Fig. 27.1
Generations of left ventricular assist devices. (a) Schematic drawing of the implanted first-generation LVAD HeartMate XVE. (b) Schematic drawing of the implanted second-generation LVAD HeartMate II. (c) Third-generation LVADs. Upper image HeartMate III and lower image HeartWare HVAD. (d) Schematic drawing of the implanted HeartWare HVAD ((a) Adapted with permission from the Wilson et al. [21], © 2009, with permission from Elsevier. (b) and (c) (upper): Adapted with permission from Thoratec incorporation. (c) (lower) and (d): Adapted with permission from HeartWare incorporation)
Table 27.1
Left ventricular assist devices approved by FDA for BTT
LVAD | Manufacturer | Generation | Portable |
---|---|---|---|
Thoratec PVAD | Thoratec | 1st generation | Yes |
Novacor | World Heart | 1st generation | Yes |
HeartMate XVE | Thoratec | 1st generation | Yes |
HeartMate II | Thoratec | 2nd generation | Yes |
HeartWare HVAD | HeartWare | 3rd generation | Yes |
First-Generation LVADs
The first-generation of implantable devices are classified as pulsatile pumps. Examples of this class are the HeartMate XVE® (Thoratec Corp., Pleasanton, CA), Thoratec PVAD™ (Thoratec Corp., Pleasanton, CA), and Novacor N100 (World Heart, Inc., Oakland, CA). First-generation devices had larger tissue and blood contacting surfaces as well as multiple moving parts [21]. Their implantation requires a median sternotomy, with inflow and outflow cannulation insertions made at the left ventricular apex and ascending aorta, respectively. Due to its large size, the pumping chamber is located entirely within the abdomen or preperitoneal space with the transcutaneous driveline exiting the abdominal wall. The major disadvantage of these pumps was their lack of durability, mostly due to failure of the inflow valve after about 18 months of support. Furthermore, high risk of infection, thrombus formation, and blood trauma were significant complications that needed to improve, especially if long-term support was to be achieved with LVAD therapy.
Second-Generation LVADs
The second-generation LVADs are continuous flow (CF) devices that are smaller in size with better efficiency and durability than the first-generation pumps. Examples of the second-generation LVADs include the HeartMate II® (Thoratec Corp., Pleasanton, CA), Jarvik 2000 (Jarvik Heart, Inc., New York, NY), and Micromed DeBakey® (MicroMed Cardiovascular, Inc., Houston, TX). Historically, some questioned whether these CF-LVADs could support long-term end-organ function. It has been well demonstrated that pulsatile blood flow primarily occurs at large arteries as compared to capillary flow, where pulsatile flow is markedly reduced and the average velocity of blood flow in capillaries is about one-thousandth of that of the aorta [22]. Subsequently, large animal studies were able to demonstrate successful long-term end-organ perfusion with CF [23, 24]. The key mechanical difference was the implementation of a valveless axial pump with a rotary motor as the only moving part in the system. This was accomplished by the introduction of an internal rotor in the axial path of flow that was suspended by blood-immersed bearings [25]. The idea behind this design was further reduction of prothrombotic sites and minimization of wear and tear associated with multiple moving parts. Efficiency was further enhanced with elimination of the reservoir chamber and inflow/outflow valves. The blood contacting surfaces were designed with textured titanium as an additional antithrombotic measure.
Its current configuration consists of an inlet cannula placed in the left ventricle, and an outflow cannula which is anastomosed to the aorta via a Dacron graft. A single driveline exits the abdominal wall just below the costal margin. The impeller blade is powered by an electromagnetic motor, which is driven by an external battery source similar to first-generation devices. The second-generation LVADs are designed to provide high-level cardiac output, with rotary speeds of 8000 to a maximum of 15,000 rpm. The benchmark predicted mechanical life of second-generation LVADs is 5 years, but longer support has been well documented. Long-term anticoagulation is still required, with emphasis on individual patient needs as discussed below.
Third-Generation LVADs
The critical difference between the second and third-generation LVADs is that the latter utilizes non-contact bearings to support its impellar which allows for rotation without friction or wear [26]. The goal of this design is to further minimize prothrombotic sites while enhancing efficiency and durability. Examples of the third-generation devices include DuraHeart™ (Terumo Heart, Inc., Ann Arbor, MI), HeartWare HVAD® (HeartWare International, Inc., Framingham MA), Incor® (Berlin Heart, Inc., Berlin, Germany), Levacor® (World Heart Inc., Salt Lake City, UT), and HeartMate III (Thoratec Corp., Pleasanton, CA). Third-generation LVADs are CF pumps that can be broadly differentiated as follows: (1) centrifugal versus axial flow pumps, and (2) magnetically levitated impeller +/− hydrodynamic support [27]. The primary difference between centrifugal flow and axial flow pumps lies in the design of their rotating elements. The rotating element of a centrifugal pump acts as a spinning disk with blades that can be viewed as a ‘thrower’; fluid is captured and then thrown tangentially off the blade tips. In contrast, the rotating element of an axial pump operates like an Archimedes screw and can be viewed as a ‘pusher’. Advantages of centrifugal pumps include lower rotational speeds, higher efficiency, and further enhanced anatomic design. Moazami et al. describes in detail differences in pump mechanics between axial and centrifugal CF pumps and its translation into clinical practice [28]. The Incor is the only third-generation axial pump under active clinical investigation, with the remaining pumps being centrifugal. In terms of levitation, the DuraHeart employs a dual hydrodynamic and magnetic system for levitation compared to the Levacor, which is completely magnetically levitated. Unique to the HeartWare, which was FDA approved for BTT in November 2012, is that implantation is completely intrapericardial due to the smaller pump size, eliminating the need for an abdominal pocket [29]. In terms of survival, reports demonstrate expected success with third-generation devices. One European single-center study reported very promising long-term outcomes in 68 patients implanted with the DuraHeart. Overall survival at 3, 6, 12, and 24 months was 87 %, 81 %, 77 %, and 61 %, respectively [30]. The HeartWare Ventricular Assist Device (HVAD) BTT ADVANCE trial enrolled, in a non-randomized fashion, 140 patients who received the HVAD investigational pump versus 499 patients who received a commercially available pump, most controls had received the HeartMate II LVAD. The study showed that the HVAD was non-inferior to the commercially available pumps. Survival in patients receiving the HVAD was 91 % at 6 months and 84 % at 1 year. The disease specific and global quality-of-life scores improved significantly, adverse event rates remained low and the median 6-min walk distance improved by 128.5 m at 6 months after HVAD implantation [31, 32] which is almost a three-fold greater than the improvement achieved in the cardiac resynchronization therapy (CRT) COMPANION trial that enrolled patients with class IV of NYHA [33]. These outcomes reflect incremental improvement since the initial European BTT trial of the same device [34]. Infection, right heart failure, device replacement, stroke, kidney dysfunction, hemolysis and arrhythmia rates were similar to those reported previously for the HeartMate II [35]. Electromagnetic interference between the HVAD and an implantable defibrillator occurred in two patients and thus evaluation for electromagnetic interference before discharge is recommended. The majority of third-generation devices are either in clinical trials, under development or only available in Europe.
Molecular Changes After LVAD Implantation and Their Correlation to Clinical Recovery
Patients with chronic advanced HF can show near-normalization of nearly all of the structural abnormalities of the myocardium or reverse remodeling after LVAD support due to near-total unloading of the ventricle. However, reverse remodeling does not always equate with clinical recovery. In spite of the reversal of structural changes in most patients after a period of support, only a small percentage of patients have shown significant associated improvement in their myocardial function. This improvement in myocardial function can be significant enough to allow the device to be removed, known as myocardial recovery. The molecular changes occurring after LVAD support, both in patients bridged to transplantation and in patients who have recovered enough myocardial function to have the device removed, have been studied [36]. Reverse remodeling may be attributable to a reversal of the pathological mechanisms that occur in remodeling or the generation of new pathways. A reduction in myocardial cell size occurs after LVAD unloading, which does not necessarily correlate with improved cardiac function [37, 38]. However, some of the changes in both the cardiac myocyte and the matrix after LVAD support have been shown to correlate with myocardial recovery. In the myocyte, increases in the cytoskeletal proteins and improvements in the Ca2+ handling pathway seem to be specifically associated with myocardial recovery [39]. Changes in the matrix are complex, but excessive scarring appears to limit the ability for recovery, and the degree of fibrosis in the myocardium at the time of implantation may predict the ability to recover [40–42]. However, translation of structural recovery to sustainable function permitting device removal remains uncertain. In the series by Dandel and coworkers, 32 nonischemic patients who were weaned demonstrated a survival rate of 78 % at 5 years after device explantation. Clinical heart failure recurred during the first 3 years after weaning in 31.3 %, and two died of heart failure. More recently, the same group has updated their experience and defined parameters likely to be associated with successful long-term survival after LVAD explantation. They entertained that left ventricular ejection fraction >45 % at an end-diastolic diameter of <55 mm carries a predictive value of 87.5 % for 5-year cardiac stability. More meaningful and sustained recovery after LVAD explantation is seen in patients who develop HF in the context of myocarditis, peripartum cardiomyopathy, and post cardiac surgery [43]. Birks and colleagues implanted LVADs in 15 patients with severe HF due to nonischemic cardiomyopathy. The patients were treated with lisinopril, carvedilol, spironolactone, and losartan to enhance reverse remodeling. Once regression of left ventricular enlargement had been achieved, the β2-adrenergic receptor agonist clenbuterol was administered to prevent myocardial atrophy. Of the 15 patients, 11 had sufficient myocardial recovery to undergo explantation of the LVAD at 10 months after implantation of the device. One patient died of intractable arrhythmias 24 h after explantation; another died of carcinoma of the lung 27 months after explantation. The cumulative rate of freedom from recurrent heart failure among the surviving patients was 100 % and 88.9 % at 1 year and 4 years, respectively [44]. This investigation suggested long-term recovery with pharmacologic support; however, the absence of a control group precludes conclusive statements about the precise role of quadruple therapy and particularly raises doubts about the true impact of the agent clenbuterol. These data require further confirmation in randomized controlled trials.
Patient Selection and Clinical Outcomes with LVADS
Critical factors in order to achieve optimal outcomes after implantation of a LVAD include patient’s illness, underlying comorbidity and timing of device implantation. It is very important that surgery take place at the right time when the patient is neither too sick nor too early in the course of the patient’s illness.
The Heart Failure Survival Score and the Seattle Heart Failure Model can be used to estimate a heart failure patient’s expected survival during the next 1 to 2 years with medical management and to identify patients at high risk of death who might benefit from LVAD support. Before consideration for LVAD implantation, patients should be evaluated at specialized centers, where they receive aggressive medical management for advanced heart disease. If they remain refractory to standard therapy, they should be assessed and, if appropriate, listed for cardiac transplantation. Patients who have prolonged waitlist time, especially if they are inotrope dependent, should be considered for LVAD implantation as a BTT as the waitlist mortality for transplant exceeds by far that of LVAD mortality [45]. Other indications for LVAD implantation include individuals who require temporary circulatory support and are expected to recover after a cardiac insult (Bridge to recovery) or those who need long-term support but have a relative or absolute contraindication to cardiac transplantation (DT). About 80–90 % of LVADs are implanted in transplant candidates who are not expected to survive until transplant or who are deemed too sick for transplant or who have potentially reversible transplant contraindications [46]. On the contrary, up to 17 % of DT patients subsequently undergo heart transplantation [47] and many BTT patients subsequently become ineligible for heart transplantation. Despite that recommendations for mechanical circulatory support have been implemented [48], patient selection usually relies on certain criteria such as patient clinical status, inotrope dependence and invasive hemodynamic parameters. It is noteworthy to mention that with worsening clinical status, the need for LVAD increases but so does the peri-operative risk and thus optimal operative timing becomes difficult. Another key consideration for LVAD implantation is the expected waiting time for heart transplantation, which is highly variable between different regions and is dependent on body size, blood type and panel reactive antibodies. The main goals of LVAD therapy are to improve symptoms, quality of life and prognosis. Other goals include stabilization or reversal of organ dysfunction and lowering pulmonary vascular resistance which may then allow subsequent successful heart transplantation. Table 27.2 highlights the indications and contraindications of LVAD implantation.
Table 27.2
Indications and contraindications for LVAD implantation
Indications |
NYHA class IV symptoms and failure to respond to OMT for at least 45 days of the last 60 days |
Chronic inotrope dependence and life expectancy <2 years (more than 50 % 1-year mortality) |
Left ventricular ejection fraction <25 % |
Peak oxygen consumption ≤12 ml/kg/min with significant cardiac limitation |
Refractory cardiogenic shock or failure (SBP ≤80–90 mmHg, PCWP ≥ 20 mmHg, CI ≤ 2.2 L/min/m2) or declining renal or right ventricular function. |
Recurrent sustained ventricular tachycardia in the setting of an untreatable arrhythmogenic substrate |
Body surface area >1.5 m2 |
Contraindications to heart transplant or PVR >5 Woods.unit or GFR <25–30 ml/min/1.73 m2 that is likely to improve after LVAD implantation. |
Relative contraindications |
Age >65, unless minimal or no other risk factors |
Chronic kidney disease with Cr >3 mg/dL |
Severe chronic malnutrition (BMI <21 kg/m2, in males and <19.21 kg/m2 in females) |
Morbid Obesity (BMI >40.21 kg/m2) |
Mechanical ventilation |
Severe mitral stenosis or moderate to severe aortic insufficiency or uncorrectable mitral regurgitation |
Absolute contraindications |
Potentially reversible cause of heart failure or high surgical risk for successful implantation |
Neurological deficits impairing the ability to manage device, or lack of psychosocial support or new evolving stroke |
Severe multiorgan failure, severe pulmonary hypertension and severely reduced RV function |
Active systemic infection or terminal illness (such as metastatic cancer and cirrhosis) |
Inability to tolerate systemic anticoagulation and heparin induced thrombocytopenia |
Impending renal or hepatic failure or severe pulmonary dysfunction (FEV1 <1 L) |
Patient risk factors that are associated with worse outcomes after LVAD implantation or preclude LVAD implantation include deficiencies in nutritional status, hematologic abnormalities, presence of hepatic and renal insufficiency, right ventricular dysfunction, lung disease and neuropsychiatric and psychological considerations [48–50]. A clinical algorithm that takes into account a patient’s risk factors is shown in Fig. 27.2. Various composite risk scores have been devised from hemodynamic parameters and measures of end-organ function to help identify predictors of survival and guide patient selection. Examples of these risk scores are the Columbia University/Cleveland Clinic risk factor selection scale (RFSS) [51], The Columbia University/Cleveland Clinic revised screening scale (RSS) [52], the Lietz-Miller Destination Therapy Risk Score (DTRS) [47] and most recently the HeartMate II risk score (HMRS) [53]. Although useful in clinical decision-making, none of the risk scores, except for the HMRS, have been prospectively validated. They are derived from small selected populations and are limited to specific mechanical devices. The HMRS has been prospectively validated and included 1122 patients who have received HeartMate II as a BTT or DT. The limitation of the HMRS is that clinical trial patient population is not representative of many non-trial patients. Moreover, the applicability of the HMRS to patients undergoing implant of other types of CF devices is not known.


Fig. 27.2
Clinical algorithm to initiate mechanical circulatory support and factors involved in determining outcomes and appropriateness of LVAD implantation. OMT optimal medical therapy, CRT cardiac resynchronization therapy, HTx heart transplant, MS mitral stenosis, AI aortic insufficiency
The INTERMACS registry [54] has defined patient profiles which distinguish various categories of risk among the subset of advanced HF patients. The INTERMACS risk levels, Table 27.3 , range from 1–7 and in general level 1–5 fall under NYHA class IV, with level 1 classifying as the worst state, known as cardiogenic shock or Crash and Burn. Levels 6–7 are patients in the advanced NYHA class III subset and can be viewed as relatively low risk. Patients receiving implantable ventricular assist devices were frequently in profiles 1 and 2 and experienced relatively high perioperative mortality. This lead to the gradual shift towards implanting less sick individuals with INTERMACS profiles level 3–4 as reported in the second INTERMACS report that analyzed 1092 primary LVAD implants including 48 % pulsatile-flow and 52 % CF pumps [55]. An emerging issue in BTT is whether to implant an LVAD before the institution of chronic inotrope support. The vast majority of patients implanted to date have been inotrope dependent. Inotrope dependence is associated with more than 50 % mortality at 6 months [56] and the medical arm in the REMATCH [18] and INTrEPID [19] had 76 % and 89 % mortality at 1-year, respectively. Moreover, earlier implantation, before right ventricular and multiorgan failure, leads to better outcomes. This is a favored strategy for DT. It is also important to realize that poor tolerance of evidence-based pharmacologic therapy, repeat hospitalizations, escalating inotrope or pressor needs and end-organ dysfunction are more integrated criteria for LVAD than single hemodynamic parameters. In general, outcomes are better for stable patients entering an operative procedure than for subjects who are in extremis.
Table 27.3
Interagency Registry for Mechanically Assisted Circulatory Support (INTERMACS) clinical profiles [54]
Level | Definition | Description |
---|---|---|
1 | Critical cardiogenic shock | “Crash and burn”. Patients with cardiogenic shock despite escalating inotropic support resulting in critical organ hypoperfusion. Definitive intervention needed within hours. |
2 | Progressive decline | “Sliding fast”. Patients with clinical status declining despite being on inotropes or are unable to tolerate inotropic therapy. Definitive intervention needed within few days. |
3 | Stable but inotrope dependent | “Dependent stability”. Patients whose clinical status (blood pressure, organ function) is stable with continuous intravenous inotropic support or a temporary support device but are unable to be weaned off. Definitive intervention during a period of weeks to few months. |
4 | Resting symptoms | “Resting symptoms”. Patient experiences daily symptoms at rest and require high doses of diuretics. Definitive intervention elective within a period of weeks to few months. |
5 | Exertion intolerant | “Housebound”. Patient asymptomatic at rest but is unable to engage with any other activity. May consider for LVAD implantation. |
6 | Exertion limited | “Walking wounded”. Patient with no evidence of fluid overload and is comfortable at rest and with activities of daily living but gets fatigued within the first few minutes of any meaningful activity. May consider for LVAD implantation. |
7 | Advanced NYHA III | “Advanced NYHA III”. Patients without evidence of fluid overload who are living comfortably with meaningful activity limited to mild physical exertion. Advanced HF therapies may not currently be indicated. |
The effects of LVAD support as a BTT on heart transplantation outcomes have been conflicting in different trials. In the ISHLT registry, patients with pre-heart transplant LVAD fared worse after heart transplantation [57]. This registry did not account for selection bias, era of implant, patient characteristics and other confounding factors. In fact other studies suggested a neutral [58] or favorable [59] effect. Moreover, it has been shown that the HeartMate II® device reduces wait list mortality and improves post-transplant survival as opposed to inotrope therapy and other mechanical assist device therapy [60]. The duration of support with HeartMate II LVAD did not affect early and long-term post-transplant survival when compared to that of conventional transplantation [61, 62]. However, device infections and the increased need for blood transfusions during LVAD support were associated with significant reduction in 1 year and 10 years post-transplant survival [61, 62]. The frequent need for blood transfusions during LVAD support may provoke HLA sensitization, which can impact heart transplant candidacy. Complications other than those listed above did not seem to have significant impact on post-transplant survival in HeartMate II trials [61].
The REMATCH trial, a multicenter study that compared long-term implantation of LVADs with optimal medical management for patients with refractory heart failure, was conducted to test the hypothesis of destination therapy for patients ineligible for transplantation [18]. This trial demonstrated that compared with best medical therapy device implanted patients had an average extension of life of 8 months, of which 3 months were spent in the hospital. This particular trial demonstrated that when patients were appropriately selected, even patients with a futile outlook could achieve clinically meaningful benefits. However, patients with devices were more than twice as likely to develop an adverse event than the medical therapy group and had a higher median number of days spent in and out of the hospital. Thus, at 2 years, only 23 % in the ventricular assist device group were alive compared with 8 % in the medical group. The probability of infection with the device was 28 %; bleeding, 42 %; and device failure, 35 %, requiring device replacement. This early proof-of-concept trial did not result in an aggressive widespread translation into community application because of concerns of cost, lack of device durability, and complications related to infection and thromboembolism.
To date, the only CF-LVAD approved for both BTT and DT is the HeartMate II. The HMII BTT clinical trial was a prospective, multicenter study without a concurrent control group that included 133 patients with end-stage heart failure who were awaiting heart transplantation. The principal competing outcomes were the number of patients bridged to transplantation; who experienced myocardial recovery or continued to be supported while remaining eligible for transplantation at 180 days after LVAD implantation. The principal outcome occurred in 100 patients and the median duration of support was 126 days. The survival rate was 75 % and 68 % at 6 and 12 months, respectively. The therapy resulted in significant improvement in functional status and in quality of life at 3 months after LVAD implantation. Major adverse events included postoperative bleeding, stroke, right heart failure and percutaneous lead infection. Pump thrombosis occurred in two patients. This study resulted in market approval of the HeartMate II as a BTT by the FDA in April 2008 [63]. The pivotal DT trial with the HeartMate II, is the largest published, randomized clinical trial of DT. The trial evaluated the use of the second-generation CF axial pump, the HeartMate II. The study was conducted between March 2005 and May 2007and enrolled 200 patients with advanced HF not eligible for heart transplant at 38 United States hospitals. The trial entry criteria were similar to that of the REMATCH trial. The study randomized patients in a 2:1 fashion; 134 patients received CF axial flow device, the HeartMate II, and 66 patients received the first-generation pulsatile device HeartMate XVE. The study demonstrated that the HeartMate II had a significantly greater percentage of patients who reached the primary endpoint of survival at 2 years, free of disabling stroke and reoperation for pump replacement, compared with the XVE [64]. Survival rates were 68 % and 58 % at 1 year and 2 years, as compared to 55 % and 24 % for the HeartMate XVE, respectively (Fig. 27.3). These results led to FDA approval of HeartMate II® for destination therapy in January 2010.
Current State of LVAD Utilization and Quality of Life Post LVAD Implantation
The choice of device largely depends on the indication for which it is being used and the anticipated duration of support. The most common indications for the use of LVADs are in patients with heart failure (BTT, DT or as a bridge to candidacy), cardiogenic shock (post-cardiotomy or post-myocardial infarction or myocarditis) or refractory ventricular arrhythmias. According to the latest INTERMACS annual report, 98 % of LVADs being implanted are second-generation [65]. In addition, the indications for device implantation between January 2009 and June 2010 were BTT (41 %), bridge to candidacy (43 %), DT (13.8 %), bridge to recovery (1.0 %), rescue therapy (0.5 %) and other (0.5 %). The area of biggest change over the previous 2 years was the increase of device implantation as DT. This trend can partly be attributed to the approval of the HeartMate II® in January 2010 as a device for destination therapy in patients deemed not eligible for transplantation. Nearly 30 % of patients receiving implantation as BTT were still on mechanical support at 2 years and 43 % of those patients were no longer listed for transplant after 2 years of mechanical support. Another significant finding of the INTERMACS data is the continued improvement in overall survival with LVAD implantation. The latest reported survival of 79 % at 1 year and 66 % at 2 years among all patients receiving LVAD support is quite remarkable given the dismal prognosis with best medical therapy in this group of patients. A subgroup analysis of the data showed that patients receiving CF pumps, especially the HeartMate II LVAD, fared even better, with a survival rate of nearly 80 % at 1 year [65, 66]. DT continues to carry a slightly higher risk than BTT therapy. The survival difference in predicted 1-year survival, when adjusted for risk factor prevalence in each group, is approximately 5 %. The small survival difference is likely related to the ability of transplantation for some BTT patients in the event of device related complications [67]. However, it is noteworthy to mention that the mortality rate in patients with INTERMACS level 1 continues to be high.
Despite potential complications, LVADs significantly improved the quality of life (QOL) and functional capacity in patients with advanced HF. QOL in a diseased state is a multidimensional concept and includes aspects of physical, mental and social functioning. The Minnesota Living With Heart Failure Questionnaire (MLHFQ) and the Kansas City Cardiomyopathy Questionnaire (KCCQ) are reliable and validated health status questionnaires specific to patients living with HF [68, 69]. LVAD patients, when surveyed about lifestyle changes, were able to drive, exercise, travel, return to work or school and engage in hobbies [70]. The improvement in QOL can be seen as early as 1 to 3 months after LVAD implantation and is sustained for the duration of support. This QOL improvement surpasses that achieved with adjunctive pharmacologic or cardiac resynchronization therapy in patients with advanced HF [71, 72]. More recently, in BTT and DT patients, the HeartMate II CF device has improved QOL on multiple validated indexes such as the MLHFQ and KCCQ [18, 63]. Patients with HeartMate II LVAD had significantly higher peak VO2 at 6 months when compared with that at 3 months post LVAD implantation [73]. Additionally, there was significant increase in the distance that the patients could walk between baseline (43 m) and 3 months after HeartMate II implantation (292 m) [63]. These improvements were maintained but did not change significantly for the duration of the follow-up period [35]. The impact of different devices on QOL has not been compared directly in clinical trials to date. It is supposed that QOL may be even higher with the newer devices because of their smaller size, higher efficiency and durability and less associated complications. QOL outcome measures have become an integral part of all clinical trials and registries involving LVADs due to the evolution of the mechanical circulatory support devices.
Another emerging aspect is donor heart allocation in the present LVAD era. According to the United Network of Organ Sharing (UNOS), patients who are critically ill (class IA) who could not receive a heart transplant and instead ended up having a LVAD implant as a bridge to transplant, remained classified as class IA for the next 30 days after LVAD implantation. The rationale behind this is that LVAD supported patients remained at high risk for LVAD failure over time. However, this decision was implemented back when pulsatile flow devices were being incorporated. This prioritization has remained even after the emergence of continuous flow LVADs, which are less likely to fail and have produced higher survival rates. Because of that, there is a perception that stable patients are able to ‘jump the list’ ahead of other more critically ill patients and has raised a concern as to whether the UNOS donor heart allocation system in its present configuration is indeed fair [74]. The question that comes to the surface is whether clinically stable LVAD patients should continue to receive prioritization for donor hearts. Dardas et al. proposed that they should not as this action violates the justice principle. There is no utilitarian reason to prioritize stable LVAD patients because transplant outcomes are not superior in this group compared with other class IA and class IB subgroups [75]. In the Eurotransplant system, there is no prioritization for stable LVAD patients unless they develop a device complication [76]. The solution to this problem would perhaps be by adapting a heart allocation score similar to that for end-stage liver disease. Another possibility is to expand the number of prioritization categories matching individual risk profiles [74].
Optimal Anticoagulation Regimen in Patients with LVAD
Patients with axial-flow LVADs develop substantial alterations in their prothrombotic profile and platelet function, which appears to be reversible after the removal of the device and are likely to be responsible for the non-surgical bleeding episodes [77–79]. Warfarin seems to offer a lower thromboembolic risk compared with unfractionated heparin or low molecular weight heparin [80]. There are reports which suggest that some axial-flow LVAD patients may be managed without anticoagulation, for example after major bleeding complications; however, these papers are subject to publication bias as poor outcomes are unlikely to have been reported [81, 82]. Antiplatelet and anticoagulant therapy must be started early, but only after meticulous control of postoperative bleeding has ensued. The use of warfarin (INR target 2–3), in association with aspirin at 81–325 mg/day, or with point-of-care tests titrated antiplatelet therapy to inhibit 70 %, is one strategy that can be used. Although HeartWare targets a higher INR, the target INR for the newer CF devices is in the range of 1.5 to 2.5, beyond which the risk of thrombosis and bleeding escalates [49, 83]. This is because gastrointestinal bleeding (GIB) is more commonly encountered with the newer CF pumps [84]. Although the exact reason for this occurrence is uncertain, one hypothesis implicates the development of acquired von Willebrand (vWF) factor deficiency caused by increased shear stress and reduced pulsatility of these devices with an increased prevalence of arteriovenous malformations (AVMs). An identical constellation of findings is described in Heyde’s syndrome, namely, aortic stenosis with acquired vWF deficiency and GIB from AVMs. The rate of GIB ranges between 18–30 %. Importantly, these rates are significantly higher than that described in patients receiving Aspirin and Warfarin for mechanical valve prosthesis [85]. Recurrent bleeding after a first event is not uncommon and ranges between 21–44 %. However, available data suggests that GIB is associated with very low mortality (<1 %) [86, 87]. History of GIB, age, INR and platelet count were found as an independent predictors for GIB in a multivariate analysis. The risk/benefit of reducing or discontinuing anticoagulation should be thoroughly assessed and discussed with the patient. Lowering of the device power and speed to decrease shear stress may decrease such bleeding episodes but remains to be proven as a viable treatment option. Higher degree of anticoagulation must be targeted in patients with atrial fibrillation, prior thromboembolic events, presence of atrial or ventricular thrombi and if low assist device flow rates of less than 3 L/min are anticipated. Patients who develop heparin-induced thrombocytopenia after LVAD implantation, which is verified by serotonin release assay [88], are at high risk of thromboembolism and should be treated with a direct thrombin inhibitor. Bivalirudin is the preferred agent as its elimination is enzymatic by thrombin and is not dependent on renal or liver functions, which are frequently impaired in patients at the time of LVAD implantation [88].
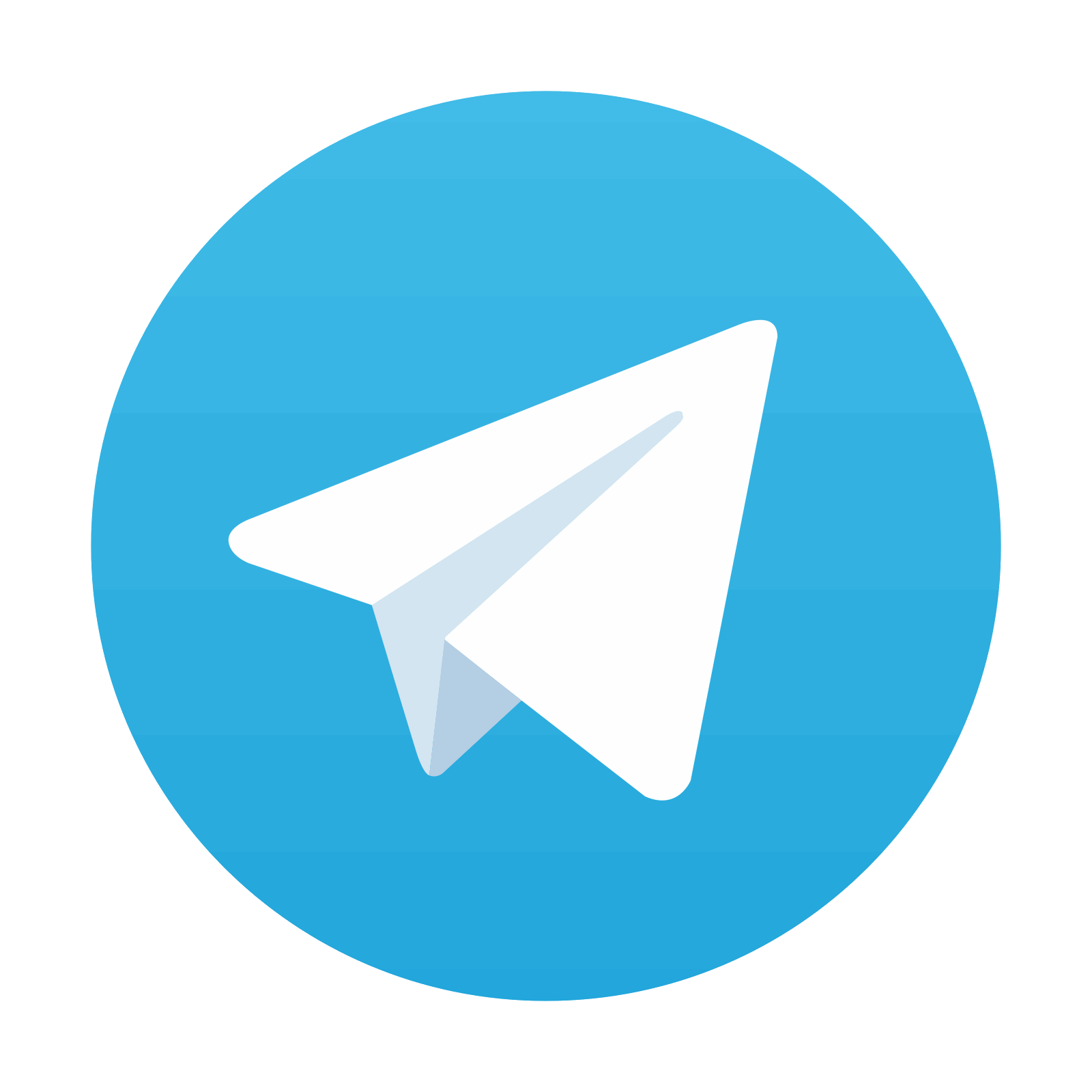
Stay updated, free articles. Join our Telegram channel

Full access? Get Clinical Tree
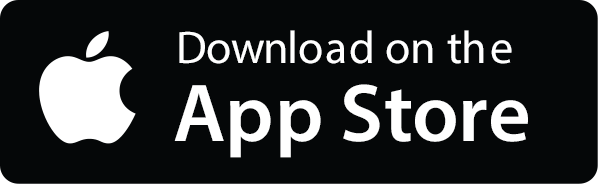
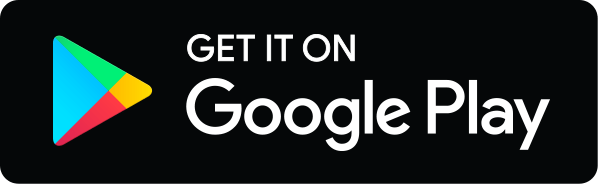