Background
Clinical experience suggests that measurement of left ventricular (LV) ejection fraction (EF) using two-dimensional echocardiography (2DE) is often at variance with results of three-dimensional echocardiography (3DE) in patients who have undergone heart transplantation (HT). The aim of this study was to test the hypothesis that LV mechanical dyssynchrony and abnormal regional strain are present in asymptomatic pediatric HT patients and that they promote errors in the measurement of LV function when 2DE is used.
Methods
HT subjects and normal volunteer children were prospectively enrolled. All had normal estimated right ventricular systolic pressure and function. LV EF, global and regional strain, and systolic dyssynchrony index (SDI) were quantified using real time 3DE. SDI was determined from volume-time curves of the 16 LV segments and expressed as the standard deviation of the heart rate–corrected time to reach minimal segmental systolic volume. Septal strain was defined as the average of five segments in the interventricular septum. In addition to 3DE, the Teichholz, biplane Simpson, and bullet (5/6 area-length) methods were used to measure EF using 2DE in each subject. Ninety-three examinations were done: 40 in the 40 normal control subjects (mean age, 14.6 ± 10.6 years; 10 male) and 53 in 36 HT subjects (mean age, 10.3 ± 6.2 years; 21 male).
Results
SDI was greater in HT patients (mean, 6.2 ± 4.3%) than in normal controls (mean, 2.2 ± 1.1%) ( P < .0001). Global and septal strain was lower in HT patients than in normal controls. EF divergence (absolute difference between two- and three-dimensional EFs) was greater in HT patients (mean, 3.8 ± 2.2%) than in normal controls (mean, 0.7 ± 0.5%) ( P < .0001). EF divergence had a strong positive correlation with SDI (adjusted r 2 = 0.46, P < .001) and negative correlations with all measures of strain (range of adjusted r 2 values, 0.13–0.32). SDI had no particular relation to LV mass or to QRS duration.
Conclusions
Children after HT have abnormal LV mechanics characterized by greater dyssynchrony and lower strain. These features correlate with, and possibly contribute to differences between measurements by 2DE and 3DE. EF should be calculated using 3DE in this population and others with dyssynchrony and regional strain abnormalities.
Pediatric heart transplantation (HT) is the widely accepted destination therapy for children with end-stage cardiac disease. Ten-year outcomes data indicate significant improvement in survival for children with HT; however, graft dysfunction can occur both early and late after HT. Ventricular dysfunction may occur early after HT because of the transplantation ischemic period, early rejection, fluid overload, rhythm disturbances, or other factors. Late left ventricular (LV) dysfunction can result from (1) cardiac ischemia, including changes in myocardial tissue characteristics such as hypertrophy secondary to ischemia-reperfusion injury; (2) abnormal electrical activation including bundle branch block and atrioventricular dissociation due to conduction system injury; (3) right ventricular dysfunction and consequent adverse ventricular-ventricular interactions; (4) multiple episodes of rejection and associated coronary vasculopathy; or (5) cardiac effects of immunosuppressive agents such as corticosteroids. Assessment of cardiac graft function following pediatric HT is therefore an important aspect of post-HT care.
Echocardiography plays a major role in cardiac surveillance of pediatric HT patients. In pediatric patients, especially infants and small children, the risks for cardiac catheterization and endomyocardial biopsy are heightened, so monitoring for rejection, vasculopathy, or early graft dysfunction is often primarily noninvasive via transthoracic echocardiography. Abnormal myocardial mechanics after pediatric HT have been demonstrated using two-dimensional (2D) echocardiography (2DE), but newer techniques such as deformation imaging using speckle-tracking echocardiography (STE) may be more sensitive to dysfunction than is conventional 2DE. Real-time three-dimensional (3D) echocardiography (3DE) confers the advantage of tracking myocardial motion in three dimensions and can be used to quantify LV ejection fraction (EF) and global and regional strain in three dimensions. It also provides a measure of LV mechanical dyssynchrony using the systolic dyssynchrony index (SDI). Three-dimensional echocardiography is particularly advantageous because it enables imaging of the entire ventricle in a single data set, thus allowing rapid semiautomated quantification.
LV wall motion abnormalities, particularly abnormal interventricular septal motion, are frequently observed in transplanted hearts. It is well recognized that abnormal LV geometry introduces inaccuracies in 2D echocardiographic determinations of LV EF, while 3DE-derived EF measurements are unaffected by LV geometry or apical foreshortening. Recent adult guidelines recommend 3DE rather than 2DE for quantification of LV volumes and EF when feasible. We hypothesized that LV mechanical dyssynchrony and abnormal regional strain are present in asymptomatic pediatric HT patients and that they promote errors in 2D echocardiographic measurement of LV systolic function. The objectives of this study were twofold: (1) to characterize LV regional and global mechanics in pediatric HT using 3DE and (2) to identify and measure any divergence between EF by 2DE and 3DE in pediatric HT.
Methods
Patients
We prospectively enrolled orthotopic HT recipients and normal control subjects from September 2013 to October 2014 at two centers. The HT patients were recruited only during routine clinic visits or after routine surveillance biopsy, not if they were hospitalized for any acute illness, including symptomatic or suspected rejection episode. Research imaging was performed as part of a routine clinically indicated echocardiographic examination during the visit, and patient follow-up was not prescribed by a specific protocol. The only exclusion criterion was heart failure, defined as LV EF < 50% as measured by the 2D biplane Simpson’s method. Normal control subjects were pediatric patients who underwent echocardiography (for indications such as heart murmur) and had structurally and functionally normal hearts. Control subjects were not age or gender matched. In all subjects, 2DE and 3DE were performed at a single setting. Four sonographers with experience in 3DE acquired all images. Clinical data, including demographics, age, height, and weight, were collected. Body surface area was calculated using the Haycock formula. Electrocardiograms obtained within 3 months of the study in each HT patient were selected from the medical records for the purpose of obtaining the QRS duration.
Seventeen HT patients who returned for clinical follow-up during the time frame of the study had a second set of 2D and 3D echocardiographic data at a median interval of 4.5 months (range, 3.6–8.0 months). Because of the potential for myocardial functional changes in the transplantation population to occur in a relatively short time frame, we elected to include these echocardiograms as de novo examinations in this investigation. Thus, 93 examinations were done in 76 subjects, consisting of 36 HT patients (mean age, 10.3 ± 6.2 years; 21 male; 53 examinations) and 40 normal control subjects (mean age, 14.6 ± 10.6 years; 10 male; 40 examinations). The institutional review boards at the University of Nebraska Medical Center and Children’s Mercy Hospitals and Clinics approved the study protocol and data transfer, and all enrolled patients and control subjects provided written informed consent.
Echocardiography
Echocardiography was performed using an Acuson SC2000 (Siemens Medical Solutions USA, Inc, Mountain View, CA) or an iE33 (Philips Medical Systems, Andover, MA). Images were optimized for gain, compression, depth, and sector width and acquired at frame rates of 50 to 80 frames/sec and 20 to 40 volumes/sec for 2DE and 3DE, respectively. For the Philips system, the X5-1 and X7-2 transducers were used for 3DE, while the S5-1 and S8-3 transducers were used for 2DE, depending on body size. For the Siemens system, the 8V3 and 4V1 transducers were used for 2DE, and the 4Z1 transducer was used for 3DE. Apical four-chamber, two-chamber, and parasternal long-axis and short-axis images were acquired during quiet respiration for 2D echocardiographic analysis. All subjects had normal right ventricular systolic function by qualitative assessment and normal estimated right ventricular systolic pressure by tricuspid regurgitation Doppler (maximal velocity < 2.5 m/sec). Three full-volume 3D echocardiographic data sets of the left ventricle were acquired from the apical four-chamber plane during quiet respiration from three consecutive cardiac cycles (single-beat captures, stitched).
Two-Dimensional and 3D Echocardiographic Analysis
From the M-mode and 2D echocardiographic acquisitions, a single observer (L.L.) measured LV EF offline using the following methods: Teichholz, bullet (5/6 area-length), and biplane Simpson. The same observer analyzed 3D echocardiographic data sets using 4D LV-Analysis 3 software (Image Arena version 4.6; TomTec Imaging Systems, Unterschleissheim, Germany) and measured LV myocardial strain, LV dyssynchrony, LV mass, and LV EF. The 3D echocardiographic full volume was postprocessed by placing points at the LV apex, mitral valve annulus, and aortic valve in a single frame. This was followed by semiautomated endocardial and epicardial tracking in the four-chamber, three-chamber, and two-chamber views in end-systole and end-diastole. Manual corrections were performed to achieve adequate tracking by the 4D LV-Analysis algorithm ( Figure 1 ). Global (longitudinal, circumferential, and radial) and regional strain, EF, mass, and SDI for the left ventricle were calculated. SDI was determined from volume-time curves of the 16 LV segments and expressed as the standard deviation of the heart rate (HR)–corrected time to reach minimal segmental systolic volume ( Figure 2 ). From the regional strain output, longitudinal, circumferential, and radial strain for the interventricular septum (septal strain) was calculated as the average of five segments constituting the interventricular septum ( Figure 3 ). To determine the reproducibility of 3D echocardiographic parameters, a blinded second observer (S.K.) repeated SDI and strain analysis in 10 randomly chosen transplantation studies, and the first observer repeated the analysis after a 3-month interval.



Statistical Analysis
Comparisons of categorical variables between groups were made using the χ 2 method. Unpaired Student’s t tests were used to compare LV SDI and global and regional strain between the HT and control groups. Linear regressions were used to analyze correlations between 2D and 3D echocardiographic measurements. The EF divergence (absolute difference between 3D EF and the average of EF by 2D methods) was calculated. Correlations between EF divergence and SDI and between the various measures of strain and SDI were evaluated. For each regression, the coefficient of determination ( r 2 ) was calculated. To assess for potential disproportionate influence of outliers, the regression of EF divergence and SDI was repeated without the two outliers for which SDI exceeded 20%. To assess potential group dependent difference in the correlation of EF divergence with SDI, the regression was repeated using control subjects alone and using HT patients alone. Bland-Altman limit-of-agreement analysis was used for inter- and intraobserver reliability. Intraclass correlation coefficients were calculated to test measurement variability. P values < .05 were considered statistically significant. Statistical analyses were performed using SPSS version 17.0 (IBM, Armonk, NY).
Results
Demographic data for HT patients and control subjects are summarized in Table 1 . The SDI and peak global and septal strain (longitudinal, circumferential, and radial) obtained by 4D LV-Analysis in HT patients compared with normal control subjects are shown in Table 2 .
Variable | HT group | Control group | P |
---|---|---|---|
Number of subjects | 36 | 40 | |
Male/female | 21/15 | 10/30 | .003 |
Age (y) | 10.3 ± 6.2 | 14.6 ± 10.6 | .033 |
Height (cm) | 130.7 ± 33.4 | 140.3 ± 32.2 | .207 |
Weight (kg) | 37.9 ± 26.8 | 47.4 ± 29.0 | .142 |
BSA (m 2 ) | 1.0 ± 0.6 | 1.3 ± 0.6 | .054 |
SBP (mm Hg) | 107.6 ± 14.1 | 104.9 ± 10.5 | .631 |
DBP (mm Hg) | 63.6 ± 11.3 | 60.4 ± 9.8 | .542 |
Variable | HT group | Control group | P |
---|---|---|---|
SDI | 6.2 ± 4.3 | 2.2 ± 1.1 | <.001 |
Global strain | |||
Radial | 35.2 ± 5.7 | 42.2 ± 4.3 | <.001 |
Circumferential | −23.5 ± 4.6 | −28.0 ± 4.1 | <.001 |
Longitudinal | −18.3 ± 3.5 | −22.0 ± 3.0 | <.001 |
Septal strain | |||
Radial | 29.9 ± 8.7 | 40.2 ± 5.8 | <.001 |
Circumferential | −20.2 ± 7.1 | −27.4 ± 5.6 | <.001 |
Longitudinal | −16.3 ± 5.0 | −20.7 ± 3.7 | <.001 |
2D EF | |||
Teichholz | 57.7 ± 5.3 | 61.2 ± 4.3 | .003 |
Bullet | 58.5 ± 5.5 | 61.5 ± 4.4 | .013 |
Biplane Simpson | 57.8 ± 4.7 | 61.1 ± 4.0 | .006 |
3D EF | 54.3 ± 6.0 | 61.5 ± 4.3 | <.001 |
EF divergence | 3.8 ± 2.2 | 0.7 ± 0.5 | <.001 |
SDI was greater in HT patients (6.2 ± 4.3%) compared with normal controls (2.2 ± 1.1%) ( P < .0001). Global and septal strain was lower in HT patients than in normal control subjects. The 2D and 3D EF data are listed in Table 2 . EF divergence was greater in HT patients (3.8 ± 2.2%) than in normal control subjects (0.7 ± 0.5%) ( P < .0001).
EF divergence had a moderate positive correlation with SDI (adjusted r 2 = 0.46, P < .001) ( Figure 4 ). When the two outliers ( Figure 4 ) for whom SDI was >20% were excluded from the analysis, r 2 was somewhat lower (0.41). EF divergence had weak negative correlations with all measures of strain (range of adjusted r 2 values, 0.13–0.32) ( Figures 5 and 6 ).


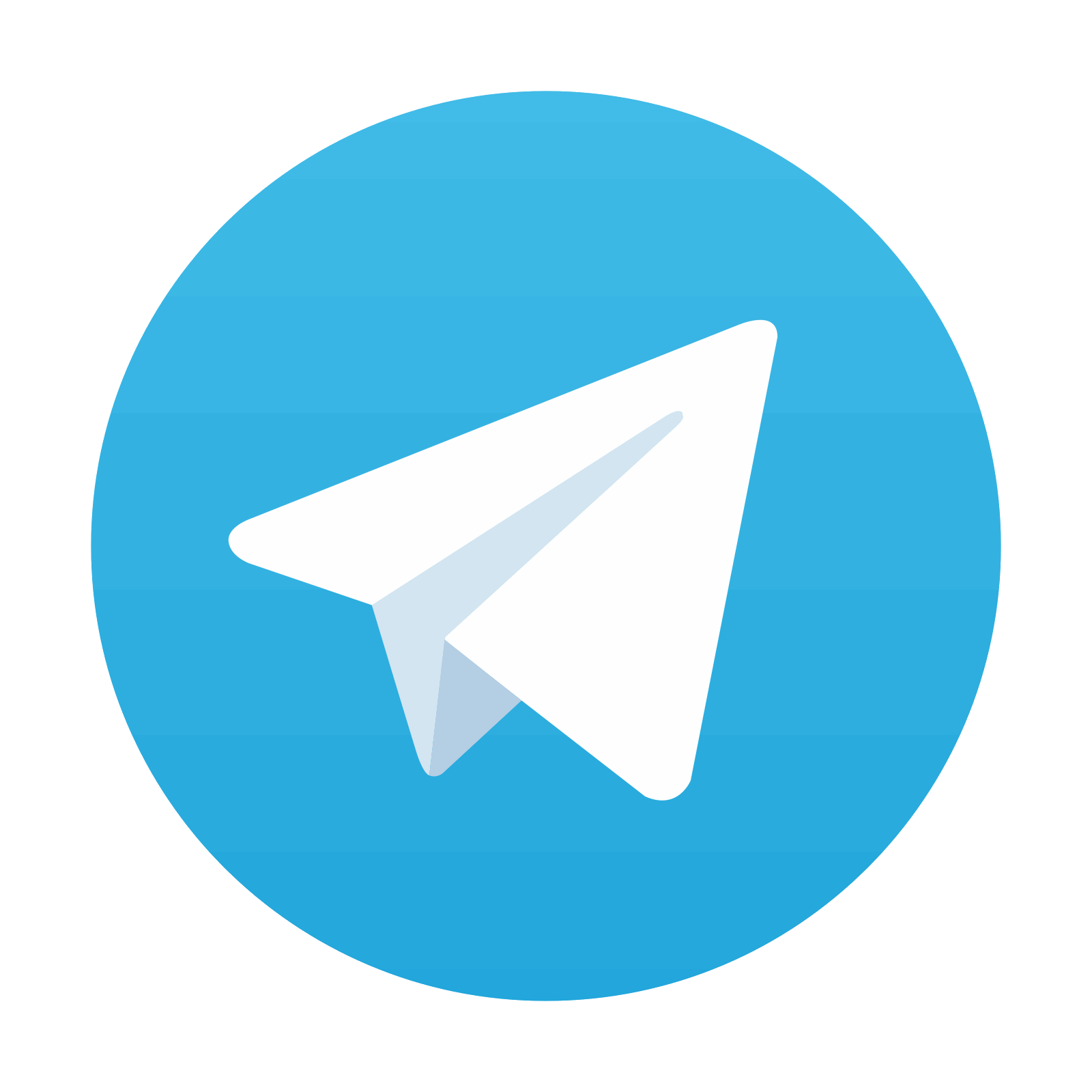
Stay updated, free articles. Join our Telegram channel

Full access? Get Clinical Tree
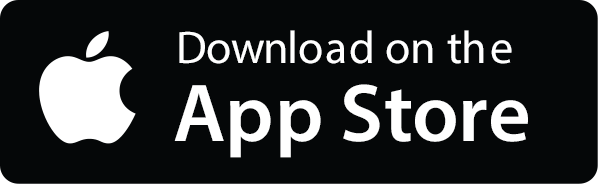
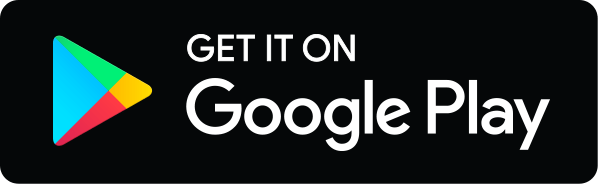
