Mechanisms of Tachycardia
Introduction
The three mechanisms responsible for tachycardia are 1) reentry, 2) enhanced automaticity, and 3) triggered activity. Electrocardiographic presentations of tachycardia are mechanism specific including its initiation, termination, and response to pacing maneuvers. Understanding tachycardia mechanisms are important for developing specific mapping strategies for ablation.
The purpose of this chapter is to:
Discuss different mechanisms and clinical presentations of tachycardia.
Discuss mapping strategies for ablation based on tachycardia mechanism.
MECHANISMS
REENTRY
The mechanism responsible for the majority of pathologic tachycardias is reentry. These tachycardias include atrial fibrillation, atrial flutter, intraatrial reentrant tachycardia, atrio-ventricular nodal reentrant tachycardia (AVNRT), accessory pathway-mediated reentrant tachycardias (ortho- and antidromic reentrant tachycardia), sinus node reentrant tachycardia (SNRT), bundle branch reentrant tachycardia (BBRT), idiopathic left ventricular tachycardia (VT), and scar-related VT.
Circuit
A simplified reentrant circuit is composed of two longitudinally dissociated but complementary pathways (α and β) linked to form a functional circuit (Fig. 2-1).1 The α pathway has slow conduction and short refractoriness, while the β pathway has rapid conduction and long refractoriness. The conduction and refractory properties of each pathway must complement the other so that conduction times over one pathway exceeds the refractory period of its counterpart. The depolarizing wavefront, therefore, always encounters excitable tissue to perpetuate reentrant excitation. An exit site allows the depolarizing wavefront to leave the circuit and activate the rest of the heart. The revolution time around the circuit equals the tachycardia cycle length (TCL). Tissue refractory periods are dynamic and show restitution or cycle length dependency (hyperbolic relationship between action potential [AP] duration and preceding diastolic interval) contributing to dispersion of refractoriness (particularly at the steep portion of the restitution curve) that facilitates reentry.
Two basic models of reentry depict circus movement around either a 1) fixed anatomic obstacle or 2) functional area of block.2,3 During anatomical reentry, the circuit length is fixed and exceeds the tachycardia wavelength (λ = conduction velocity × refractory period). The difference between the circuit length and λ is the excitable gap. The excitable gap allows premature stimulation to penetrate the circuit and reset or entrain tachycardia. Antiarrhythmic drugs that prolong refractoriness (K channel blockers) increase the tachycardia λ, narrow the excitable gap, and terminate or prevent tachycardias by increasing the λ beyond the circuit length.4 Antiarrhythmic drugs that slow conduction velocity (Na channel blockers) decrease conduction velocity and promote conduction block, particularly at fast rates (use dependence) causing tachycardia termination. However, reducing the λ can also cause tachycardia to become incessant. Because the circuit length is fixed in anatomical reentry, the TCL (revolution time around the circuit) is inversely related to conduction velocity. By contrast, functional reentry is the smallest circuit (“leading circle”) around a central area (core) of refractoriness created by the convergence of multiple centripetal wavelets. The head of the excitatory wavefront is continuously biting its tail of refractoriness, and therefore, a fully excitable gap is absent. In functional reentry, the TCL is proportional to the tissue refractory period (not conduction velocity).
Variations of the reentrant model include 1) spiral wave reentry, 2) phase 2 reentry, and 3) reflection.5,6 In the spiral wave theory, wave curvature and conduction velocity are inversely related. Spiral waves rotate around a central inexcitable rotor core where wave curvature is greatest, conduction velocity is slowest, and therefore propagation fails. The more distal parts of
the spiral wave have less curvature and faster conduction. Stationary (anchored) and drifting spiral waves have been implicated in monomorphic and polymorphic VT, respectively. Spiral waves that encounter inexcitable tissue can break up and form daughter waves whose multiplicity is responsible for fibrillation. Phase 2 reentry is the presumptive mechanism underlying ventricular fibrillation (VF) in the Brugada syndrome. Compared to the endocardial AP, the normal epicardial AP shows a “spike and dome” configuration. The spike or epicardial notch (phase 1) results from the outward K current, Ito, while the dome (phase 2) results from inward ICa. Brugada syndrome results in an exaggerated spike (prominent Ito in the RV epicardium) and loss of the AP dome resulting in a transmural (epicardium-endocardium) gradient of repolarization that gives rise to the prominent J waves and ST segment elevation in ECG lead V1). Propagation of the dome (inward ICa) to nearby sites having loss of the dome causes local reentry (hence, termed phase 2 reentry) within the epicardium generating tightly coupled extrasystoles that trigger VF in the milieu of a transmural repolarization gradient. Reflection (reflected reentry) is the to and fro propagation of an impulse over a functionally inexcitable pathway and might contribute to ischemic ventricular extrasystoles.
the spiral wave have less curvature and faster conduction. Stationary (anchored) and drifting spiral waves have been implicated in monomorphic and polymorphic VT, respectively. Spiral waves that encounter inexcitable tissue can break up and form daughter waves whose multiplicity is responsible for fibrillation. Phase 2 reentry is the presumptive mechanism underlying ventricular fibrillation (VF) in the Brugada syndrome. Compared to the endocardial AP, the normal epicardial AP shows a “spike and dome” configuration. The spike or epicardial notch (phase 1) results from the outward K current, Ito, while the dome (phase 2) results from inward ICa. Brugada syndrome results in an exaggerated spike (prominent Ito in the RV epicardium) and loss of the AP dome resulting in a transmural (epicardium-endocardium) gradient of repolarization that gives rise to the prominent J waves and ST segment elevation in ECG lead V1). Propagation of the dome (inward ICa) to nearby sites having loss of the dome causes local reentry (hence, termed phase 2 reentry) within the epicardium generating tightly coupled extrasystoles that trigger VF in the milieu of a transmural repolarization gradient. Reflection (reflected reentry) is the to and fro propagation of an impulse over a functionally inexcitable pathway and might contribute to ischemic ventricular extrasystoles.
Initiation
Three criteria necessary for initiation of reentry are 1) unidirectional block, 2) slow conduction, and a 3) functional circuit (Fig. 2-1).5,6,7 Absolute and post-repolarization refractoriness are factors that can contribute to unidirectional block facilitating reentry. Relative refractoriness and anisotropy (differences in conduction velocity related to fiber orientation) are factors contributing to slow conduction. Premature impulses (hence, the rationale behind programmed extrastimulation) are effective triggers of reentry by exposing differences in refractory periods between two longitudinally dissociated pathways. Premature impulses that fall into the tachycardia window (defined as the difference in refractory periods between the α and β pathways) fail to conduct over the β pathway with its longer refractory period (unidirectional block) and conduct exclusively over the α pathway (slow conduction), thereby fulfilling two requirements for reentry. Sufficient delay over the α pathway allows the β pathway time to recover excitability and initiate the first beat of reentry (functional circuit).
Reentrant initiation shows abrupt onset with an inverse relationship between the coupling interval of the initiating complex and the first beat of tachycardia due to slow conduction within the circuit. Induction is facilitated by rapid pacing (causing conduction slowing) especially with stimulation close to the tachycardia circuit.
Resetting and Entrainment
The existence of an excitable gap allows reentrant tachycardias to be reset or entrained. During resetting, a critically timed extrastimulus penetrates the excitable gap giving rise to two activation wavefronts: orthodromic and antidromic (Fig. 2-1). The orthodromic wavefront advances and resets the tachycardia, while its antidromic counterpart collides with the tachycardia wavefront. The resetting zone (difference between the longest and shortest coupled extrastimuli that reset tachycardia)
defines the excitable gap. The ability of a premature impulse to reset tachycardia is dependent on the 1) size of the excitable gap, 2) distance between the pacing site and circuit, and 3) electrophysiologic properties (e.g., conduction velocity) of the intervening tissue. A macroreentrant circuit can be reset with fusion (resetting + fusion = macroreentry). Although automatic tachycardias can be reset, they cannot be reset with fusion. Entrainment is continuous resetting of tachycardia by overdrive pacing without tachycardia termination. Entrainment (as determined by presence of any four criteria of transient entrainment) establishes existence of an excitable gap and is specific for a reentrant mechanism.
defines the excitable gap. The ability of a premature impulse to reset tachycardia is dependent on the 1) size of the excitable gap, 2) distance between the pacing site and circuit, and 3) electrophysiologic properties (e.g., conduction velocity) of the intervening tissue. A macroreentrant circuit can be reset with fusion (resetting + fusion = macroreentry). Although automatic tachycardias can be reset, they cannot be reset with fusion. Entrainment is continuous resetting of tachycardia by overdrive pacing without tachycardia termination. Entrainment (as determined by presence of any four criteria of transient entrainment) establishes existence of an excitable gap and is specific for a reentrant mechanism.
Criteria of Transient Entrainment
The four criteria of transient entrainment are 1) constant ECG fusion (except for the last entrained beat, which is unfused and occurs at the pacing cycle length [PCL]), 2) progressive ECG fusion, 3) tachycardia termination associated with localized conduction block to a site activated orthodromically by tachycardia followed by activation of that site from a different direction and with a shorter conduction time, and 4) progressive electrogram (EGM) fusion.8,9,10,11,12,13,14 While it is not always possible to demonstrate these four criteria, the presence of any one of them indicates a reentrant mechanism. The presence of constant and progressive ECG fusion implies that the circuit has separate entrance and exit sites (not to be confused with the entrance and exit sites of the critical isthmus or zone of slow conduction within the circuit). During transient entrainment, pacing stimuli collide with tachycardia outside the circuit (resulting in ECG fusion) while penetrating the circuit within its excitable gap to produce orthodromic and antidromic wavefronts. The antidromic wavefront of the first pacing stimulus (n) collides with tachycardia. Its orthodromic counterpart advances it. Pacing accelerates tachycardia to the pacing rate with each orthodromic (n) wavefront colliding with the subsequent antidromic (n + 1) wavefront. Upon pacing cessation, the last orthodromic wavefront has no antidromic wavefront with which to collide producing an orthodromically activated (unfused) complex occurring at the PCL. Transient entrainment at a fixed PCL causes a stable collision site between orthodromic (n) and antidromic (n + 1) wavefronts (constant ECG [and intracardiac] fusion) (Figs. 2-2, 2-3 and 2-4). Accelerating the pacing rate shifts the collision site farther from the pacing site so that more of the ECG complex is paced (progressive ECG fusion) and more EGM sites are antidromically captured by pacing (progressive EGM fusion) (Figs. 2-5, 2-6, 2-7, 2-8, 2-9 and 2-10). Interruption of tachycardia is associated with localized conduction block to a site orthodromically activated
by tachycardia followed by activation of that site from a different direction and with a shorter conduction time from the pacing stimulus. Transient entrainment from within the circuit (e.g., entrainment with concealed fusion during VT mapping—all pure tachycardia QRS complexes) or from outside the circuit having a single entrance and exit site to the chamber paced (e.g., concealed entrainment of AVNRT from the ventricle—all pure paced QRS complexes) causes orthodromic and antidromic collision exclusively within the circuit and, therefore, absence of paced ECG fusion (hence, the term “concealed”).15,16,17
by tachycardia followed by activation of that site from a different direction and with a shorter conduction time from the pacing stimulus. Transient entrainment from within the circuit (e.g., entrainment with concealed fusion during VT mapping—all pure tachycardia QRS complexes) or from outside the circuit having a single entrance and exit site to the chamber paced (e.g., concealed entrainment of AVNRT from the ventricle—all pure paced QRS complexes) causes orthodromic and antidromic collision exclusively within the circuit and, therefore, absence of paced ECG fusion (hence, the term “concealed”).15,16,17
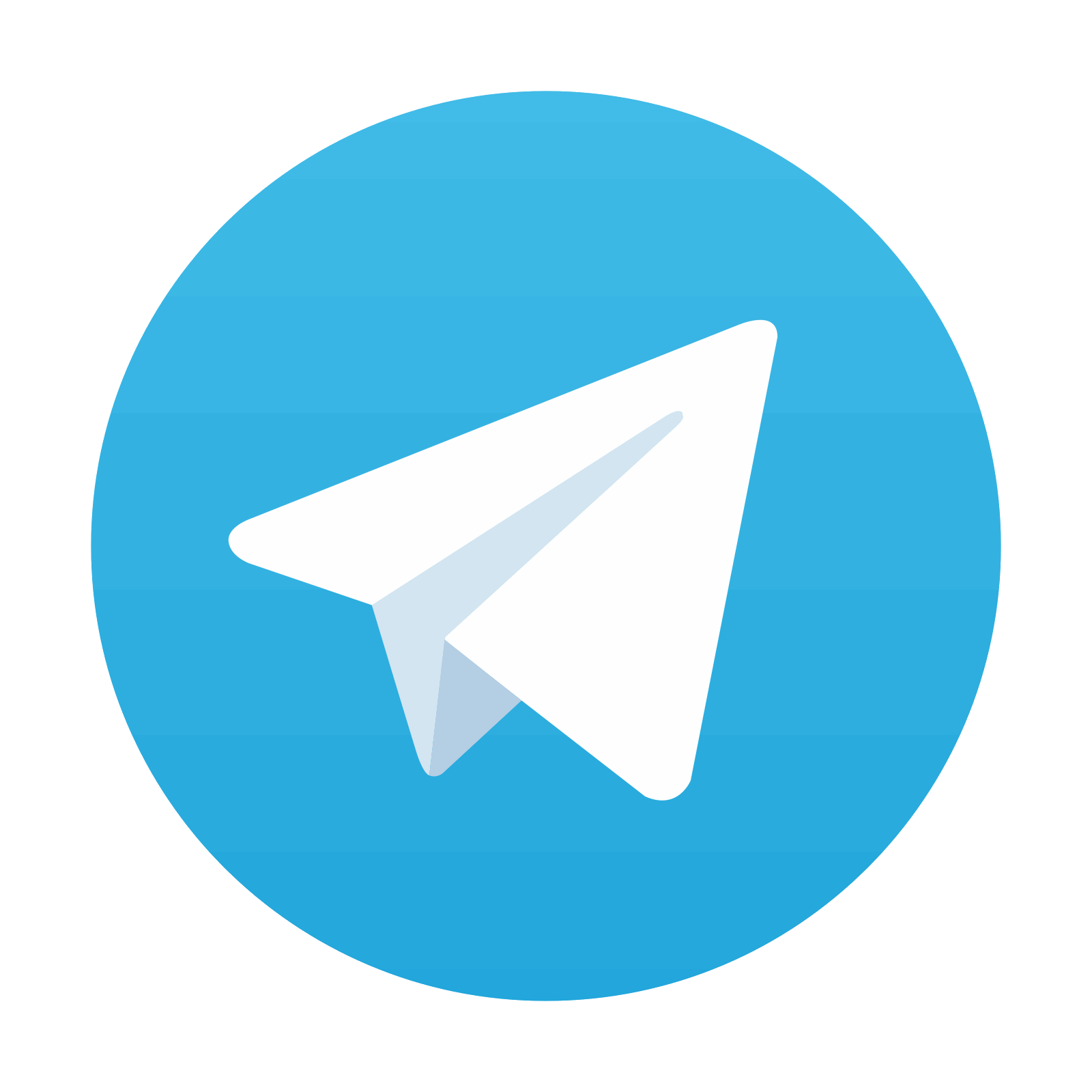
Stay updated, free articles. Join our Telegram channel

Full access? Get Clinical Tree
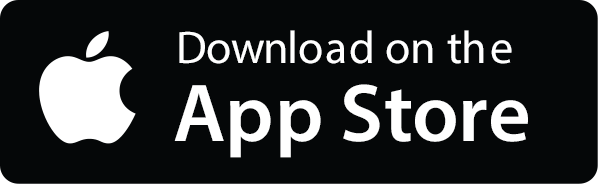
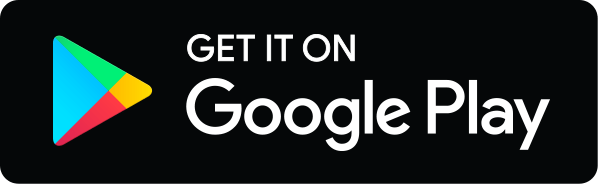
