Medical simulation comprises the artificial representation of a real-world medical environment with sufficient realism to facilitate learning through immersion, reflection, feedback, and practice without the risks inherent to a similar real-life experience.1 As a result, simulation’s re-creation of a real-world process/system permits operators to practice/rehearse various scenarios that may range from routine practice to rare events including complications. One central purpose of simulation is for “safe learning,” ie, learning without a real-world consequence if errors occur. As such, simulation has a long history of use in professions that require the execution of precise physical and neurocognitive tasks in high-risk environments. For example, simulation has been used to train pilots and other professions where mistakes can have disastrous consequences. We know from the aviation industry as well as other nonmedical fields that simulators are effective teaching tools, improve learner success, enable repetitive practice of tasks in a range of conditions, and enhance task safety.2-7 Despite the ability of simulators to recreate numerous high-risk and complex environments, the uptake of simulation for the training and assessment of interventional cardiologists has lagged. This chapter will review the use of medical simulation in the realm of interventional cardiology as it stands today in hopes that it will continue to grow and be integrated more effectively into training and practice.
Interventional cardiology is a relatively new field, having only been in existence for just over 3 decades. Many current operators have been practicing since its inception and have vast experience, essentially precluding a need for simulation prior to the turn of this century. Previously, coronary interventions were only being performed in high-volume centers by high-volume operators. However, since Andreas Gruentzig’s first foray into the heart in September 1977, there have been many changes in the landscape of interventional cardiology. No longer are we as a profession performing only coronary interventions. Where previously invasive surgery represented the only option for patients, thanks to countless technologic advancements and the pioneering nature of numerous physicians/scientists/industry partners, patients with structural heart disease, peripheral arterial disease, and coronary disease now have minimally invasive options. However, with the expansion of interventional cardiology beyond the coronary vessels has come the challenge/inability of most interventionalists to practice all 3 subsets of interventional cardiology, ie, coronary, structural, and peripheral. This fact, coupled with demands for “centers of excellence,” along with an ever competitive market further underscore the need for an environment where the interventional cardiologist can assimilate new skills, rehearse rare emergency procedures, and perhaps provide evidence of their own. Simulation can provide such an environment.
In fact, interventional cardiology is a field well suited for simulation.8 Simulation can be used to both educate and improve performance by enabling repetitive practice of tasks in a range of difficult clinical conditions.9-11 Education in an era where work hour restrictions are a reality has also proven challenging. This, in addition to the well-publicized decrease in coronary percutaneous coronary intervention (PCI) volume over the past decade, drug-eluting stents, and appropriate use criteria, has led to a newer generation of interventionalists who will never attain the clinical experience/volume of their predecessors. Compounding this are the constant advances in techniques and devices and the ever present pressure to reduce costs while enhancing quality all within an environment where patient safety is paramount.12 The emergence of hospital departments, national bodies, high-profile conferences, and scholarly journals focused solely on patient safety seeks to serve the growing societal pressure for transparency in medical practice, in concert with public expectations for reductions in medical error.13 Thus, there is a resounding need for a “safe” environment where physicians may practice their current skill set and/or attain new skills without subjecting patients to real harm. The days of “see one, do one, teach one” are gone and perhaps will be replaced with “see one, do several in a simulated environment and then more with a proctor, and then only after a certain volume be permitted to teach one.” Fortunately, technology has also advanced in the realm of medical simulation, and today there are multiple high-fidelity medical simulators available that can provide operators with a wide range of clinical scenarios in all 3 realms of interventional cardiology. Simulation provides a medium by which providers can learn and practice despite explosions in technology and limitations in procedural volume and work hours. Simulation thus provides an answer of how to train, test, and practice in the 21st century where only the highest quality health care will be accepted.
Another application of simulation has been its use to test individuals in a “safe environment.” The Federal Aviation Administration and several other nonmedical professions require simulation as part of routine training and/or maintenance of competency and annual skills training.2 Recently, governing bodies such as the American Board of Internal Medicine (ABIM), the National Board pf Medical Examiners (NBME), and the Accreditation Council for Graduate Medical Education (ACGME) have been investigating the use of simulation to assure procedural competency and patient safety.8 Indeed, the ABIM has incorporated the use of simulation into their Maintenance of Certification program, and the ACGME has mandated that simulation be a current component of all interventional cardiology fellowship training programs. These applications of simulation have come despite a lack of large-scale studies showing improved patient outcomes with simulation in the field of interventional cardiology.
Although the comprehensive review of the evidence of all simulation studies is beyond the scope of this chapter and has been performed elsewhere,9,10,14-16 it is important to review the origins of simulation in procedural fields. The evidence that a simulation-based curriculum is useful and valid in procedural fields originates from the surgical realm, specifically from laparoscopic surgery. In the 1980s, with the advent of minimally invasive laparoscopic surgery followed by a large number of patient complications from this new technique, several credentialing societies developed guidelines on the proper acquisition of laparoscopic skills prior to performing this surgery on real patients.17 Although initially performed in animals, with the advent of newer technologies, simulated laparoscopic training eventually became possible. Scott et al,18 in 2000, clearly demonstrated that a 4-week simulation course on “box simulators” enhanced the performance of laparoscopic cholecystectomy in the operating room.18 To date, there have been 2 large randomized clinical trials19,20 that confirm the ability of a virtual reality simulator to enhance operative speed and economy of movement and decrease technical error. This was further confirmed in a Cochrane review21 that involved 23 trials with over 600 participants. The benefits of virtual reality training extend beyond practicing on a model as the trainee can practice in an educationally oriented and safe environment, at progressively more challenging levels, which leads to a proficiency-based approach to skills acquisition.13 It is a logical extension that if simulation training can be used to enhance the proficiency of surgeons performing a laparoscopic cholecystectomy, such training should be valid in enhancing the skills of an interventional cardiologist.
In this era with heightened emphasis on cost containment, quality, and outcomes, simulation has shown some promise. Although not in interventional cardiology per se, simulation-based training of residents in the proper aseptic insertion of central venous catheters was shown not only to reduce the incidence of catheter-related bloodstream infections, but also to have a net savings of $700,000 from reduction in rates of infections.22 To date, there are no studies that have shown simulation training in interventional cardiology to be able to reduce healthcare costs. However, one can imagine a simulation-based training module for interventional cardiology that would incorporate various aspects of cost-savings measures such as proper guide selection or stent selection, thus precluding the current trial-and-error technique often employed by inexperienced operators.
Diagnostic cardiac catheterization is now over half a century old and has evolved to include both femoral as well as radial approaches. The sheer volume of cardiac catheterizations has made the use of simulation for diagnostic catheterization from the femoral approach limited as fellows in training usually obtain their required procedural numbers to become certified in this procedure. In fact, there is a clear dearth of studies involving this area. Simulation, however, can provide a safe arena in which operators may encounter rare complications of diagnostic catheterization. All of the major simulation vendors have simulations including diagnostic cardiac catheterization, and most provide simulated complications that permit the learner to see these potential complications and how they would treat them in a benign simulated world as well as provide feedback if their treatment was not correct.8
The use of simulation for transfemoral diagnostic catheterization has likely been muted due to the large group of operators well versed in its technique. For transradial catheterization, due to the well-documented “learning curve” that comes with this technique,23 the limited number of highly experienced operators, and the push for increased use due to its enhanced safety, there has been a remarkable interest in the use of simulation. In fact, transradial catheterization is an ideal procedure for simulation, ie, it involves a large group of operators who already have a basic skill set/knowledge base (left heart catheterization) but who must now learn how to approach it differently.8 Simulation provides a venue wherein operators can safely learn to apply their skill set in this new approach. Additionally, there is the opportunity here to use simulation to assess procedural competence, although to date, this has not been studied or validated.
Most of the major simulator companies provide transradial coronary simulations (Table 77-1) and provide users with the opportunity to encounter several unique scenarios including anatomic variants such as radial and/or brachial loops, aberrant origins of the radial artery, and variations in aortic arch anatomy. Commonly encountered issues such as spasm can also be simulated. These simulations also allow operators to learn the behavior of different catheters than those used for transfemoral cases. There are numerous transradial courses currently available that incorporate simulation as part of its curricula, including the Society for Cardiovascular Angiography and Interventions (SCAI)–sponsored Transradial Intervention Program (TRIP), which features expert proctors working with radial trainees on simulators.
Procedure | CAE | MSC | Mentice | Symbionix | |
---|---|---|---|---|---|
Coronary | |||||
LHC | X | X | X | X | |
PCI | Coronary | X | X | X | X |
SVG | X | ||||
FFR | X | ||||
IABP | X | X | X | ||
Rotational Atherectomy | X | ||||
RHC | X | ||||
Radial | X | X | |||
Structural | |||||
Transeptal | X | X | X | ||
PFO/ASD | X | X | X | ||
PABV | X | X | X | ||
PMBV | X | X | |||
Alcohol Septal Ablation | |||||
Evalve | |||||
LAA Closure | X | X | |||
TAVI | X | X | X | X | |
Peripheral | |||||
Carotid | X | X | X | X | |
Renal | X | X | X | X | |
Iliac | X | X | X | X | |
SFA/Popliteal | X | X | X | X | |
Infrapopliteal | X | X | |||
Coiling | X | X | |||
Neuro-coiling | X | X | X | ||
Thrombolytics | |||||
Thrombectomy | |||||
Atherectomy | |||||
TEVAR | X | X | |||
EVAR | X | X | |||
Adjunct Imaging | |||||
IVUS | X | ||||
OCT | |||||
ICE | X | X | X | ||
TEE | X | X | X | X |
Simulation of PCI has also evolved. Currently, numerous simulations of complex clinical scenarios including acute myocardial infarction, shock, multivessel disease, chronic total occlusion, bifurcation lesions, left main intervention, and elective PCI are available. These simulations require operators to manage the whole case including periprocedural pharmacology, assessment and treatment of hemodynamic and electrical instability, and selection of equipment and interventional strategy. For performance assessment purposes, numerous metrics can be measured including catheter selection, advancement, and coronary cannulation, wire manipulation, appropriate device selection, lesion coverage, stent expansion, residual disease, and “fluoroscopic” time.8 In addition to standard PCI equipment, some companies have partnered with various simulation companies and offer device-specific simulations, including rotational atherectomy and distal protection devices for saphenous vein graft intervention (eg, Boston Scientific Corp. with Medical Simulation Corp.).
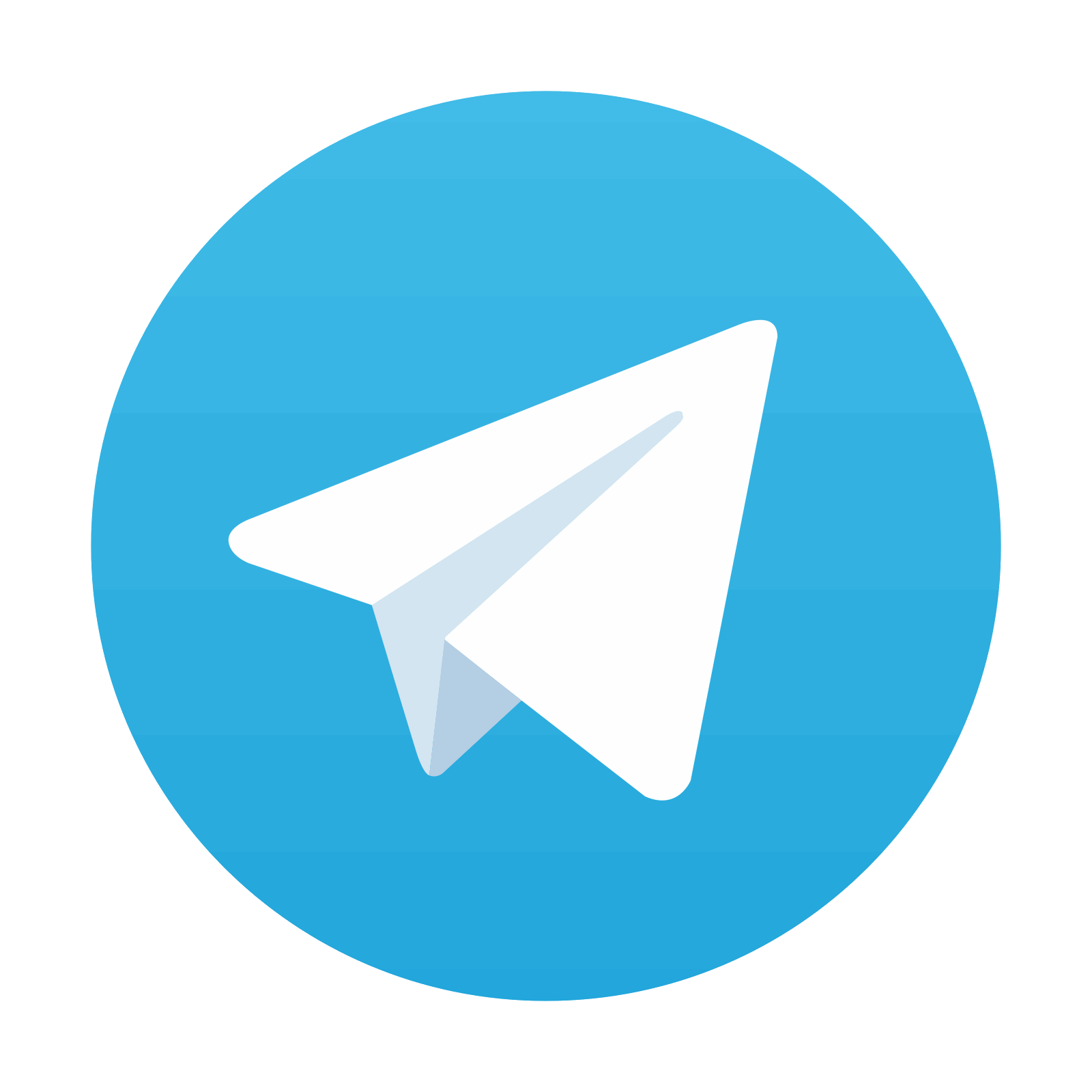
Stay updated, free articles. Join our Telegram channel

Full access? Get Clinical Tree
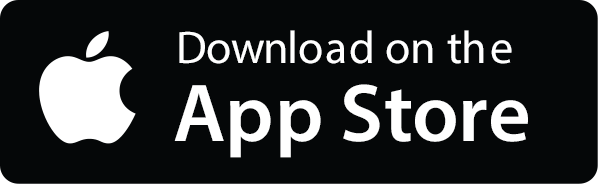
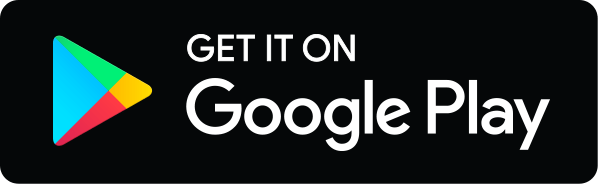