Fig. 28.1
Thoratec HeartMate II Left Ventricular Assist Device (LVAD). Left; LVAD system complete with external controller connected to the LVAD via a percutaneous drive line with power input from batteries suspended in a holster. The pump is located within the extraperitoneal space. Right; Impeller generated unidirectional axial flow (arrows) (Images courtesy of Thoratec (Pleasanton, CA))

Fig. 28.2
Heartware HVAD Left Ventricular Assist Device. Left; HVAD with system controller and battery packs affixed to patient belt. Note position of HVAD directly opposed to left ventricular apex within the intrapericardial space. Right Upper; HVAD components. Right Lower; Unidirectional centrifugal flow through the HVAD (Image courtesy of Heartware (Framingham, MA))
HeartMate II | HVAD | |
---|---|---|
Manufacturer | Thoratec | Heartware |
FDA approval | Bridge to transplant (2008) | Bridge to transplant (2012) |
Destination therapy (2010) | ||
Clinical trials | HeartMate II BTT trial [2] | ADVANCE BTT trial [4] |
HeartMate II DT trial [3] | ||
Size | 342 g | 160 g |
Speed range | 6000–15,000 rpm | 1800–4000 rpm |
Mechanism of flow | Axial | Centrifugal |
Flow estimation | Less reliable | More reliable |
The HeartMate II device has become the predominant CF-LVAD for patients with medically refractory heart failure [5, 6]. In 2008, it was approved by the US Food and Drug Administration (FDA) as a bridge to transplant strategy after pivotal clinical trial data demonstrated 6–12 month survival rates of 75 % and –68 %, respectively, in patients awaiting cardiac transplantation [2]. In 2010, it received FDA approval for destination therapy after the HeartMate II DT trial [3] demonstrated a 2 year survival rate of 58 % in patients with CF-LVADs, and superior outcomes with respect to disabling stroke, reoperation or device replacement as compared to the first generation pulsatile LVADs.
The Heartware HVAD received its FDA approval as a bridge to transplant strategy in 2012 following results of the ADVANCE trial [4]. In comparison to INTERMACS controls, consisting predominantly of HeartMate II LVADs, Heartware HVAD outcomes were non-inferior for the primary endpoint of 180 day survival, transplantation or device explantation for myocardial recovery. In addition, patients with Heartware HVADs had improved functional capacity and overall quality of life [4].
The continuously improving outcomes benefits afforded by LVADs have been somewhat offset by device-related complications. Reports from the INTERMACS registry indicate significant adverse event rates related to bleeding, infection and cardiac arrhythmias [1] that often lead to increased hospitalization and patient morbidity [7]. With pressures to improve patient survival, enhance quality of life and limit hospital readmission rates, optimizing LVAD outpatient medical management has become both a necessity and priority. This chapter will outline current approaches to the care of the ambulatory patient with continuous flow ventricular assist devices.
Goals of Outpatient Management
Prior to discharge, patients undergo extensive preparation for life outside of the hospital with a ventricular assist device [8]. A multidisciplinary team is involved to ensure patient safety and education. This medical team includes cardiac surgeons, heart failure cardiologists, nurse practitioners, physician assistants, physical and occupational therapists, nutritionists and pharmacists. Specific learning objectives include recognition of device alarms, percutaneous lead and abdominal wound care, and safe device operation including power cable and battery exchanges. Additional teaching will inform the patient of potential signs and symptoms of VAD-related complications, and reinforce compliance with medications and healthy heart lifestyles standard to their pre-device heart failure management. Only following an education program, and often requiring successful completion of an examination, are patients deemed safe for hospital discharge either to a home environment or rehabilitation center.
Ambulatory Clinical Assessment and Management
Regular outpatient follow-up ensures the longitudinal assessment of patient status, evaluation of appropriate device function, screening for VAD-related complications and optimization of medical therapy. Additional studies including the 6-min walk test, cardiopulmonary exercise testing, echocardiography and right-heart catheterization may provide further indices of functional capacity and overall myocardial function. Accordingly, the clinical assessment may be divided into a thorough examination of patient and device.
LVAD Focused Patient History
A clinical history will attempt to reveal signs and symptoms of volume overload, including fatigue, dyspnea, orthopnea, paroxysmal nocturnal dyspnea, pedal edema and worsening abdominal girth. Potential LVAD-related complications may be elicited from a history of fever, jaundice, discolored urine, transient neurological deficits, bleeding, defibrillator discharges or audible LVAD alarms. Additional history may focus on a review of medications and patient compliance, dietary discretion, quality of life and caregiver fatigue.
Physical Examination
Physical examination begins with a measurement of baseline and orthostatic vitals. A palpable pulse may not reliably be present in the CF-LVAD patient depending on the degree of mechanical unloading, aortic valve opening and intrinsic myocardial contractility. As such, heart rate and rhythm are typically derived from a traditional electrocardiogram. Measurement of an auscultatory blood pressure using a sphygmomanometer may also prove difficult in the absence of pulsatility. In such patients, a Doppler probe may be used to signal a blood pressure reading. Once the brachial artery is localized using the Doppler, the blood pressure cuff is inflated until the signal is no longer audible. The blood pressure is recorded at which point the signal returns with cuff deflation. However, care must be taken in LVAD patients with a pulse as the Doppler recording may in fact represent a systolic blood pressure rather than the assumed mean [9].
Measurement of jugular venous distension, the presence of c-v waves or a Kussmaul’s sign may be indicative of elevated right-sided filling pressures due to right ventricular dysfunction or frank volume overload.
Precordial auscultation should reveal a continuous LVAD hum with superimposed first (S1) and second (S2) heart sounds, the latter predominantly arising from pulmonic valve closure with potential contribution from the aortic valve depending upon the frequency and degree of its opening. Low frequency heart sounds including an S3 or S4 may prove challenging to auscultate over the LVAD hum. Systemic perfusion may be derived from the relative warmth of the peripheral extremities.
A respiratory exam including measurement of ambient oxygen saturation and auscultation of the chest for potential adventitious sounds may be followed with an examination of the abdomen for ascites, hepatomegaly or jaundice suggestive of liver congestion or hemolysis. A rectal exam may be necessary if a clinical history elicits concern for melena or frank blood per rectum. The key component of the abdominal exam is assessment of the percutaneous driveline, its position, securement and the healing status of its exit site. Discharge, purulence, or palpable tenderness along the driveline’s intra-abdominal course should heighten the suspicion for an infection.
Neurological examination may yield potential deficits arising from a cerebral bleed or embolic event to which the LVAD patient is vulnerable. Assessment of body mass index (BMI) may serve as a marker of nutritional status and cardiac transplant eligibility.
LVAD Interrogation: Thoratec HeartMate II
LVAD parameters can be assessed once the patient connects their system controller to a power base unit (PBU). The initial PBU display provides a table of parameter readings logged in chronological order (See Fig. 28.3). Device alarms and temporal trends of LVAD flow, pulsatility index (PI) and pump power may provide important clues to device function and patient clinical status.


Fig. 28.3
HeartMate II Monitor Display Screens. Upper left panel; Power base unit (PBU) display demonstrating normal values for pump speed, flow, power and pulsatility index (PI). Upper right panel; PBU display detailing device speeds, power and pulsatility index over time. The highlighted PI event is notable for a speed drop from 9600 rpm to the low speed limit set at 9000 rpm. Lower left panel; Low speed alarm triggered if the device operates at a speed at least 200 rpm slower than the low speed limit. In this instance, the LVAD speed is 8600 rpm despite a low speed limit of 9000 rpm. Lower right panel; Red heart alarm display resulting from a pump stoppage, low flow (< 2.5 L/min) or disconnection of the percutaneous lead (Modified and courtesy of Thoratec (Pleasanton, CA))
Power, the product of the voltage and current applied to the motor, is directly measured by the LVAD. Normal power for the HeartMate II LVAD may range from 5 to 7 Watts. Higher power readings may reflect greater demand from faster device speeds. However, sustained power spikes of greater than 10–12 Watts at baseline speed may suggest intermittent resistance to flow from a developing device thrombosis.
Flow is an estimated value derived from device speed and consumed power. As such, higher flow values may be confounded by conditions (i.e. device thrombosis) that result in increasing power requirements but not greater pump flow.
The PI displayed on the power module represents the average magnitude of each flow pulse over a 15 s interval of time. A low PI (<3.0) may arise from conditions related to poor left ventricular preload, including excessive unloading from accelerated device speeds, hypovolemia, functional mitral stenosis, severe pulmonary hypertension, right ventricular failure or cardiac tamponade. In contrast, higher PI values may reflect enhanced contractility indicative of myocardial recovery or alternatively, high left ventricular preload states resulting from volume overload or inadequate mechanical unloading due to slower device speeds.
A “PI event” refers to a sudden change in pump flow pulsatility of at least 45 % of the PI averaged over the preceding 15 s interval. PI events may be attributed to significant orthostasis exaggerated by hypovolemia or vasodilation, or arrhythmias leading to poor ventricular preload. They may also arise from “suction events” due to transient septal contact with the inflow cannula, or from acute proximal device occlusion. In response to a PI event and assuming a suction event, the HeartMate II will automatically slow down to a preset lower speed to improve preload conditions (See Fig. 28.4). Initial clinical management of a PI event entails optimization of ventricular preload conditions, clinical reassessment with slower device speeds and echocardiography to assess ventricular chamber size.


Fig. 28.4
Heartware HVAD monitor display screens. Upper; Normal HVAD Power Base Unit (PBU) display demonstrating fixed pump speed, estimated flow and device power. Normal flow waveforms with a minimum trough flow and its difference from peak greater than 2 L/min (*). Lower; HVAD PBU display screen demonstrating a red heart alarm due to a critical battery issue. The troubleshooting tab provides a differential diagnosis underscoring each of the three potential red heart alarm triggers (critical battery, high watts and power disconnect) (Image modified and courtesy of Heartware (Framingham, MA))
Red heart alarms indicate critically low pump flow and/or pump stoppage either representing mechanical failure of the pump itself or, more commonly, malfunction or disconnection of the percutaneous driveline and/or controller circuits. Such events should prompt a thorough evaluation of the device with inputs from the manufacturer and the LVAD care team including cardiac surgeons and heart failure specialists.
LVAD Interrogation: Heartware HVAD
The Heartware HVAD monitor also displays pump speed and power. Flow is reported with very good precision using software that takes the patient’s current hematocrit into account. In addition, flow is presented as a pulsatility waveform. With the HVAD, pulsatility is measured as the difference between the peak and trough of the flow waveform. Optimal pump flow requires a waveform trough and calculated pulsatility to each be greater than 2 l per minute. The relationship between device speed, the magnitude and pattern of waveform pulsatility, and LVAD flow is depicted in Fig. 28.5. Highlighting the dynamic relationship between patient clinical status and device function. As demonstrated, faster device speeds result in higher LVAD flow and therefore, greater mechanical unloading. However, excessive LVAD speed may unduly unload the ventricle. This may lead to reduced pulsatility and smaller ventricular chamber sizes that are vulnerable to suction events and possible device occlusion with complete cessation of flow. As such, an understanding of HVAD waveform analysis can facilitate the optimization of device-based patient care. In addition, the HVAD monitor may display critical alarms and provide a differential diagnosis of their potential etiologies.


Fig. 28.5
Relationship between Heartware HVAD flow rate, device speed and pulsatility. Faster HVAD speeds result in higher LVAD flow rates and lower pulsatility from greater mechanical unloading. Partial and full suction events, characterized by both waveform inversion and their magnitude of deflection, can arise if speeds are increased beyond permissive ventricular loading conditions and may eventually lead to cessation of pump flow from device occlusion (Image courtesy of Heartware (Framingham, MA))
Investigations
Routine laboratory tests measured during an LVAD clinic visit typically consist of a measured hematocrit, electrolytes, creatinine and blood urea nitrogen (BUN). Anticoagulation targets may be adjusted according to device and patient-specific international normalized ratio (INR) goals. Serum lactate dehydrogenase (LDH) may be used as an important marker of hemolysis. Clinical suspicion for device thrombosis should also prompt testing for plasma free and urine hemogloblin, haptoglobin levels and reticulocyte counts [10]. Abnormal cardiac biomarker measures, such as brain natriuretic peptide (BNP) or troponin, may suggest myocardial strain from elevated filling pressures, inadequate mechanical unloading or infarction.
Chest and abdominal x-rays provide important clues related to LVAD inflow cannula position, outflow graft bend relief status and the integrity of system controller wires.
A transthoracic echocardiogram should be undertaken at routine intervals during follow-up or with any change in clinical status [11]. A baseline echocardiogram offers invaluable information related to cardiac structure and hemodynamics following LVAD implantation. Prior to discharge, a ramp echocardiogram is often performed to assess the change in left and right ventricular dimensions and severity of valvular regurgitation across a range of device speeds. Although a validated approach with prognostic evidence is lacking, there is general consensus that the optimal device speed refers to that which ensures the adequate decompression of the left ventricle while maintaining the interventricular septum in mid-position, minimizing mitral regurgitation and preserving right ventricular size and function. During subsequent clinical follow-up, echocardiographic comparisons of these indices or the presence of a device-related complication (e.g. thrombus, aortic insufficiency) may account for changes in clinical status, and suggest the need for further tailoring of medical therapy or adjustment of LVAD parameters.
Medical Therapy
Heart Failure
Volume overload may arise in the LVAD patient due to inadequate diuresis, medical or dietary non-compliance, right ventricular failure or ineffective mechanical unloading. Therapy entails counseling and optimization of diuretics and device parameters. There is general consensus that chronic heart failure management, including beta-blockers, angiotensin converting enzyme inhibitors or angiotensin blockers, and aldosterone antagonists be re-initiated following LVAD implantation [11]. Whether such therapies alter the natural history of the right or mechanically supported left ventricle in all LVAD patients remains to be clarified. However, there is evidence that amongst select individuals, namely younger patients with non-ischemic cardiomyopathies of short (< 3 months) duration, maximally tolerated neurohormonal blockade with adjunctive mechanical support may facilitate myocardial recovery [12]. Previous studies demonstrated recovery rates ranging from 1 to 13 % amongst LVAD patients supported with variable device types and medical therapies [13–15].
The Harefield protocol [12] was the first to mandate a two phase strategy in which patients with dilated non-ischemic cardiomyopathies follow a strict neurohormonal regimen of lisinopril 40 mg daily, carvediolol 25 mg three times daily, spironolactone 25 mg daily, digoxin 125 μg daily and losartan 100 mg daily to promote reverse ventricular remodeling following placement of a HeartMate II LVAD. Patients were maintained on this protocol until which time their left ventricular end diastolic dimension was less than 60 mm when measured at the lowest LVAD speed of 6000 rpm for 15 min. Subsequently, in the second phase of the protocol, patients were given clenbuterol, a sympathomimetic amine with β2 agonist properties, with the substitution of the non-selective beta-blocker, carvedilol, with the β1 antagonist, bisoprolol. It has been proposed that the addition of clenbuterol promotes physiologic myocardial hypertrophy. With this strategy, 63 % of patients underwent LVAD explantation after an average of 286 days of mechanical support with normalization of filling pressures, ejection fraction and an 83 % survival without recurrent heart failure at 1 and 3 years following device removal [16].
More recently, the Montefiore three-step cardiac recovery protocol, involving echocardiography, cardiopulmonary stress testing and right heart catheterization, demonstrated normalization of cardiac function in 24 % of LVAD patients with a device explantation rate of 14 % [17, 18]. Myocardial recovery did not require clenbuterol and was achieved using the combination of neurohormonal blockade and continuous flow mechanical support.
Blood Pressure Management
Current consensus recommends a mean blood pressure of less than 80 mmHg for patients with CF-LVADs [11]. Heart failure medications, such as beta blockers and renin-angiotensin-aldosterone system antagonists are the preferred first line agents to achieve a normotensive LVAD state [11]. Higher systemic pressures have anecdotally been associated with an increased propensity for intracranial bleeding. Pressure-dependent long term outcomes, particularly in DT LVAD patients, merit further investigation.
Antithrombotic Therapy
CF-LVADs require both anticoagulation and antiplatelet therapy to reduce the risk of device thrombosis and other thromboembolic events. Coumadin is initiated in the post-operative state once hemostasis has been achieved. INR targets vary according to LVAD device (2.0 ± 0.5 for HM II and 2.0–3.0 for Heartware) and their relative thrombotic risk [10]. This range bears clinical significance as an INR < 1.5 has been associated with an increased risk of thromboembolism in HeartMate II patients, whereas hemorrhagic event rates were reportedly higher with an INR > 2.5 [19]. In practice, however, this may vary according to institutional experience and individual patient history with respect to bleeding and thrombosis.
All LVAD patients should receive daily aspirin (81–325 mg). Some institutions may also add dipyridamole 75 mg three times daily to their antiplatelet regimen. Heartware HVAD patients should be assessed for aspirin resistance using Verifynow, a P2Y12 resistance assay. For aspirin non-responders, combination therapy with clopidogrel or dipyridamole may be necessary.
Adjuvant Medical Therapy
Particular patients may require specific medical therapies given their VAD-related complications, including anti-arrhythmic agents for ventricular arrhythmias, chronic suppressive antibiotic therapy for drive-line infections and pulmonary vasodilators for right ventricular dysfunction.
LVAD-Related Complications
Despite improved survival outcomes with device therapy, LVAD complications remain a significant source of patient morbidity and healthcare burden [20]. The following section will elaborate upon common complications related to CF-LVADs and their current management strategies.
The Hemostatic Conundrum: Bleeding and Thrombosis
Bleeding
In both clinical trials and registry data, bleeding constitutes the greatest complication burden to patients with CF-LVADs. Patients are at significant risk for bleeds ranging from epistaxis and gastrointestinal (GI) bleeding to fatal intracerebral hemorrhage. The incidence of hemorrhagic strokes in the HeartMate II BTT, DT and Heartware ADVANCE trials has ranged from 2 to 11 % with annual event per patient rates as high [2–4].
However, the vast majority of bleeding complications with CF-LVADs arise from GI bleeding. Some centers report an incidence of 19–23 % in their HeartMate II patient population with independent predictors consisting of thrombocytopenia, an elevated INR, hypertension and a prior history of GI bleeding source [21–23]. Thrombotic and bleeding complications amongst HeartMate II patients have respectively been reported to account for 9 and 29 % of hospital readmissions [7].
CF-LVAD patients predisposed to the development of arteriovenous malformations [23, 24] in a manner similar to Heyde’s syndrome in which high shear stress across a stenotic aortic valve is associated with angiodysplasia within the GI tract, although it is uncertain whether new AVMs develop or existing AVMs bleed because of anticoagulation and the bleeding diathesis induced by CF-LVADs [25]. Specifically, continuous flow through the LVAD pump may lead to an acquired Type IIa von Willebrand syndrome through the loss of high molecular weight multimers of von Willebrand factor (vwf) [26–28]. Occurring as early as within 1 day following CF-LVAD implantation [27], the syndrome may lead to an age-dependent increase in mucosal bleeding [28]. Reversibility of the syndrome was evidenced by vwf restoration following device removal or cardiac transplantation [28, 29]. Yet, bleeding does not occur in all CF-LVAD patients despite ubiquitous vwf deficiency and further study is needed to prospectively identify those patients who may be free of thrombosis with minimal or no anticoagulation.
Management of a bleed typically begins with discontinuation of anticoagulation and antiplatelet therapies, blood transfusions when deemed necessary, initiation of proton-pump inhibitors and consultation with gastroenterology specialists. Investigations include fecal occult blood tests to confirm melena and imaging modalities such as upper GI endoscopy, colonoscopy, push enteroscopy, mesenteric angiography, tagged red blood cell scans or pill capsule endoscopy to localize and potentially treat a bleeding source [23, 30, 31]. However, non-focal GI bleeding may occur in a subset of patients despite diagnostic efforts creating a management dilemma. Studies have begun to explore novel therapies including the somatostatin analogue, octreotide; Factor VIII/von Willebrand factor replacement therapy using Humate P; and even adrenaline as a means of increasing pulsatility to address the presumed culprit of continuous flow [21, 32, 33].
Device Thrombosis
Thrombus within the pump or the outflow graft increases the risk for systemic embolization and may lead to inadequate ventricular unloading or pump failure (See Fig. 28.6). The incidence is not trivial with an initially reported rate of occurrence of 2 [2] and 4 % [3] in the HeartMate BTT and DT trials. It is noteworthy that criteria for device thrombosis were quite rigid in the clinical trials. We believe that clinically relevant device thrombosis may occur in up to 10 % of patients and recent preliminary reports from the ADVANCE-CAP study [34] and the FDA report for Heartware HVAD approval support this contention.


Fig. 28.6
VAD-Related Thrombosis. Clot within the outflow graft (left; black arrow). In situ device thrombus (right; red arrow) (Images courtesy of Dr. Hiroo Takayama, Division of Cardiothoracic Surgery, Columbia University Medical Center)
Pump thrombosis may arise from multiple factors including inadequate anticoagulation, malposition of the inflow cannula, intrinsic hypercoagulability, ingestion of residual clot from the cardiac chambers or from imperfections of the impeller. Frequency of aortic valve opening and device flow have been proposed as plausible risk factors for pump thrombosis [35]. Closure of the aortic valve with faster speeds ensures that blood flows in series from the ventricle into the pump ensuring maximal flow through the device. On the other hand, aortic root washout may be suboptimal in this setting. In contrast, LVAD flow may be diminished when blood flows in parallel via both the pump and the left ventricular outflow tract when the aortic valve is permitted to open although this situation permits optimal root washout. Ultimately, such hypotheses will have to be prospectively investigated.
Device thrombosis may initially present with or without of signs and symptoms of worsening heart failure due to inadequate mechanical unloading. Clinical markers of hemolysis including jaundice or icterus, and laboratory measures such as an elevated plasma free hemoglobin, low haptoglobin or the presence of hemoglobinuria are suggestive of red blood cell lysis due to obstruction-related non-laminar flow through the device. Moreover, a serum level of lactate dehydrogenase (LDH) greater than three times normal has been found to have a specificity of 90 and a 100 % sensitivity for a device thrombosis [36]. Interrogation of the system controller may further reveal episodes of sustained power spikes. Echocardiographic findings of an unloaded ventricle including increased myocardial contractility, ventricular dilatation, changes in Doppler flows or worsening mitral regurgitation may also suggest impairment of LVAD function [37]. In the presence of a high pre-test likelihood of device thrombosis, e.g. LDH greater than three times normal, the Columbia Ramp protocol can be used to quantify the relative change in left ventricular dimension with changing LVAD speeds (See Fig. 28.7). The slope derived from the linearization of this relationship may be used to noninvasively identify device thrombosis [36]. In this study, each patient with a positive ramp study and evidence of end-organ dysfunction, e.g. renal failure, proceeded to device exchange with subsequent intra-operative confirmation of pump thrombosis.


Fig. 28.7
Diagnostic algorithm for device thrombosis using the Columbia Ramp Protocol (Modified from Uriel et al. [36])
Management of Device Thrombosis
Initial management of an in situ pump thrombosis entails aggressive anticoagulation typically with intravenous heparin. The use of GPIIa/IIIb inhibitors [38] and intraventricular thrombolytics [39] have demonstrated modest success but have frequently been limited by potentially fatal hemorrhagic complications. Increasing LVAD speed may also be considered as a strategy to overcome a hemodynamically obstructive pump thrombosis. However, this carries the theoretical risk of systemic LVAD clot embolization. Ultimately, symptom severity and signs of end-organ damage indicative of poor systemic perfusion often requires reoperation for device exchange.
A subset of patients with HeartMate II LVADs implanted between February 2010 and April 2012 may be at risk for a bend relief disconnect [40, 41]. The bend relief refers to the polytetrafluoroethylene tubing that sheathes the proximal outflow graft at its insertion point with the pump. Detachment renders the underlying outflow graft susceptible to malformation, damage and potential obstruction from kinking or in situ thrombus formation. Patients may present with features of hemolysis and symptoms of heart failure due to outflow impairment. The incidence of full and partial disconnection has been reported to be as high as 11 and 23 % at Columbia University Medical Center for LVADs implanted during this period. Anterior-posterior abdominal x-rays facilitate the diagnosis and treatment may require surgical correction (See Fig. 28.8). However, design modifications following a US FDA Class 1R recall in April 2012 should obviate this complication for HeartMate II LVADs produced after this date.


Fig. 28.8
HeartMate II bend relief disconnect. Normal bend relief position (white arrow) at its insertion point between the outflow graft (black arrowhead) and the LVAD pump (black arrow) as demonstrated by an abdominal x-ray (a) and photo (b). Inflow cannula marked by white arrowhead. Abdominal x-ray (c) and photo (d) of a full bend relief disconnection [40]
Aortic Insufficiency
The de novo development of native valve aortic insufficiency (AI) has gained greater recognition as an acquired complication with long-term device use. Mild to moderate AI has been reported by several groups to have a prevalence of 25 % after 1 year of support [42–44]. In the presence of AI, blood initially pumped by the LVAD into the ascending aorta regurgitates back into the left ventricle rendering the device inefficient. Multiple risk factors have been associated with AI development including patient age, duration of LVAD support, as well as aortic root diameter. In addition, failure of the aortic valve to open during LVAD support has been associated with AI and its progression [43–45]. Leaflet stasis arising from aortic valve closure can predispose to commissural fusion and consequent malcoaptation leading to valvular incompetence [46]. However, consensus is lacking in regards to the ideal LVAD setting to ensure appropriate mechanical unloading and frequency of aortic valve opening as lower speeds required to accomplish this may be associated with a higher frequency of device thrombosis.
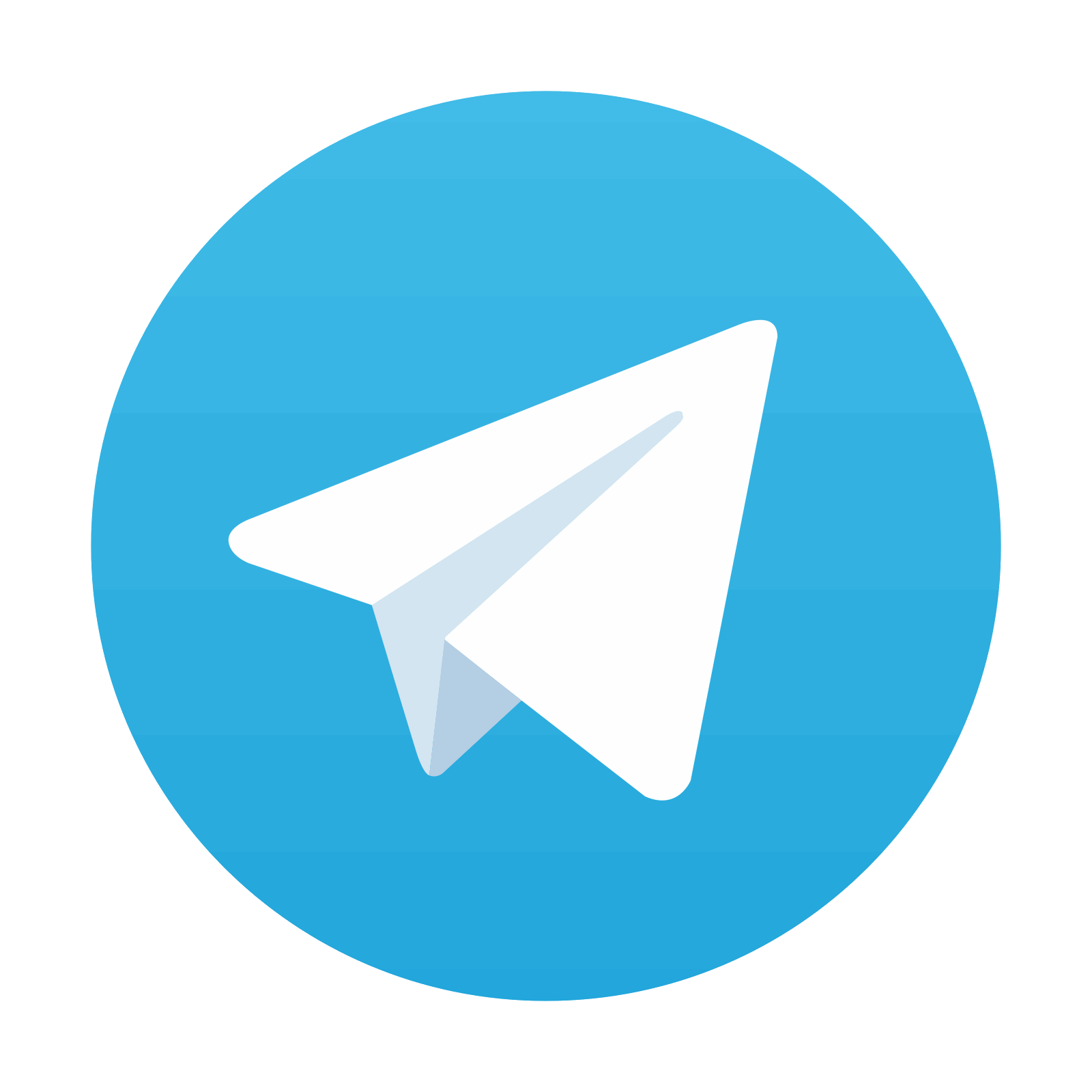
Stay updated, free articles. Join our Telegram channel

Full access? Get Clinical Tree
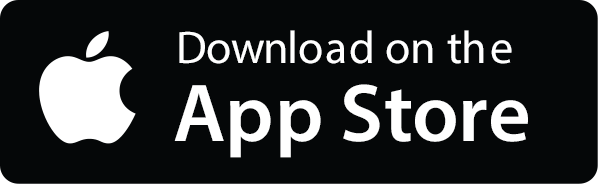
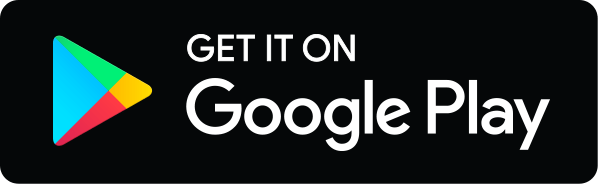