Mechanisms of Cardiac Arrhythmias
Peter Hanna
Kalyanam Shivkumar
James N. Weiss
INTRODUCTION
For several billion heartbeats in a lifetime, electromechanical coupling ensures normal electrical activity, and activates working myocardium to contract and perform its mechanical function to maintain the circulation. Normal electrical activity necessitates impulse formation and conduction, and electrophysiologic disturbances in impulse formation or conduction result in cardiac arrhythmias that can significantly compromise cardiac contraction (Algorithm 50.1). Mechanisms of such aberrations are discussed in this chapter.
IMPULSE FORMATION
Spontaneous Automaticity
Specialized conducting cells in the heart are found in the sinoatrial (SA) node, atrioventricular (AV) node, and the His-Purkinje system and exhibit automaticity to self-generate spontaneous, rhythmic action potentials. A cardinal attribute of these pacemaking cells is the spontaneous diastolic depolarization during phase 4 that follows an action potential, originally attributed to the “funny” current (If) identified in the late 1970s.1 This current is now known to be mediated by sarcolemmal potassium (K+)/sodium (Na+) hyperpolarization-activated cyclic nucleotide-gated channels (HCN4 in the heart), distinct among voltage-sensitive ion channels in their activation upon hyperpolarization rather than depolarization (Figure 50.1).2,3 Permeable to both Na+ and K+ ions, If is an inward current that depolarizes the diastolic resting potential. Assisted by a host of other sarcolemmal ion channels constituting the membrane clock, If brings the cell to the threshold for voltage-gated L-type calcium Ca2+ channels to generate the action potential upstroke. In the early 2000s, improved confocal microscopic imaging demonstrated that the membrane clock is integrally coupled to a Ca2+ clock that plays an essential shared role in automaticity.4 The Ca2+ clock, driven by progressive Ca2+ release from the sarcoplasmic reticulum (SR) into the cytoplasm during diastole, activates inward current via electrogenic Na+-Ca2+ exchange (ie, three Na+ in, one Ca2+ out) to assist in depolarizing diastolic membrane potential sufficiently to activate voltage-gated L-type Ca2+ channels and generate the upstroke of the action potential.5 Overall, the heart rate is regulated by phosphorylation of key proteins in both clocks in response to changes in autonomic tone and other factors.
![]() ALGORITHM 50.1 Classification of cardiac arrhythmias. APD, action potential duration. |
In tissues with pacemaking ability, the SA node normally has the fastest intrinsic rate and serves as the primary pacemaker. Impairment of SA nodal function results in latent or subsidiary pacemakers in atria or ventricles taking over, albeit at slower rates, with atrial and AV junctional subsidiary pacemakers also responsive to autonomic tone. Normally, quiescent cardiac tissue can also develop automaticity or triggered activity under pathophysiologic conditions or due to a host of genetic abnormalities, as discussed in a later chapter.
![]() FIGURE 50.1 Schematic of regulation of automaticity in pacemaker cells. The membrane and calcium (Ca2+) clocks are coupled to regulate spontaneous, rhythmic action potentials. The membrane clocks involve the HCN4 channels at the sarcolemmal membrane. The calcium clock regulates (1) intracellular Ca2+ cycling between the SR and myoplasm and (2) Ca2+ balance via Ca2+ channels at the sarcolemmal membrane. ATPase, adenosine triphosphatase; cAMP, cyclic adenosine monophosphate; Ca2+, calcium; K+, potassium; M clock, membrane clock; NCX, Na+/Ca2+ exchanger; RyR, ryanodine receptor; SERCA, sarco/endoplasmic reticulum calcium-ATPase; SR, sarcoplasmic reticulum. Created with BioRender.com. |
Changes in Spontaneous Automaticity
The SA node is densely innervated and under neural control that regulates the heart rate. The enhanced automaticity mediates physiologic responses to stress such as exercise, anxiety, or hypovolemia. Autonomic modulation of heart rate occurs through affecting both the Ca2+ and membrane clocks (Figure 50.1). The sympathetic nervous system provides adrenergic input to increase the heart rate through multiple ionic currents, including If, to increase the rate of diastolic depolarization and shorten diastole.4 For example, β-adrenergic stimulation increases intracellular cyclic adenosine monophosphate (cAMP), which then directly binds HCN4 channels to augment If.6 The Ca2+ clock is also modulated by phosphorylation of Ca2+ cycling proteins (eg, phospholamban, L-type Ca2+ channels, and ryanodine receptors) that regulate [Ca2+]i balance and spontaneous SR Ca2+ cycling.7 The resultant changes in rate may be accompanied by shifts in the dominant pacemaking site within the SA node or to a subsidiary pacemaker site. Enhanced automaticity in other foci may cause ectopic beats or rhythms to emerge. For example, insults to the AV node such as acute myocardial infarction, digitalis toxicity, isoproterenol, or cardiac surgery may result in enhanced automaticity. In such instances, the firing rate of the AV junction may supersede that of the SA node and result in an accelerated junctional rhythm.
Conversely, the parasympathetic nervous system activates muscarinic K+ channels and decreases If to decrease the rate of diastolic depolarization and reduce heart rate. Acetylcholine (ACh) binds muscarinic receptors to inhibit adenylate cyclase and reduce cAMP levels at low-moderate vagal stimulation.4 Decreased automaticity of the SA node may further result in escape rhythms to become the dominant rhythm. In short, in each SA nodal pacemaking cycle, the membrane and calcium clocks are continuously coupled and are modulated by the autonomic nervous system to maintain spontaneous, rhythmic action potentials across a wide physiologic range.
Enhanced Automaticity
Abnormal automaticity may also develop in normally non-pacemaking cells. A high resting K+ conductance together with a high intracellular-to-extracellular ratio of K+ maintained by Na+-K+ adenosine triphosphatase (ATPase) generate a negative resting membrane potential that approaches the K+ equilibrium potential (˜-95 mV by the Nernst equation), rendering working atrial and ventricular cardiomyocytes quiescent during diastole. However, multiple different mechanisms can cause abnormal automaticity in such normally quiescent cells, such as membrane depolarization.8 Some factors, such as hypokalemia, can also induce automaticity in association with membrane hyperpolarization. At normally polarized resting
membrane potentials, Na+ channels are activated and contribute to a rapid upstroke. At depolarized resting membrane potentials, Ca2+ currents mediate a slow upstroke.
membrane potentials, Na+ channels are activated and contribute to a rapid upstroke. At depolarized resting membrane potentials, Ca2+ currents mediate a slow upstroke.
Automaticity of most pacemakers, including the sinus node, is inhibited by overdrive suppression and remains latent unless their intrinsic rate exceeds that of the sinus node.9 Overdrive suppression of automaticity can result from the faster pacing rate, causing intracellular Na+ accumulation that increases outward current via electrogenic Na+-K+ ATPase activity. The resulting hyperpolarizing current opposes phase 4 depolarization to slow spontaneous diastolic depolarization. Even after overdrive pacing terminates, continued Na+-K+ ATPase activity suppresses the rate of diastolic depolarization and heart rate until the elevated intracellular Na+ levels normalize. The faster the rate or longer the duration of overdrive suppression, the greater the intracellular Na+ accumulation and duration of increased Na+-K+ ATPase activity, resulting in a longer period of quiescence after cessation of stimulation.
Latent pacemakers are typically suppressed because of resetting by the faster dominant pacemaker, whereas a latent pacemaker site can also exhibit entrance block that insulates it either fully or partially from being reset by the dominant pacemaker. The result is a parasystole or modulated parasystole, an ectopic rhythm that marches independently or quasi-independently through the dominant rhythm. This situation can arise when the dominant pacemaker is surrounded by ischemic, infarcted, or otherwise electrically compromised tissues that inhibit resetting of the latent pacemaker. This activity is typically characterized by premature ventricular contractions that march through the sinus rate and rarely result in a life-threatening ventricular arrhythmia.
Triggered Activity
Afterdepolarizations are abnormal impulses that are classified as early afterdepolarization (EAD) or delayed afterdepolarization (DAD) depending on whether they arise during or following an action potential, respectively. Unlike automaticity, they require an antecedent action potential, whether spontaneous, triggered, or artificially paced. If an EAD or DAD depolarizes the membrane potential to threshold, an action potential results, and is referred to as triggered activity (Figure 50.2). In cardiac tissue, such triggered beats can propagate from regions with EADs into regions without EADs, generating tachycardias and initiating reentry when dispersion of refractoriness is sufficient.10 Unfortunately, EADs and DADs cannot be directly detected clinically from an electrocardiogram.
Early Afterdepolarizations
EADs occur during phase 2 or phase 3 of the cardiac action potential before repolarization is complete. They typically occur in the setting of a prolonged action potential such as in long QT syndromes. Multiple conditions may result in QT prolongation including myocardial injury, electrolyte disturbances (hypokalemia, hypomagnesemia), acidosis, hypoxia, catecholamines, QT prolonging drugs (antiarrhythmic and noncardiac drugs), left ventricular hypertrophy, heart failure, and congenital ion channels defects. Clinically, EADs can cause polymorphic ventricular tachycardias and torsades de pointes when QT prolongation concomitantly increases dispersion of refractoriness and makes tissue vulnerable to reentry.
Action potential duration (APD) prolongation occurs when net outward current during the action potential plateau is reduced so that repolarization reserve becomes impaired. The imbalance can be caused by uncompensated increases in inward currents such as the late sodium (INa) or Ca2+ currents (ICa or INCX) or decreases in outward repolarizing potassium currents (IKr, IKS, IK1). If sufficiently severe, reactivation of the L-type Ca2+ channels during phase 2 or 3 can reverse repolarization altogether and cause the upstroke of EAD11 to trigger a new action potential that propagates into surrounding tissue that has already repolarized.
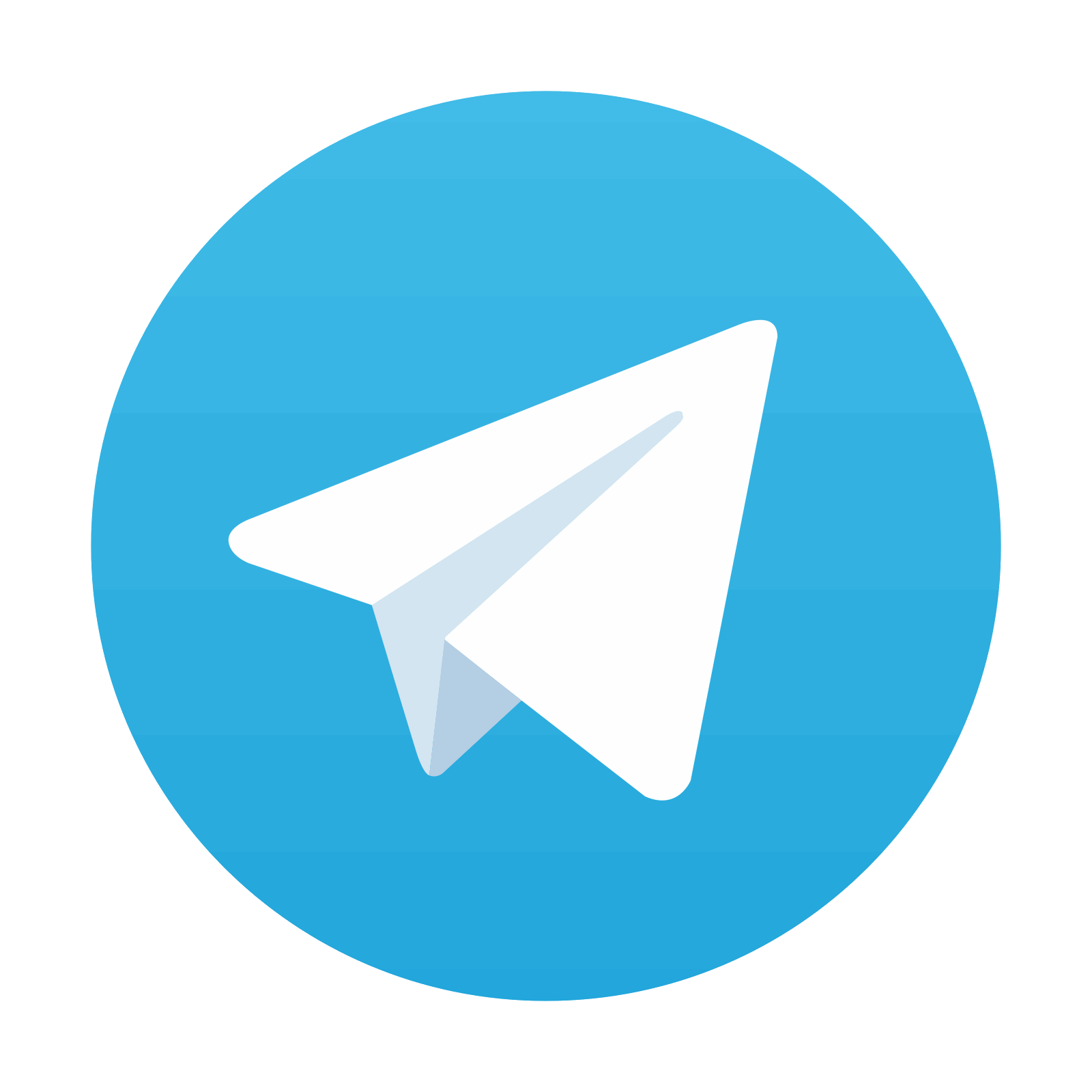
Stay updated, free articles. Join our Telegram channel

Full access? Get Clinical Tree
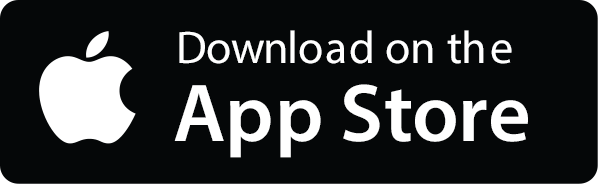
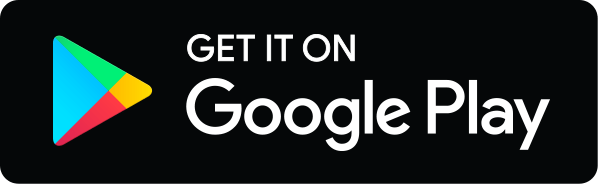