Fig. 29.1
Computed tomographic pulmonary angiogram demonstrating bilateral filling defects in the branches of the pulmonary arteries (white arrows), which are diagnostic of acute pulmonary embolism. (a) A “four-chamber view” in the transverse plane, which allows a first assessment of right ventricular size, more specifically of the right-to-left (R/L) ventricular diameter ratio at the tricuspid/mitral annulus level (b)
Clinical Severity and Risk of Early Death Determine Initial Management
Current international guidelines emphasize that the appropriate management of patients with confirmed acute PE requires their stratification into classes of disease severity in order to create an algorithm which adjusts the modalities of medical, surgical, or interventional treatment to the early death or complication risk (Fig. 29.2) [6]. Patients with clinically overt right ventricular failure on admission, which results in reduced cardiac output and manifests as persistent hypotension accompanied by signs of end-organ hypoperfusion (i.e., frank cardiogenic shock), are classified into the high-risk (or “massive”) PE category. These patients undoubtedly constitute the most challenging subgroup, exhibiting 30-day fatality rates of 20–40% or even higher, and they are in need of immediate treatment of acute right heart failure in addition to medical or pharmacomechanical reperfusion.


Fig. 29.2
Integrated risk-adjusted management algorithm for acute pulmonary embolism (adapted from [6] A/C = anticoagulation, CT = computed tomographic, PE = pulmonary embolism, PESI = Pulmonary Embolism Severity Index, RV = right ventricular, sPESI = simplified Pulmonary Embolism Severity Index)
The high-risk group represents only 5% or even less of all PE patients [1, 7]. Outside this emergency situation, normotensive, “not-high-risk PE” patients should further be stratified into intermediate versus low risk using two categories of tools or modalities: (1) the Pulmonary Embolism Severity Index (PESI) , or its simplified form (sPESI) , reflecting clinical severity and comorbidity, and (2) imaging and/or laboratory tests detecting subclinical right ventricular dysfunction or myocardial injury [6]. While PESI and sPESI primarily serve to identify low-risk patients who may be eligible for early discharge and home treatment, echocardiographic (or computed tomographic) or biochemical markers of right ventricular (RV) dysfunction represent the key tool for defining the groups of “intermediate-low risk” (with either evidence of RV dysfunction or elevated biochemical markers) or “intermediate-high risk” (with RV dysfunction combined with elevated biochemical markers) (Table 29.1). This advanced classification on the basis of the functional status of the right ventricle helps to determine the need for and duration of hemodynamic monitoring as well as the need for (rescue) reperfusion treatment (Fig. 29.2). It may also be helpful for the choice of the initial anticoagulant regimen, as will be explained below.
Table 29.1
Risk categories in patients with acute pulmonary embolism
Early mortality risk | Risk parameters and scores | ||||
---|---|---|---|---|---|
Shock or hypotension | PESI class III–V or sPESI ≥1 | Signs of RV dysfunction on an imaging test | Cardiac laboratory biomarkers | ||
High | + | (+) | + | (+) | |
Intermediate | Intermediate-high | − | (+)a | Both positive | |
Intermediate-low | − | (+)a | Either one (or none) positive | ||
Low | − | − | Assessment optional; if assessed, both negative |
Management of Acute Right Heart Failure
The principles of acute right heart failure management were recently reviewed in a statement from the Heart Failure Association and the Working Group on Pulmonary Circulation and Right Ventricular Function of the European Society of Cardiology [9]; an overview of the current treatment options for acute RV failure is provided and briefly discussed in Table 29.2.
Table 29.2
Medical and device treatment of overt right heart failure in patients with acute high-risk pulmonary embolism
Strategy, drug, or device | Dosage | Properties and instructions | Caveats |
---|---|---|---|
Volume optimization | |||
Volume loading with saline or ringer lactate | At least 200 mL over 15–30 min | Consider patients with decompensated RV failure, normal central venous pressure, and low arterial pressure | Volume overloading may further distend the ventricles, worsen ventricular interdependence, and reduce cardiac output |
Vasopressors and inotropes | |||
Norepinephrine | 0.2–1.0 μg/kg/min | Increases RV inotropy, systemic blood pressure, promotes positive ventricular interactions, restores coronary perfusion gradient | Excessive vasoconstriction may further reduce tissue perfusion |
Dobutamine | 2–20 μg/kg/min | Increases RV inotropy, lowers filling pressures | May aggravate arterial hypotension if used alone, without a vasopressor, especially if left heart failure coexists |
Levosimendan | 0.1–0.2 μg/kg/min (6–12 μg/kg bolus over 10 min optional and not recommended if SBP <90 mmHg) | Combines RV inotropy and pulmonary vasodilation; favorably effects right ventricular-arterial uncoupling | May aggravate arterial hypotension |
Infusion can be decreased to 0.05 or increased to 0.2 μg/kg/min | |||
Mechanical circulatory support | |||
ECMO/ECLS | – | Short-term support, cost-effective, rapid; oxygenator can be added | Complications with use over longer periods (>5–10 days) |
Percutaneous catheter-mounted micro-axial pumps | – | – | Limited pump capacity; ECLS preferred in severe cardiogenic shock or if high pump flow required |
Paracorporeal RVAD | – | Appropriate for longer-term use (e.g., weeks or months); can be combined with oxygenators when pulmonary support also needed | – |
Acute RV failure principally responds to changes in preload. Importantly, however, excessive volume loading may increase wall tension, decrease contractility, impair left ventricular filling, and ultimately further reduce systemic cardiac output and tissue perfusion. Cautious volume loading guided by central venous pressure monitoring and aimed at reaching and maintaining pressures of 5–10 mmHg is the most reasonable approach.
Vasopressors, particularly noradrenaline, are preferred in shock since they restore blood pressure and improve cerebral, coronary, and other organ perfusion, without disproportionately increasing pulmonary vascular resistance. By combining RV inotropy and pulmonary vasodilation, levosimendan appears to have a favorable hemodynamic profile, even though the evidence to support its use in precapillary RV failure (i.e., not due to left heart disease) is not very strong yet. Phosphodiesterase III inhibitors are also expected to exert positive inotropic effects on the RV without increasing pulmonary vascular resistance; however, like dobutamine, they may aggravate arterial hypotension and should therefore be combined with noradrenaline if used. Finally, mechanical circulatory support of the RV, including extracorporeal membrane oxygenation (ECMO) or life support (ECLS), may be required in acute high-risk PE. Timely implantation is critical to avoid irreversible organ damage, and thus early transfer of the patient to an expert referral center is essential.
Thrombolysis and Pharmacomechanical (Interventional) Reperfusion Therapy
Systemic Intravenous Thrombolysis
Thrombolytic agents have been used for the treatment of acute PE since the late 1960s [10]. Today, immediate systemic reperfusion treatment with intravenous thrombolysis continues to be the mainstay of therapy for high-risk (or massive) PE [6, 11, 12]. This recommendation is partly supported by meta-analyses of randomized trials which enrolled, in total, more than 2000 patients with acute PE and suggested that thrombolysis may reduce, by approximately two thirds, early mortality or hemodynamic decompensation requiring further “rescue” treatment (odds ratio [OR] 0.34, 95% CI: 0.22–0.52) [13]. Of course, this benefit is to be viewed against the risk of major bleeding which occurs much more frequently than under anticoagulation alone (OR 2.91, 95% CI: 1.95–4.36), particularly when fatal or intracranial hemorrhage is considered (OR 3.18, 95% CI: 1.25–8.11) [13]. In light of this delicate balance, current evidence-based guidelines point out that intermediate- or low-risk patients with acute PE are not likely to benefit from the routine use of systemic thrombolysis and that this treatment should therefore only be used as rescue treatment in case of hemodynamic decompensation under anticoagulation alone [6, 12]. The basis for this recommendation has been provided by the results of the Pulmonary Embolism Thrombolysis (PEITHO) trial, which compared a single bolus of tenecteplase (plus heparin) with placebo (plus heparin) in 1006 patients with acute PE and RV dysfunction plus myocardial injury detected by imaging and a positive cardiac troponin test; in this intermediate-high-risk group (Table 29.1), the clinical benefits of thrombolysis were counterbalanced by the intracranial and other major bleeding risks [14].
Emerging approaches to reperfusion treatment of PE might help to achieve comparable efficacy while minimizing the bleeding risk associated with systemic (intravenous) full-dose thrombolysis. Preliminary evidence from small studies suggests that reduced-dose systemic thrombolysis might represent an option for improving safety while maintaining efficacy of this treatment. In a prematurely terminated trial of 118 patients, half-dose recombinant tissue-type plasminogen activator (alteplase) was comparable to the full dose in terms of efficacy and possibly associated with improved safety [15]; in another study of 121 patients with (rather arbitrarily defined) “moderate PE,” reduced-dose rtPA appeared to be safe and effective over a follow-up period of more than 2 years (Table 29.3) [16]. However, until the hypothesis generated by these data is confirmed by larger, appropriately designed trials with standardized selection criteria and outcomes, the use of “low-dose” thrombolytic regimens cannot, at the moment, be proposed as an alternative to the dosage approved for systemic intravenous use.
Table 29.3
Functional and hemodynamic outcomes a in patients receiving thrombolysis for acute pulmonary embolism: overview of randomized trials and prospective cohort studies
Author and reference | Study populationa | Groupsa | Outcomeb | Follow-up | Thrombolysis group | Control group | Comparison between groups |
---|---|---|---|---|---|---|---|
Kline et al. [17] | Intermediate-risk or submassive PE (n = 83) | Tenecteplase vs placebo | NYHA class III–IV | 3 months | 5.4% | 20.5% | p = n.s |
RV dilation or hypokinesis | 33.3% | 37.8% | p = n.s | ||||
6-min walking distance <330 m | 16% | 28% | p = n.s | ||||
Composite functional outcome | 85% | 63% | p = 0.017 | ||||
Becattini et al. [18] | Intermediate-risk PE (n = 58) | Tenecteplase vs placebo | ∆ RV/LV ratio | 7 days | 0.47 ± 0.07 | 0.34 ± 0.05 | p = n.s. |
Sharifi et al. [16] | “Moderate” PE (n = 121) | Half-dose tPA vs anticoagulant alone | Mean SPAP (mmHg) | 6 months | 31 ± 6 | 49 ± 8 | p <0.001 |
SPAP ≥40 mmHg | 28 months | 16% | 57% | p <0.001 | |||
Mean SPAP (mmHg) | 28 ± 7 | 43 ± 6 | p <0.001 | ||||
Death | 1.6% | 5.0% | p = n.s. | ||||
Wang et al. [15] | High- and intermediate-risk PE (n = 118) | Half-dose tPA 50 mg vs tPA 100 mg | ∆ RVED/LVED; ∆ RVWM; ∆ SPAP; lung perfusion defects and obstruction score | 24 h and 14 days | No differences between groups reported | ||
Kuo et al. [19] | Massive or submassive PE (n = 101) | All treated with CDT | SPAP | Pre- vs post-CDT | 51.2 ± 14.1 → 37.2 ± 15.8 | p <0.001 | |
RV strain (Bowker test of symmetry) | 89.1% (95%CI: 76.8%–94.4%) with vs 10.9% with no improvement | p <0.001 | |||||
Kucher et al. [20] | Intermediate- risk PE (n = 59) | CDT vs anticoagulant alone | ∆ RV/ LV ratio | 24 h | 0.30 ± 0.20 | 0.03 ± 0.16 | p <0.001 |
∆ RV/RA pressure gradient (mmHg) | 9.8 ± 9.9 | 0.3 ± 10.9 | p = 0.03 | ||||
∆ TAPSE (mm) | −3.1 ± 4.4 | 0.9 ± 4.9 | p = 0.02 | ||||
∆ RV/LV ratio | 90 days | 0.35 ± 0.22 | 0.24 ± 0.19 | p = n.s. | |||
∆ RV/RA pressure gradient (mmHg) | 12.3 ± 12.8 | 11.6 ± 15.1 | p = n.s. | ||||
∆ TAPSE (mm) | −6.1 ± 4.6 | −3.4 ± 5.4 | p = n.s. | ||||
Piazza et al. [21] | Intermediate- or high-risk PE (n = 150) | All treated with CDT, low-dose thrombolysis | Mean RV/LV ratio | Pre- vs post-CDT (48 h) | 1.55 ± 0.39 → 1.13 ± 0.2 | p <0.001 | |
Mean SPAP (mmHg) | 51.4 ± 16 → 36.9 ± 14.9 | p <0.001 | |||||
Modified Miller index | 22.5 ± 5.7 → 15.8 ± 5.9 | p <0.001 |
Pharmacomechanical, Catheter-Directed Reperfusion
Catheter-directed pharmacomechanical reperfusion with low-dose local thrombolysis has been developed as an option for clearing pulmonary emboli from larger arteries [22]; this procedure is an alternative to operative embolectomy if systemic thrombolysis is contraindicated or the bleeding risk is high [8]. A phase 2 randomized multicenter trial enrolled patients with acute PE and a right-to-left ventricular dimension ratio >1.0, comparing unfractionated heparin plus a 15-h catheter-directed, ultrasound-assisted regimen of 10–20 mg rtPA versus heparin alone [20]. Catheter-directed treatment led to significant recovery of RV function at 24 h, with no increased risk of major hemorrhage [20]. The efficacy and safety of the pharmacomechanical approach using low-dose local thrombolysis were more recently supported by the results of a prospective, single-arm multicenter trial [21], and those of a registry, both including patients with massive or submassive PE [19] (Table 29.3).
Like any other interventional procedures, catheter-directed pharmacomechanical reperfusion requires adequate operator expertise and institutional volume. Furthermore, it remains to be determined whether the speed of thrombus removal, and consequently of the relief of the RV from pressure overload, is adequately high in patients with overt or imminent hemodynamic decompensation and whether the use of ultrasound is really necessary for obtaining maximum efficacy [20].
Impact of Thrombolysis on Late Outcomes After Pulmonary Embolism
Cohort studies with long-term follow-up suggest that a substantial proportion of patients who have survived an acute PE episode may complain of persistent functional limitation and/or reduced quality of life for long periods after the index event [2]. Moreover, some degree of persistent pulmonary hypertension or RV dysfunction was observed in as many as 40% of survivors followed over 6 months to 1 year after acute PE [23]. These data are to be interpreted with caution as the number of patients followed in observational studies performed so far was rather small, echocardiographic parameters of RV dysfunction were not standardized, and a correlation of ultrasound findings with the severity of patients’ symptoms or the degree of functional limitation could not be established [24]. Similarly, the broad range of the reported CTEPH incidence rates after symptomatic PE (0.1–9.1% of the patients within the first 2 years [3]) is probably due to referral bias, absence of early symptoms, and the occasional difficulty in differentiating truly acute PE at baseline from an acute episode superimposed on pre-existing CTEPH [25].
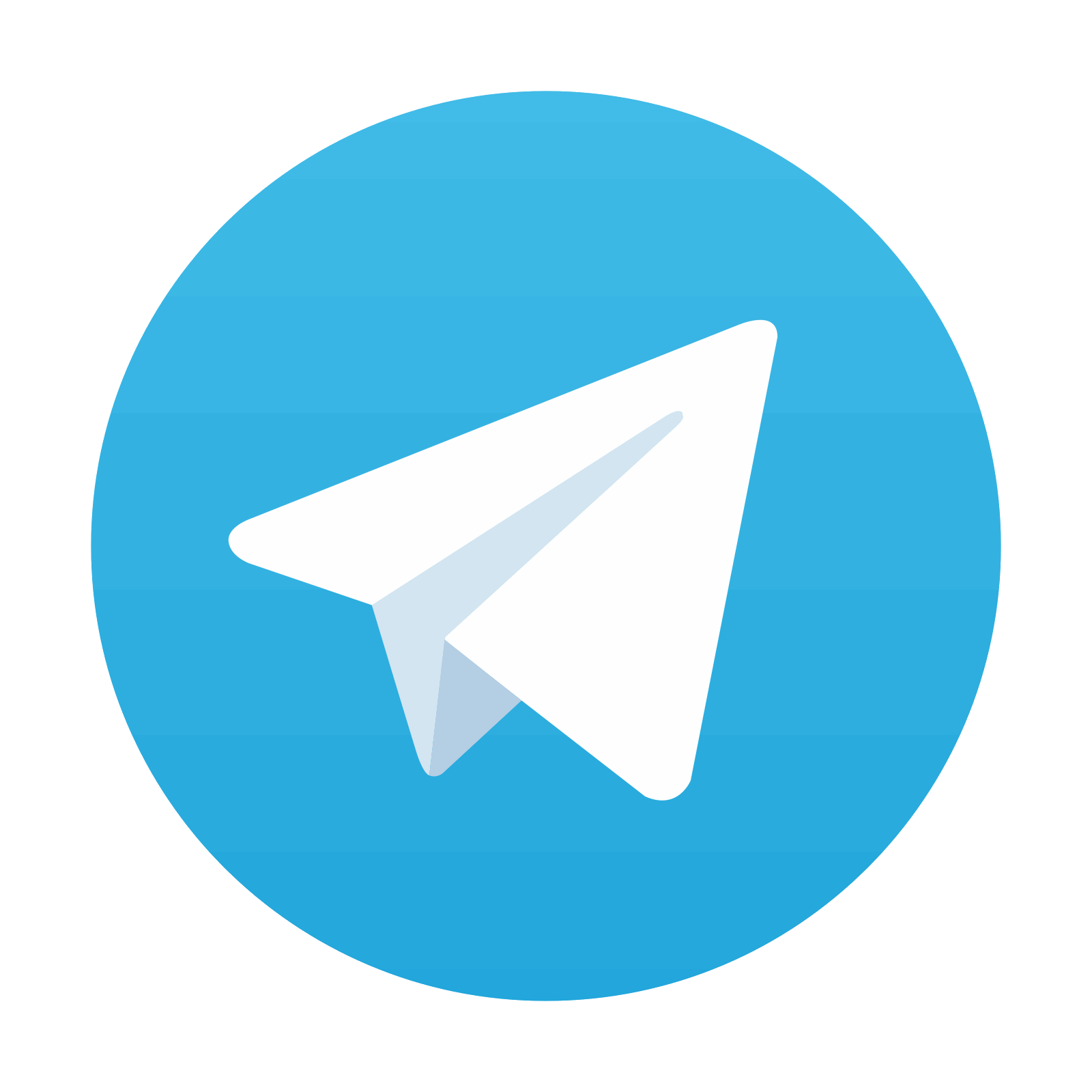
Stay updated, free articles. Join our Telegram channel

Full access? Get Clinical Tree
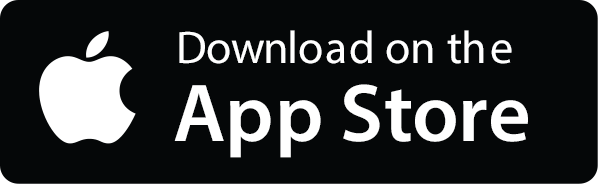
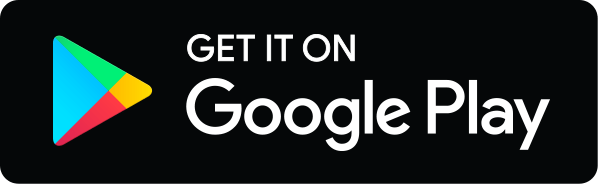