Background
Previous studies have demonstrated that global longitudinal strain (GLS) is altered in patients with hypertrophic cardiomyopathy (HCM) in comparison with athletes. Nevertheless, these results rely on studies comparing sedentary patients with HCM with healthy athletes. The aims of this study were to confirm these findings in an appropriate group of athletes with HCM and to study the additive value of novel parameters (i.e., mechanical dispersion and exercise GLS).
Methods
Thirty-six athletes with HCM were prospectively included and were compared with 36 sedentary patients with HCM, 36 healthy athletes, and 36 sedentary control subjects of similar age. Athlete groups had similar training duration and HCM groups similar maximal wall thickness. All underwent echocardiography at rest and during submaximal exercise. GLS was assessed; the SD of time to maximal myocardial shortening of longitudinal strain was calculated as a parameter of mechanical dispersion.
Results
The HCM sedentary group showed the lowest resting and exercise GLS. Resting GLS was not different between athletes with HCM and the two control groups, but exercise GLS enabled the differentiation of athletes with HCM from healthy athletes. Mechanical dispersion was higher in both HCM groups compared with both control groups at rest and during exercise. Receiver operating characteristic analysis in the athlete groups demonstrated that resting mechanical dispersion (area under the curve = 0.949 ± 0.023) had better ability to identify HCM compared with GLS at rest (area under the curve = 0.644 ± 0.069) ( P < .001) or during exercise (area under the curve = 0.706 ± 0.066) ( P < .005).
Conclusions
In athletes, normal resting GLS does not rule out the diagnosis of HCM. Mechanical dispersion of longitudinal strain seems to be a promising tool for the diagnosis of HCM in athletes.
Introduction
Attention ASE Members:
The ASE has gone green! Visit www.aseuniversity.org to earn free continuing medical education credit through an online activity related to this article. Certificates are available for immediate access upon successful completion of the activity. Nonmembers will need to join the ASE to access this great member benefit!
The use of strain analysis is now recommended in HCM as a clinical tool for the evaluation of LV systolic function. Thus, a decrease in global longitudinal strain (GLS) has been proposed to distinguish HCM from athlete’s heart. Furthermore, it has been shown that because of myocardial disarray and fibrosis, systolic contraction in HCM is heterogeneous, in contrast to the homogenous contraction in athletes. Mechanical dispersion assessed by two-dimensional strain reflects heterogeneous myocardial contraction.
The main weakness of these studies was that they compared sedentary patients with HCM with healthy athletes. Indeed, it has been reported that some athletes with proven HCM are able to exercise at very high levels, probably because of good LV compliance. In the same way, a recent study demonstrated that athletes with HCM have better systolic and diastolic function than sedentary patients with HCM. But to our knowledge, resting GLS has not been compared between athletes with HCM and sedentary patients with HCM.
Moreover, it must be underlined that previous results concerned only the resting state, although the evaluation of athlete’s heart response to exercise seems promising. GLS analysis during exercise is still scarce, even if its feasibility and its interest have already been reported in previous studies in healthy athletes and in patients with HCM.
Thus, the aims of this study were (1) to compare resting GLS in sedentary patients with HCM and in athletes with HCM and (2) to study the additive value of mechanical dispersion and of exercise GLS in the diagnosis of HCM. For these purposes, four populations, athletes with HCM, sedentary patients with HCM, healthy athletes, and sedentary control subjects were included.
Methods
Study Population
Athletes with HCM
From December 2008 to May 2013, 36 athletes with confirmed diagnoses of HCM were prospectively enrolled in the Department of Sport Medicine of our institution ( Figure 1 ). Athletes had trained >4 hr/wk during the past 5 years and regularly took part in competition at inclusion. The diagnosis of HCM was performed because of symptoms or workup of cardiovascular abnormalities (mainly electrocardiographic abnormalities) during competitive sports’ preparticipation evaluation. The diagnosis of HCM was based on LV hypertrophy (LVH) >16 mm in any myocardial segment, as assessed on echocardiography and confirmed by cardiac magnetic resonance imaging (CMR), in the absence of another cardiac disorder or systemic condition able to produce the same level of LVH. In cases of mild LVH (15 mm), HCM was diagnosed with an association of features, including (1) identification of HCM in a first-degree relative, (2) an asymmetric pattern of LVH (i.e., apical hypertrophy), (3) dynamic LV outflow tract obstruction, and (4) late gadolinium enhancement (LGE) on CMR. Importantly, the isolated association of electrocardiographic anomalies and mild concentric LVH was not considered for diagnosis of HCM.

Sedentary Patients with HCM
To establish potential differences between individuals with HCM engaged in competitive sport and those who are sedentary, athletes with HCM were compared with 36 sedentary patients with HCM of similar age and maximal wall thickness on echocardiography selected from 156 patients with HCM enrolled during the same period of time at our regional HCM competence center. These subjects practiced ≤1 hr/wk of moderate physical activity during all their lives.
Exclusion criteria for patients with HCM were depressed LV ejection fraction (≤50%), persistent atrial fibrillation, history of coronary artery disease, and inability to perform a maximal stress test.
Healthy Athlete Control Subjects
Athletes with HCM were compared with 36 athletes of similar ages, types of sports, and training durations, selected from a group of 75 athletes. Exclusion criteria for healthy athletes were borderline wall thickness of 14 to 15 mm, in which HCM diagnosis might have been missed by current methods.
Healthy Sedentary Control Subjects
Thirty-six sedentary control subjects with similar ages were included from a group of 60 sedentary control subjects.
All subjects provided informed consent to participate in the study. The study was performed in accordance with the principles outlined in the Declaration of Helsinki on research in human subjects (Commission Nationale de l’Informatique et des Libertés declaration no. 909378).
Study Protocol
All subjects underwent, the same day, a clinical examination, resting 12-lead electrocardiography, transthoracic echocardiography at rest and during submaximal exercise, and a maximal cardiopulmonary exercise test. All subjects with HCM also underwent CMR the same day. Patients with HCM were asked to withhold β-blockers and calcium channel antagonists 24 hours before evaluation.
Echocardiography
All subjects underwent resting and exercise echocardiography using a Vivid 9 (GE Vingmed Ultrasound AS, Horten, Norway). Exercise echocardiography was performed on an integrated electromagnetic cycle ergometer tilt table (Ergometrics, Lynnwood, WA). The initial workload was 30 W, with a 30-W increment every 2 min. As previously described, echocardiographic data were recorded at a submaximal stage with a stable heart rate between 100 and 120 beats/min to enable accurate strain measurements. The electrocardiogram was recorded continuously, and blood pressure was manually measured every 2 min.
The standard acquisitions, parasternal, apical, and subcostal views, were recorded during resting and exercise echocardiography. All data were stored on a workstation for offline analysis (EchoPAC BT12; GE Vingmed Ultrasound AS) by a cardiologist blinded to the clinical data. The conventional analysis of the echocardiogram preceded the two-dimensional strain analysis. For each measurement, at least two cardiac cycles were averaged. LV end-diastolic diameter and maximal end-diastolic LV wall thickness (MWT) were measured in parasternal views. LV end-diastolic and end-systolic volumes and ejection fraction were measured using the biplane method of disks. Peak E-wave and A-wave velocities of the mitral inflow were measured using pulsed-wave Doppler. Tissue Doppler imaging was recorded at the level of septal and lateral mitral annulus, to obtain the average peak velocities during systole (s′) and early (e′) diastole. The E/e′ ratio was calculated to assess LV filling pressure. LV outflow velocities were measured at rest and during exercise.
Longitudinal myocardial deformations were evaluated from standard two-dimensional images (at frame rates of 60 to 90 sec −1 ), on the basis of the speckle-tracking approach. GLS was the average of the 18 segmental strain values from the apical four-, three-, and two-chamber views. Postsystolic shortening was not included in the global strain analysis. The time to maximal myocardial shortening, including postsystolic shortening if present, was measured from the electrocardiographic onset Q/onset R wave in the 18 LV segments ( Figure 2 ). As proposed, we used the SD of the 18 time intervals to maximal myocardial shortening to quantify LV mechanical dispersion.

Cardiopulmonary Exercise Test
All subjects performed a progressive maximal exercise test on an ergocycle (ERG 900; Jaeger, Hochberg, Germany) according to the recommendations of Wasserman et al . Exercise was symptom limited or stopped at exhaustion. A 6-min passive recovery period was also recorded. Breath-by-breath gas exchanges were analyzed using an Oxycon device (Jaeger), and the electrocardiogram (CardioSys; Marquette-Hellige, Freiburg, Germany) was continuously monitored, in search of arrhythmias and/or repolarization alterations. Blood pressure was measured every 2 min using a manual manometer. Maximal oxygen uptake peak was expressed as a percentage of predicted value.
CMR
All patients with HCM underwent CMR using a Siemens Avanto 3-T CMR machine (Siemens Healthcare, Erlangen, Germany). Cine-mode sequences were acquired in the short-axis, four-chamber, and LV long-axis views. An LGE sequence to identify fibrosis was performed in the three cardiac planes 5 to 10 min after 0.1 mmol/kg gadolinium contrast injection on the basis of CMR society guidelines ; LGE was analyzed qualitatively. LV volume, mass, and function were quantified using customized analysis software by a blinded, single experienced investigator. Diastolic wall-to-volume ratio was measured.
Statistical Analysis
Gaussian distribution of all continuous variables was confirmed with the Kolmogorov-Smirnov test, and values are reported as mean ± SD. Comparisons among the four groups were performed using analyses of variance, followed when appropriate by Bonferroni tests. Comparisons between the two HCM groups were performed using Student’s t test and the χ 2 test. A linear mixed model correcting for group, heart rate, and level of exercise was performed to explain the mechanical dispersion changes induced by exercise.
Receiver operating characteristic (ROC) curves were created, and areas under curves were calculated for the ability of GLS and mechanical dispersion a rest and exercise to identify HCM. A two-tailed P value < .05 was considered to indicate statistical significance.
Inter- and intraobserver variability of GLS and mechanical dispersion were expressed by intraclass correlation coefficients and Bland-Altman plots at rest and during submaximal exercise in three subjects randomly selected from each group ( n = 12) ( Figure 3 ).


Statistical analysis was performed using SPSS version 20.0 (SPSS, Chicago, IL). ROC curve analysis and pairwise comparison were performed using MedCalc version 15.2.2 (MedCalc Software, Ostend, Belgium).
Methods
Study Population
Athletes with HCM
From December 2008 to May 2013, 36 athletes with confirmed diagnoses of HCM were prospectively enrolled in the Department of Sport Medicine of our institution ( Figure 1 ). Athletes had trained >4 hr/wk during the past 5 years and regularly took part in competition at inclusion. The diagnosis of HCM was performed because of symptoms or workup of cardiovascular abnormalities (mainly electrocardiographic abnormalities) during competitive sports’ preparticipation evaluation. The diagnosis of HCM was based on LV hypertrophy (LVH) >16 mm in any myocardial segment, as assessed on echocardiography and confirmed by cardiac magnetic resonance imaging (CMR), in the absence of another cardiac disorder or systemic condition able to produce the same level of LVH. In cases of mild LVH (15 mm), HCM was diagnosed with an association of features, including (1) identification of HCM in a first-degree relative, (2) an asymmetric pattern of LVH (i.e., apical hypertrophy), (3) dynamic LV outflow tract obstruction, and (4) late gadolinium enhancement (LGE) on CMR. Importantly, the isolated association of electrocardiographic anomalies and mild concentric LVH was not considered for diagnosis of HCM.
Sedentary Patients with HCM
To establish potential differences between individuals with HCM engaged in competitive sport and those who are sedentary, athletes with HCM were compared with 36 sedentary patients with HCM of similar age and maximal wall thickness on echocardiography selected from 156 patients with HCM enrolled during the same period of time at our regional HCM competence center. These subjects practiced ≤1 hr/wk of moderate physical activity during all their lives.
Exclusion criteria for patients with HCM were depressed LV ejection fraction (≤50%), persistent atrial fibrillation, history of coronary artery disease, and inability to perform a maximal stress test.
Healthy Athlete Control Subjects
Athletes with HCM were compared with 36 athletes of similar ages, types of sports, and training durations, selected from a group of 75 athletes. Exclusion criteria for healthy athletes were borderline wall thickness of 14 to 15 mm, in which HCM diagnosis might have been missed by current methods.
Healthy Sedentary Control Subjects
Thirty-six sedentary control subjects with similar ages were included from a group of 60 sedentary control subjects.
All subjects provided informed consent to participate in the study. The study was performed in accordance with the principles outlined in the Declaration of Helsinki on research in human subjects (Commission Nationale de l’Informatique et des Libertés declaration no. 909378).
Study Protocol
All subjects underwent, the same day, a clinical examination, resting 12-lead electrocardiography, transthoracic echocardiography at rest and during submaximal exercise, and a maximal cardiopulmonary exercise test. All subjects with HCM also underwent CMR the same day. Patients with HCM were asked to withhold β-blockers and calcium channel antagonists 24 hours before evaluation.
Echocardiography
All subjects underwent resting and exercise echocardiography using a Vivid 9 (GE Vingmed Ultrasound AS, Horten, Norway). Exercise echocardiography was performed on an integrated electromagnetic cycle ergometer tilt table (Ergometrics, Lynnwood, WA). The initial workload was 30 W, with a 30-W increment every 2 min. As previously described, echocardiographic data were recorded at a submaximal stage with a stable heart rate between 100 and 120 beats/min to enable accurate strain measurements. The electrocardiogram was recorded continuously, and blood pressure was manually measured every 2 min.
The standard acquisitions, parasternal, apical, and subcostal views, were recorded during resting and exercise echocardiography. All data were stored on a workstation for offline analysis (EchoPAC BT12; GE Vingmed Ultrasound AS) by a cardiologist blinded to the clinical data. The conventional analysis of the echocardiogram preceded the two-dimensional strain analysis. For each measurement, at least two cardiac cycles were averaged. LV end-diastolic diameter and maximal end-diastolic LV wall thickness (MWT) were measured in parasternal views. LV end-diastolic and end-systolic volumes and ejection fraction were measured using the biplane method of disks. Peak E-wave and A-wave velocities of the mitral inflow were measured using pulsed-wave Doppler. Tissue Doppler imaging was recorded at the level of septal and lateral mitral annulus, to obtain the average peak velocities during systole (s′) and early (e′) diastole. The E/e′ ratio was calculated to assess LV filling pressure. LV outflow velocities were measured at rest and during exercise.
Longitudinal myocardial deformations were evaluated from standard two-dimensional images (at frame rates of 60 to 90 sec −1 ), on the basis of the speckle-tracking approach. GLS was the average of the 18 segmental strain values from the apical four-, three-, and two-chamber views. Postsystolic shortening was not included in the global strain analysis. The time to maximal myocardial shortening, including postsystolic shortening if present, was measured from the electrocardiographic onset Q/onset R wave in the 18 LV segments ( Figure 2 ). As proposed, we used the SD of the 18 time intervals to maximal myocardial shortening to quantify LV mechanical dispersion.
Cardiopulmonary Exercise Test
All subjects performed a progressive maximal exercise test on an ergocycle (ERG 900; Jaeger, Hochberg, Germany) according to the recommendations of Wasserman et al . Exercise was symptom limited or stopped at exhaustion. A 6-min passive recovery period was also recorded. Breath-by-breath gas exchanges were analyzed using an Oxycon device (Jaeger), and the electrocardiogram (CardioSys; Marquette-Hellige, Freiburg, Germany) was continuously monitored, in search of arrhythmias and/or repolarization alterations. Blood pressure was measured every 2 min using a manual manometer. Maximal oxygen uptake peak was expressed as a percentage of predicted value.
CMR
All patients with HCM underwent CMR using a Siemens Avanto 3-T CMR machine (Siemens Healthcare, Erlangen, Germany). Cine-mode sequences were acquired in the short-axis, four-chamber, and LV long-axis views. An LGE sequence to identify fibrosis was performed in the three cardiac planes 5 to 10 min after 0.1 mmol/kg gadolinium contrast injection on the basis of CMR society guidelines ; LGE was analyzed qualitatively. LV volume, mass, and function were quantified using customized analysis software by a blinded, single experienced investigator. Diastolic wall-to-volume ratio was measured.
Statistical Analysis
Gaussian distribution of all continuous variables was confirmed with the Kolmogorov-Smirnov test, and values are reported as mean ± SD. Comparisons among the four groups were performed using analyses of variance, followed when appropriate by Bonferroni tests. Comparisons between the two HCM groups were performed using Student’s t test and the χ 2 test. A linear mixed model correcting for group, heart rate, and level of exercise was performed to explain the mechanical dispersion changes induced by exercise.
Receiver operating characteristic (ROC) curves were created, and areas under curves were calculated for the ability of GLS and mechanical dispersion a rest and exercise to identify HCM. A two-tailed P value < .05 was considered to indicate statistical significance.
Inter- and intraobserver variability of GLS and mechanical dispersion were expressed by intraclass correlation coefficients and Bland-Altman plots at rest and during submaximal exercise in three subjects randomly selected from each group ( n = 12) ( Figure 3 ).
Statistical analysis was performed using SPSS version 20.0 (SPSS, Chicago, IL). ROC curve analysis and pairwise comparison were performed using MedCalc version 15.2.2 (MedCalc Software, Ostend, Belgium).
Results
Demographic Parameters and Cardiopulmonary Exercise Test Characteristics of the Four Groups
As specified by the inclusion criteria, the physical training duration was similar in both trained groups (6.3 ± 2.9 hr/wk in athletes with HCM and 7.3 ± 4.3 hr/wk in healthy athletes, P = .880) ( Table 1 ). Sports disciplines included were cycling ( n = 48 [66.7%]), running ( n = 6 [8.3%]), soccer ( n = 12 [16.6%]), tennis ( n = 2 [2.8%]), basketball ( n = 2 [2.8%]), and rugby ( n = 2 [2.8%]). The exercise capacity of athletes with HCM (108.1 ± 21.1% of predicted maximal oxygen uptake peak value) was markedly lower than that of healthy athletes (166.7 ± 24.1%), higher than that of sedentary patients with HCM (74.9 ± 16.5%), and similar to that of sedentary control subjects (110.7 ± 21.6%).
Sedentary patients with HCM ( n = 36) | Athletes with HCM ( n = 36) | Sedentary control subjects ( n = 36) | Healthy athletes ( n = 36) | P | |
---|---|---|---|---|---|
Demographics | |||||
Male/female | 33/3 | 34/2 | 36/0 | 36/0 | .133 |
Age (y) | 40.6 ± 10.2 | 38.1 ± 16.5 | 37.1 ± 16.1 | 41.0 ± 16.7 | .636 |
BSA (m 2 ) | 1.9 ± 0.2 | 1.9 ± 0.2 | 1.9 ± 0.1 | 1.9 ± 0.1 | .467 |
Physical training (hr/wk) | 0 ∗ , ‡ | 6.3 ± 2.9 § | 0 | 7.3 ± 4.3 | <.0001 |
Maximal oxygen uptake peak (% of predicted value) | 74.9 ± 16.5 ∗ , † , ‡ | 108.1 ± 21.1 ‖ | 110.7 ± 21.6 ¶ | 166.7 ± 24.1 | <.0001 |
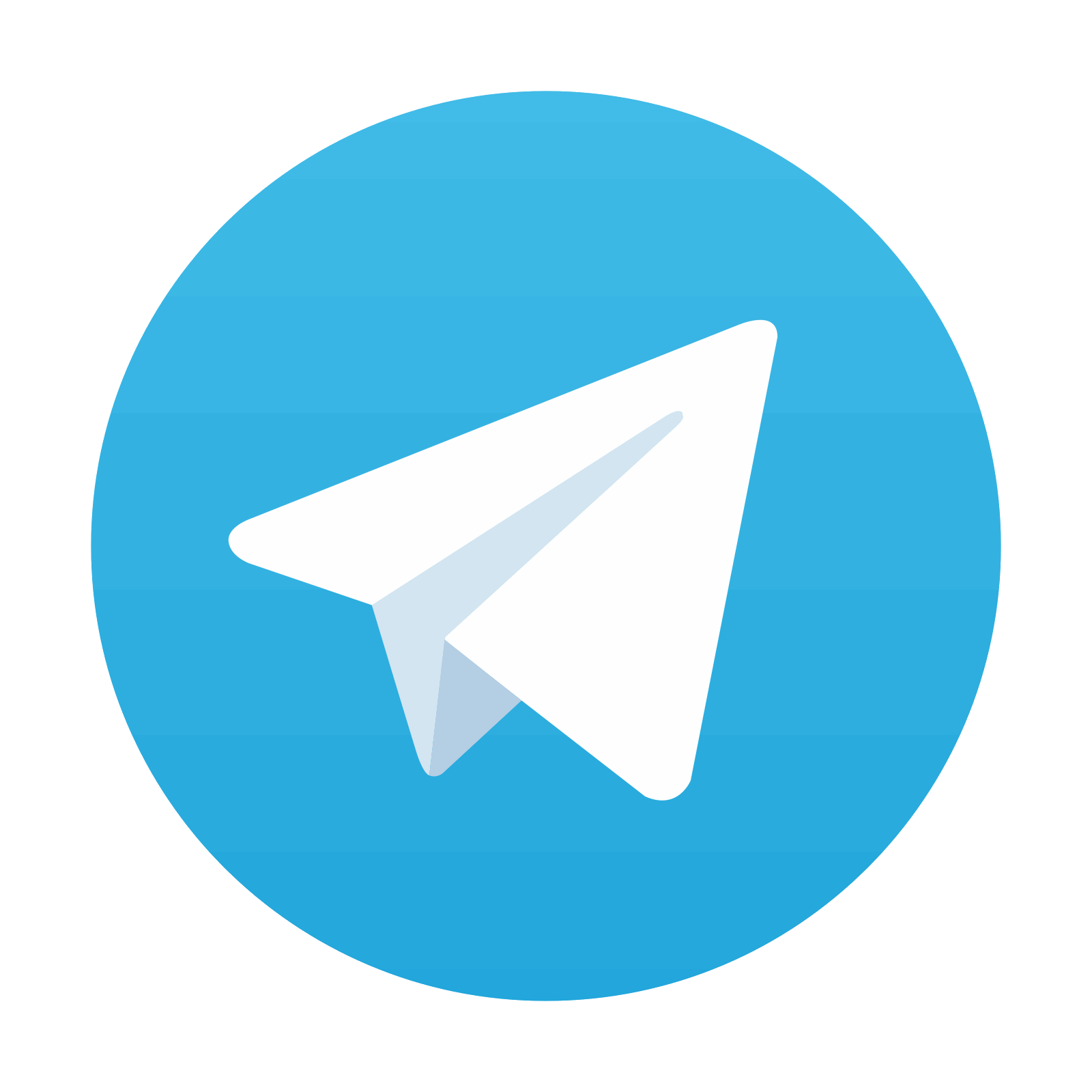
Stay updated, free articles. Join our Telegram channel

Full access? Get Clinical Tree
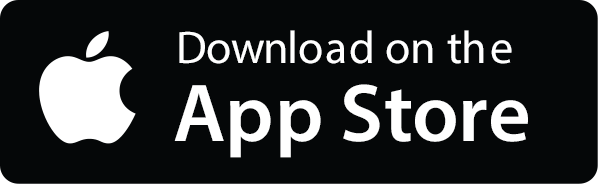
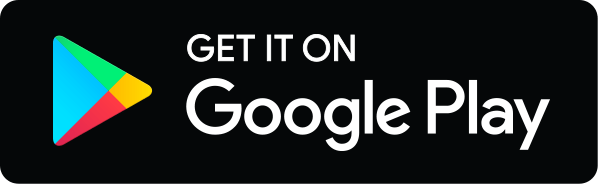
