removes heat from tissue. Endocardial treatment with cryocatheters requires the use of sufficient cooling power to offset the convective effects of blood circulation around the catheter tip while still removing enough heat from the tissue to create a clinically relevant lesion. Selection of appropriate cryogens is important, as is the process by which the low-temperature environment is achieved. This must be balanced with additional features in a shaft that can be maneuvered in and around the heart.
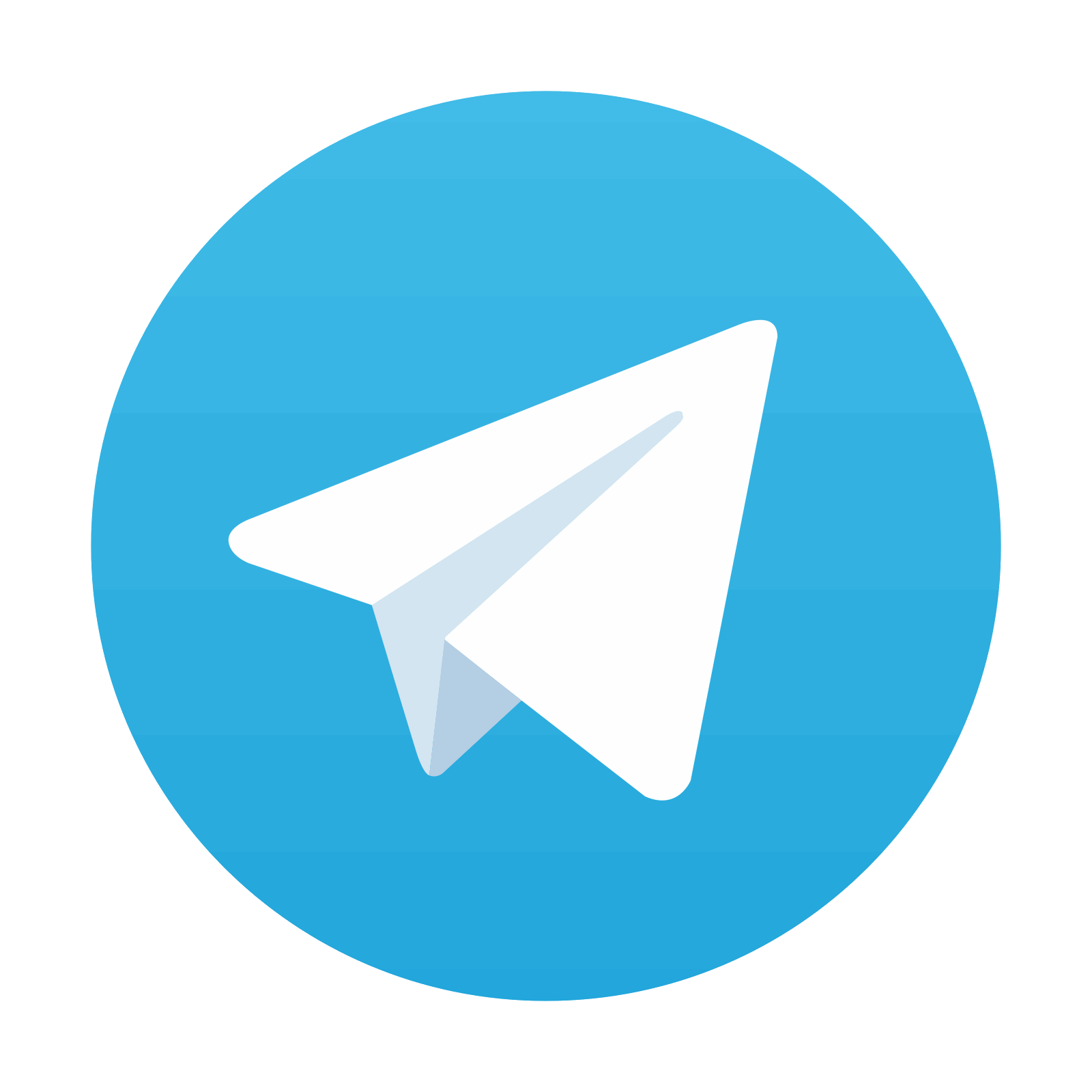
Stay updated, free articles. Join our Telegram channel

Full access? Get Clinical Tree
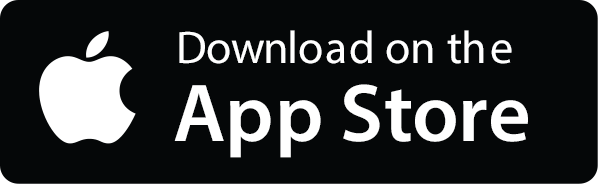
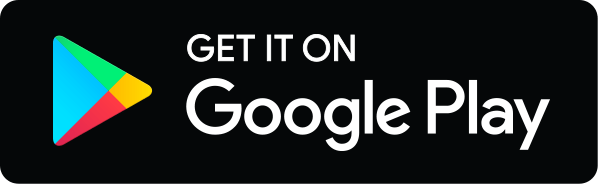