Background
Early prediction of infarct size directs therapy in patients with acute myocardial infarction (AMI). Global strain by echocardiography describes myocardial deformation and correlates with infarct size. However, peak strain measures deformation at a single time point, whereas ischemia and necrosis influence deformation throughout the heart cycle. It was hypothesized that the measurement of myocardial deformation throughout the heart cycle by mean strain is a more comprehensive expression of myocardial deformation. The aim of this study was to assess the ability of mean strain to predict infarct size and to identify large infarctions at admission and after revascularization in patients with AMI.
Methods
Seventy-six patients with AMI were included. Echocardiographic measurements were performed at admission and after revascularization. Myocardial strain was calculated using speckle-tracking echocardiography. Infarct size was measured using contrast-enhanced magnetic resonance imaging ≥3 months after revascularization.
Results
There were significant correlations between infarct size and longitudinal global mean strain, longitudinal global strain, and left ventricular ejection fraction ( P < .0001), both at admission and after revascularization. The correlations improved after revascularization. Longitudinal global mean strain had the best correlation with infarct size and the best ability to discriminate between different infarct size categories. At admission, a cutoff value of −7.6 had 89% sensitivity, 88% specificity, and an area under the receiver operating characteristic curve of 0.92 for the identification of large infarctions. Prediction of infarct size improved for all parameters after revascularization.
Conclusions
Longitudinal global mean strain provides improved early prediction of infarct size in patients with AMI compared with longitudinal global strain and left ventricular ejection fraction.
In patients with acute myocardial infarction (AMI), early determination of left ventricular (LV) function and prediction of infarct size are important for prognostic assessment and decisions on short-term and long-term therapeutic management. Contrast-enhanced magnetic resonance imaging (MRI) has emerged as the reference tool for the assessment of infarct size. However, its clinical application is limited by availability and costs. More accessible and economic methods are therefore required. LV ejection fraction (LVEF) by echocardiography is widely used to determine LV function and may predict moderate and large infarcts. Important advantages with echocardiography are extensive availability, low costs, and the absence of serious side effects. However, LVEF is limited by poor reproducibility and poor agreement with reference methods and is inaccurate in the prediction of small infarcts. Recently, myocardial deformation by strain echocardiography has been introduced to measure global and regional LV function. Global strain appears to have advantages over LVEF in the prediction of infarct size and clinical outcome.
However, strain measurements are challenging. Strain is usually reported as the amplitude of deformation at a single time point, usually as peak systolic strain. Selection of this time point requires careful evaluation of the strain curve, as well as identification of the timing of aortic valve closure, which in some cases may be inaccurate. Furthermore, ischemia and necrosis not only determine peak systolic deformation but influence deformation throughout the heart cycle. Diagnostic information might therefore be lost if the quantification of LV function is limited to peak systolic strain.
We hypothesized that a more comprehensive evaluation of myocardial strain, which includes the entire heart cycle, may provide improved diagnostic information and result in better prediction of infarct size than just measuring peak systolic strain. Therefore, the aim of this study was to assess the ability of global mean strain compared with global peak systolic strain and LVEF to predict the size of the healed infarction as measured by contrast-enhanced MRI, and to identify large myocardial infarctions, at admission and after revascularization.
Methods
Patients
Patients with first-time AMI admitted to a local hospital were evaluated for inclusion shortly after admission. Patients with (1) previous coronary artery bypass graft or valve surgery; (2) left or right bundle branch block with QRS duration > 0.12 sec; (3) severe valvular dysfunction, defined according to the guidelines of the American Society of Echocardiography ; (4) continuous severe arrhythmia; (5) dementia or other serious mental conditions; (6) clinical, biochemical, or electrocardiographic evidence of AMI between discharge and MRI; and (7) contraindications to contrast-enhanced MRI were excluded. All patients were treated according to current guidelines of the European Society of Cardiology. Patients with ST-segment elevation myocardial infarction (STEMI) were treated with primary thrombolysis. Coronary angiography was performed both in patients with STEMI and in those with non–ST-segment elevation myocardial infarction (NSTEMI) during the index hospitalization. Only patients revascularized by percutaneous coronary intervention (PCI) were included in this study. Patients in need of coronary artery bypass graft surgery were transferred to a tertiary center, and we were not able to perform follow-up measurements in these patients, so they were not included in the study. The research protocol was approved by the regional committee for medical research and ethics. All participants gave written informed consent.
Echocardiography
Echocardiographic examinations were performed using a Vivid 7 scanner (GE Vingmed Ultrasound AS, Horten, Norway). Measurements were performed shortly after admission, without delaying medical or invasive treatment, and repeated before discharge. Three consecutive heart cycles from three apical imaging planes (four chamber, two chamber, and long axis) and the papillary muscle short-axis plane were obtained by conventional two-dimensional grayscale echocardiography. Echocardiographic recordings were analyzed by a single observer blinded to patient data, using EchoPAC version 7 (GE Vingmed Ultrasound AS). LVEF was measured using Simpson’s biplane method. Wall motion score was visually assessed in a 16-segment LV model, and wall motion score index (WMSI) was the average of analyzed segments.
Strain was calculated using speckle-tracking echocardiography ( Figure 1 ). Longitudinal strains were measured from the three conventional apical imaging planes. The 18 segments obtained from EchoPAC were converted to a standardized 16-segment LV model by averaging the strain values of corresponding apical segments in the apical long-axis and four-chamber planes. Longitudinal global strain was the average segmental peak systolic strain in the 16-segment model. Peak systolic strain was defined as the highest absolute value of peak negative or peak positive strain during systole. End-systole was manually defined by aortic valve closure in apical long-axis view. Correspondingly, longitudinal global mean strain was the average segmental mean strain in the 16-segment model.

Calculation of mean strain was based on data files generated by EchoPAC, which were imported to Microsoft Office Excel 2007 (Microsoft Corporation, Redmond, WA). Mean strain was the average strain throughout an entire heart cycle. Plus and minus signs were kept during analyses.
Circumferential strains were measured from the papillary muscle short-axis imaging plane. A six-segment LV model was constructed to estimate circumferential global strain and mean strain, by averaging the segmental peak systolic strain values and segmental mean strain values, respectively. We did not calculate radial strain, because of methodologic concerns and because radial strain primarily is a function of longitudinal and circumferential deformation. Postsystolic shortening was calculated on a segmental basis as the difference between peak postsystolic strain and end-systolic strain. Longitudinal global postsystolic shortening was calculated as the average segmental value in the 16-segment model.
Contrast-Enhanced MRI
Contrast-enhanced MRI examinations were performed in all patients ≥3 months after admission, using a 3-T scanner (Philips Achieva 3T; Philips Medical Systems, Best, The Netherlands) or a 1.5-T scanner (Magnetom Sonata, Siemens Medical Systems, Erlangen, Germany; or Intera R 10.3; Philips Medical Systems). Late enhancement images were acquired 10 to 15 min after the intravenous administration of a gadolinium-based contrast agent. Infarct size was reported as a percentage of total LV mass. Analyses were performed blinded to echocardiographic findings, using Segment version 1.8 ( http://segment.heiberg.se ) or View Forum Software (Philips Medical Systems). Large infarction was defined as spatial extent of six or more segments, as recently suggested by Kelle et al.
Statistical Analysis
Data are presented as number (percentage), mean ± SD, or median (interquartile range) as appropriate. The correlations between infarct size and mean strain, global strain, WMSI, and LVEF were analyzed using linear regression (SPSS version 16.0; SPSS, Inc., Chicago, IL). Differences in echocardiographic parameters according to infarct size category were assessed using analysis of variance tests. Post hoc Bonferroni corrections were applied. Paired samples t tests were used to compare strain, mean strain, and postsystolic shortening at admission and after revascularization. Sensitivity and specificity were calculated and receiver operating characteristic (ROC) analyses were used to estimate the area under the curve for the identification of large myocardial infarctions. ROC curves were compared according to the method described by Hanley and McNeil (MedCalc version 10.4; MedCalc Software, Mariakerke, Belgium). ROC analyses were also performed to identify patients with heart failure, defined as LVEF < 40%, after revascularization. Multiple logistic regression (enter method) was performed to assess the potential benefit of combining the echocardiographic parameters. Reproducibility was calculated as intraclass correlations in 10 randomly selected patients. P values < .05 were considered significant.
Results
Patients
The study population consisted of 76 patients, 36 (47%) with STEMI and 40 (53%) with NSTEMI. Characteristics of the patients are given in Table 1 . Echocardiographic measurements were performed a median of 1 hour (interquartile range, 0.5–2 hours) after admission. The mean time from administration of thrombolytic therapy to echocardiographic examination in patients with STEMI was 2 ± 1 hours, and the median time to PCI was 17 hours (interquartile range, 3–34 hours). Patients with NSTEMI underwent PCI a median of 20 hours (interquartile range, 11–28 hours) after admission. Subsequent echocardiographic measurements were performed in all patients a median of 1 day (interquartile range, 1–2 days) after PCI. The mean time to MRI was 10 ± 5 months. The median infarct size was 8% (interquartile range, 3%–15%). In 27 patients (36%), the healed myocardial infarction involved six or more segments.
Variable | Value |
---|---|
Age (y) | 61 ± 11 |
Men/women | 61/15 |
Established coronary artery disease | 11 (15%) |
Diabetes mellitus | 5 (7%) |
Hypertension | 26 (34%) |
Active smoking | 18 (24%) |
Systolic blood pressure at admission (mm Hg) | 140 ± 28 |
Heart rate at admission (beats/min) | 71 ± 14 |
Culprit vessel | |
Left anterior descending artery | 28 (37%) |
Circumflex artery | 20 (26%) |
Right coronary artery | 28 (37%) |
Persistent coronary occlusion on coronary angiography | 22 (29%) |
Medications during follow-up | |
Platelet inhibitors | 76 (100%) |
β-blockers | 76 (100%) |
ACE inhibitors/ARBs | 52 (68%) |
Statins | 75 (99%) |
Correlations Between Mean Strain and Established Echocardiographic Parameters
There were significant correlations between longitudinal global mean strain and longitudinal global strain, both at admission ( Figure 2 ) and after revascularization. There were also significant correlations between longitudinal global mean strain and LVEF (admission, r = 0.66; after revascularization, r = 0.74), longitudinal global mean strain and WMSI (admission, r = 0.70; after revascularization, r = 0.73), circumferential global mean strain and circumferential global strain (admission, r = 0.89; after revascularization, r = 0.88), circumferential global mean strain and LVEF (admission, r = 0.59; after revascularization, r = 0.69), and circumferential global mean strain and WMSI (admission, r = 0.63; after revascularization, r = 0.70).
Prediction of Infarct Size and Identification of Large Infarctions
At admission, there were highly significant correlations between infarct size and longitudinal global mean strain, longitudinal global strain, and LVEF ( Figure 3 A). The correlations increased after revascularization ( Figure 3 B). There were also significant correlations between infarct size and circumferential global mean strain, circumferential global strain, and WMSI (admission, r = 0.65, r = 0.72, and r = 0.62, respectively; after revascularization, r = 0.77, r = 0.86, and r = 0.73, respectively).
At admission, longitudinal global mean strain was more accurate in discriminating between patients who developed none, 1% to 10%, 11% to 20%, and >20% infarct size compared with longitudinal global strain, LVEF, and WMSI ( Figure 4 A). All parameters performed well after revascularization. Circumferential global mean strain and circumferential global strain were also able to separate different infarct categories ( P < .01).
ROC analyses of the ability of echocardiographic parameters to predict large myocardial infarction at admission and after revascularization are given in Table 2 . At admission, longitudinal global mean strain had significantly better ability to predict large infarction compared with longitudinal global strain and LVEF ( Figure 5 ). Areas under the ROC curves increased for all parameters after revascularization ( Table 2 ). LVEF is the established parameter for the measurement of LV function and was entered into a multiple logistic regression model along with longitudinal global mean strain and longitudinal global strain. Longitudinal global mean strain remained the only significant parameter in identification of large infarctions, both at admission and after revascularization. Adding circumferential global mean strain, circumferential global strain, or WMSI did not improve the model.
Variable | AUC | Cutoff | Sensitivity | Specificity |
---|---|---|---|---|
Admission | ||||
Longitudinal global mean strain | 0.92 ∗ † | −7.6 | 89% | 88% |
Longitudinal global strain | 0.83 | −15.7% | 78% | 82% |
LVEF | 0.75 | 50% | 75% | 80% |
WMSI | 0.85 | 1.41 | 74 | 80 |
Circumferential global mean strain | 0.92 ∗ | −7.5 | 93% | 84% |
Circumferential global strain | 0.86 | −16.7% | 78% | 74% |
After revascularization | ||||
Longitudinal global mean strain | 0.98 ∗ | −7.7 | 96% | 90% |
Longitudinal global strain | 0.94 ‡ | −15.6% | 89% | 90% |
LVEF | 0.86 | 51% | 78% | 75% |
WMSI | 0.93 | 1.32 | 82 | 90 |
Circumferential global mean strain | 0.98 ∗ | −6.6 | 93% | 96% |
Circumferential global strain | 0.94 ‡ | −15.7% | 93% | 88% |
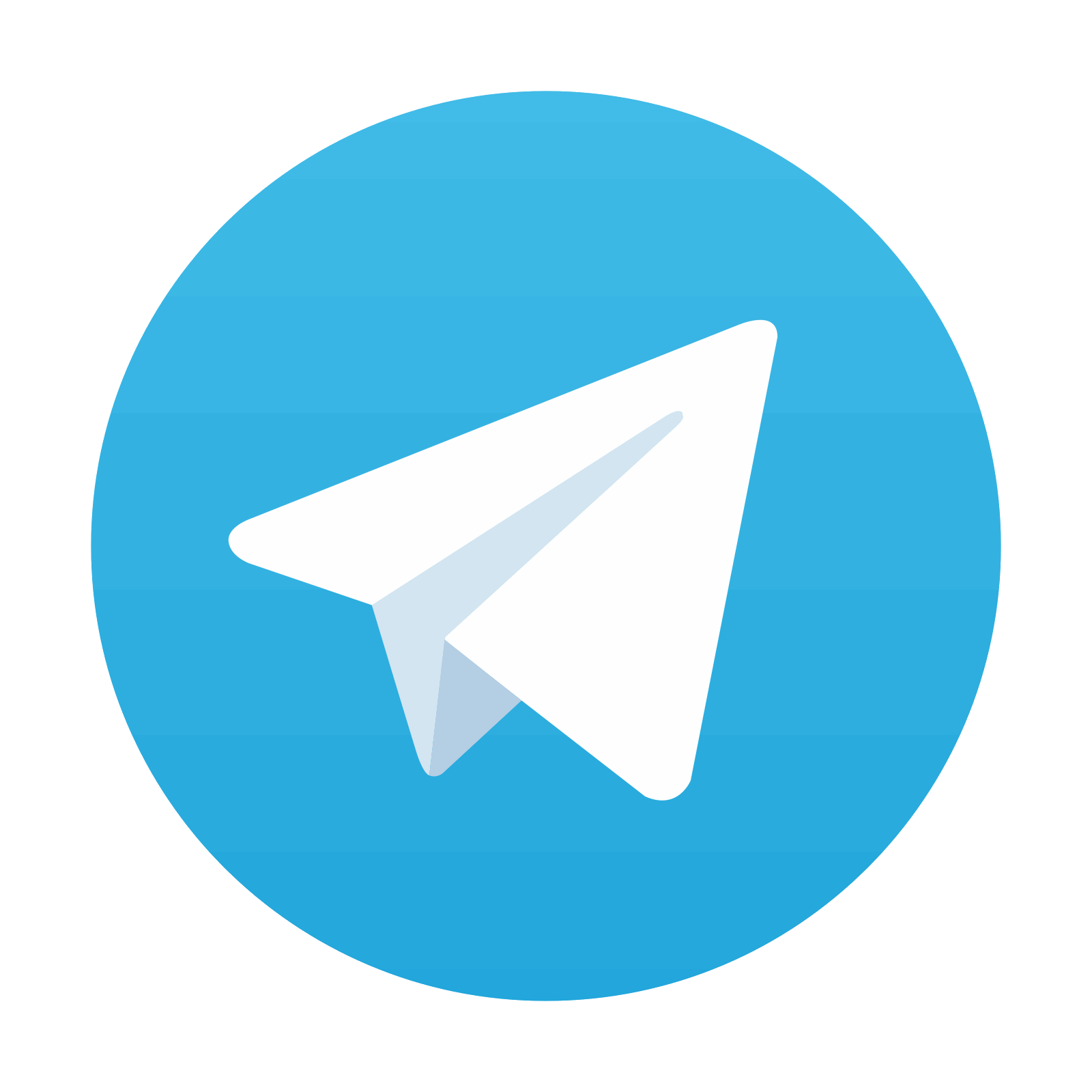
Stay updated, free articles. Join our Telegram channel

Full access? Get Clinical Tree
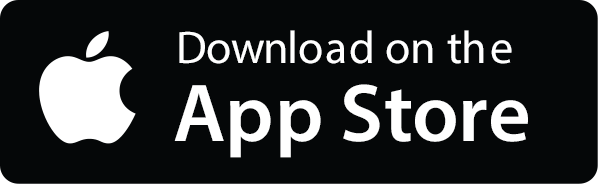
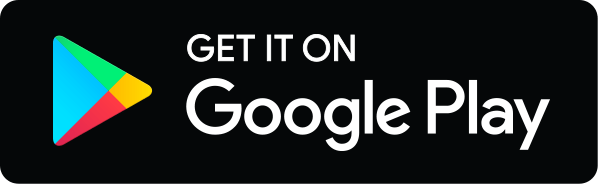
