Background
Doppler tissue imaging (DTI) has been used to evaluate myocardial velocity during ventricular filling, a means of characterizing diastolic function. Previous studies in older children have shown age-related increases in early diastolic tissue velocities, but there are limited data in preterm infants. The aim of this study was to prospectively determine maturational changes in diastolic tissue velocities at two points in time: (1) 7 days of age and (2) 36 weeks’ postmenstrual age (PMA). It was further determined whether DTI measures were altered in infants who developed bronchopulmonary dysplasia with or without pulmonary hypertension.
Methods
A total of 277 preterm infants born at <34 weeks’ PMA, with birth weights between 500 and 1,250 g, were prospectively enrolled. Echocardiograms were obtained at 7 days of age and repeated at 36 weeks’ PMA. Measurements included DTI assessment of early (E′) and late (A′) annular velocities of the left ventricular free wall, septum and the right ventricular free wall. Statistical analysis included the Wilcoxon rank sum test, simple linear regression, and the χ 2 test.
Results
At 7 days of age, there was a statistically significant increase in the E′/A′ ratio as a function of gestational age at birth. At 36 weeks’ PMA, E′/A′ ratio was increased, but there was no association with gestational age. DTI measures were not different between infants who did or did not develop bronchopulmonary dysplasia or pulmonary hypertension at either time point.
Conclusions
A gestational age–related increase was found in the early diastolic tissue velocities of preterm infants. At a gestational age equivalent to near term, no difference was observed in diastolic tissue velocities, regardless of gestational age at birth. These findings suggest that maturational changes in diastolic function occur relatively independently of the timing of birth.
Highlights
- •
Tissue Doppler was used to assess diastolic function in preterm infants.
- •
An age-related increase in early diastolic tissue velocity was found.
- •
At a gestational age equivalent to near term, tissue velocities that compare favorably with those in other term infants were observed.
- •
Tissue Doppler measurements were not different between infants who did or did not develop bronchopulmonary dysplasia or pulmonary hypertension.
Doppler tissue imaging (DTI) has been used extensively in adults to evaluate myocardial velocity as a means of characterizing diastolic function. This technique has also been applied to neonates and children, leading to the observation that myocardial tissue velocities change with somatic growth. In infants, these changes appear to be closely related to the developmental maturation that occurs during the adaptation from fetal to ex utero life.
Early diastolic filling coincides with longitudinal motion of the atrioventricular valve annuli toward the base of the heart, which is characterized by a distinguishable deflection (E′) in DTI spectral Doppler patterns, followed by late diastolic filling that results from atrial contraction (A′). The E′/A′ ratio is a useful indicator of the degree to which ventricular filling depends on atrial systole, or rather how easily blood fills the ventricle as a function of properties of the ventricle itself, relative to filling related to atrial contraction.
The ovine fetal myocardium is characterized by a larger noncontractile mass, as only 30% of the fetal myocardium contains contractile mass in comparison with 60% in the adult. Others have postulated that this structural difference results in lower ventricular compliance and higher end-diastolic pressure, suggesting suboptimal diastolic function. Postnatally, both right and left ventricles remodel to reflect changes in afterload ; during the normal transition, the right ventricle undergoes a reduction in mass related to a decrease in pulmonary vascular resistance, and the left ventricle increases wall thickness in response to its new role as the lone systemic ventricle.
The interruption of normal fetal cardiopulmonary developmental by preterm birth has poorly understood consequences with regard to diastolic function. Small studies in preterm infants have shown that early diastolic tissue velocities are lower than in term infants ; however, this finding has not been validated in a large, prospective cohort, and the developmental changes in diastolic tissue velocities are poorly understood. Similarly, the prognostic value of DTI measurements with regard to the development of pulmonary hypertension (PH) and bronchopulmonary dysplasia (BPD) has not been explored in a systematic and prospective fashion. The impact of a patent ductus arteriosus (PDA) on tissue velocities in preterm infants has been evaluated in small cohorts and appears to decrease diastolic tissue velocity relative to infants with a hemodynamically insignificant ducts.
The aim of this study was to describe the impact of gestational age (GA) on diastolic tissue velocities in preterm infants at two points in time: (1) 7 days of age and (2) 36 weeks’ postmenstrual age (PMA). Associations between DTI measurements and the development of PH and BPD were evaluated, along with the impact of a PDA. We hypothesized that diastolic myocardial tissue velocity in preterm infants would increase with GA as a function of structural changes of the myocardium that are inherent to the normal developmental pattern seen in neonates. We also hypothesized that this developmental pattern is altered in diseases such as BPD and PH, as reflected by differences in DTI measurements.
Methods
All data were obtained as part of a prospective observational research protocol performed at hospitals affiliated with two academic institutions (the University of Colorado Denver and Indiana University), which took place between July 2006 and March 2012. The protocol was approved by the institutional review board of each of the participating sites. Written informed consent was received from the parents or guardians of all participants. Assent was not obtained, because all participants were infants.
Criteria for enrollment included GA ≤ 34 weeks at birth and a birth weight of 500 to 1,250 g, and subjects were required to be enrolled before 7 days of age. “GA” refers to the period before birth, and “PMA” refers to pre- and postnatal age. Exclusion criteria included clinical evidence of congenital heart disease (except PDA, atrial septal defect < 1 cm, and ventricular septal defect < 2 mm if known before enrollment), lethal congenital abnormality, and futile cases (anticipated death before hospital discharge). Infants who died, withdrew, or were transferred before undergoing echocardiographic assessment at 36 weeks’ PMA were excluded from the analysis ( n = 39).
BPD status and severity were assessed at 36 weeks’ PMA using a modification of the National Institutes of Health workshop definition, with application of the oxygen reduction test as described by Walsh et al . Infants were assigned to a BPD status according to the following criteria: neonates on positive pressure support or receiving ≥30% supplemental oxygen (≥35% at Denver’s altitude) were assigned the outcome “severe BPD.” Patients who received <28 days of oxygen and were on room air at 36 weeks’ PMA were assigned to the outcome “no BPD.” Patients receiving >28 days of supplemental oxygen who were on room air at 36 weeks’ PMA were assigned to the outcome “mild BPD.” Those receiving <30% supplemental oxygen (<35% at Denver’s altitude) at 36 weeks’ PMA underwent oxygen reduction testing to differentiate among no BPD (required <28 days of oxygen and passed the oxygen reduction test), mild BPD (required >28 days of oxygen and passed the oxygen reduction test), and moderate BPD (required >28 days of oxygen and did not pass the oxygen reduction test). Disease severity categories were adjusted for altitude (applied to the result of the oxygen reduction test for those who performed the test) for subjects enrolled in Denver (1,600 m). All data were prospectively collected and managed using the Research Electronic Data Capture database hosted at the University of Colorado Denver Development and Informatics Service Center.
Echocardiographic Screening
Research echocardiograms were obtained at 7 days of age and at 36 weeks’ PMA. Echocardiograms obtained in the first days of life have the limitation of coinciding with rapidly changing hemodynamics during the transition from fetal to ex utero life, so initial studies were performed at 1 week of age. Echocardiography was performed using a Vivid 7 (GE Healthcare, Milwaukee, WI) or an iE33 (Philips Medical Systems, Andover, MA) platform, with probe frequencies appropriate for body habitus. Echocardiographic images were reviewed offline, using commercially available software (Cardiovascular Review Station version 2.14.03; AGFA Healthcare, Mortsel, Belgium). Measurements included tricuspid regurgitation jet velocity, measurable dimensions of heart chambers, any detectable shunt lesions, and the direction of flow for any shunt lesion. Shunt lesions were defined as any cardiac-level shunt (patent foramen ovale, atrial septal defect, or ventricular septal defect) or PDA. A PDA equal in diameter or larger than the pulmonary artery (PA) was deemed large. Differentiating between moderate and small PDAs involved subjective assessment of the two-dimensional appearance of the PDA, the appearance of the shunt vena contracta by color Doppler, and the size of the left heart from multiple views. Systemic systolic blood pressure was recorded at the time of echocardiography using a blood pressure cuff unless the patient had an existing arterial catheter. The myocardial performance index (MPI) was obtained by summing the ventricular isovolumic contraction time and relaxation time and dividing by right ventricular (RV) ejection time. Peak early diastolic tissue velocity (E′) and late diastolic tissue velocity (A′) were measured at the atrioventricular valve annular level from the apical window.
Qualitative echocardiographic measures of PH, such as right atrial enlargement, RV dilation, RV hypertrophy, ventricular septal wall flattening, and PA dilation, were noted. Measurements of tricuspid regurgitation jet velocity had to meet predefined quality criteria to be used to estimate RV systolic pressure (RVSP). The criteria required the spectral Doppler pattern to consist of a clearly defined envelope with a discernable peak velocity; incomplete or poorly demarcated envelopes were not used to estimate RVSP. Estimates of RVSP were calculated with no allowance for right atrial pressure, by using the modified Bernoulli equation ([tricuspid regurgitation jet velocity] 2 × 4).
All echocardiograms were interpreted by a single cardiologist (A.K.Y.) blinded to subjects’ clinical status to provide consistent evaluation of findings. Criteria for PH were met by any of the following findings: estimated RVSP > 40 mm Hg, RVSP/systolic blood pressure > 0.5, any cardiac shunt with bidirectional or right-to-left flow, or any degree of ventricular septal wall flattening. To determine whether DTI measures of myocardial tissue velocity were associated with the development of PH or BPD, we compared measurements between infants who were or were not subsequently diagnosed with PH or BPD. Similarly, subjects were grouped by the presence of a moderate to large PDA to determine the impact of a PDA on diastolic tissue velocities.
All research echocardiograms were also separately interpreted by each institution’s clinical cardiologists to provide real-time results to the caring physicians as required by the institutional review boards. However, these interpretations were not used in the analyses. Management decisions based on the echocardiographic results, including the use of vasodilators and the timing of additional clinical echocardiographic examinations, were at the discretion of the caring physicians.
Statistical Analysis
Continuous data are presented as mean ± SD or as median (interquartile range) and categorical data are as proportions (percentages). Distributions for all continuous variables were assessed using the Shapiro-Wilk test for normality. All outcome variables with skewed distributions were analyzed in simple comparisons using Wilcoxon rank sum tests. Simple linear regression fit DTI outcomes by GA at birth and tested whether the slopes were significantly different from zero. Chi-square tests were used to assess the associations between categorical variables. Statistical significance was assessed using an α level of 0.05. All statistics were computed using SAS version 9.4 (SAS Institute Inc, Cary, NC).
Results
Two-hundred seventy-seven subjects were enrolled and survived to undergo 36-week PMA echocardiography. All but three of the 277 infants underwent echocardiography at 7 days of age. Baseline characteristics are summarized in Table 1 . The median GA of the cohort was 27 weeks (interquartile range, 25–28 weeks), with a median birth weight of 909 g (interquartile range, 750–1,075 g), and there was no gender predominance. Two hundred seventeen of the subjects (78.3%) received antenatal corticosteroids.
Variable | Value |
---|---|
Birth weight (g) | 909 (750 to 1,075) |
Birth weight Z score | −0.27 (−0.82 to 0.29) |
Birth weight stratum (g) | |
500–749 ( n = 67) | 660 (600 to 695) |
750–999 ( n = 116) | 870 (810 to 940) |
1,000–1,250 ( n = 94) | 1,129 (1,070 to 1,190) |
GA at birth (wk) | 27 (25 to 28) |
23–26 | 129 (46.6%) |
27–29 | 114 (41.2%) |
30–33 | 34 (12.3%) |
Gender (male) | 135 (48.7%) |
Small for GA | 29 (10.5%) |
Maternal smoking | 38 (13.7%) |
Antenatal corticosteroids | 217 (78.3%) |
Cesarean section | 210 (75.8%) |
Pneumonia | 35 (12.6%) |
Necrotizing enterocolitis | 45 (16.3%) |
Sepsis | 59 (21.3%) |
PDA: indomethacin treatment | 118 (42.6%) |
PDA: surgical ligation | 44 (15.9%) |
Days of CPAP | 12 (6 to 25) |
Required CPAP at 36 wk | 15 (5.4%) |
Days of MV | 16 (5 to 41) |
Required MV at 36 wk | 20 (7.2%) |
Length of stay (NICU) | 90 (74 to 114) |
Discharged on oxygen | 166 (59.9%) |
Anatomic, volumetric, and functional echocardiographic data at 7 days of age and at 36 weeks’ PMA appear in Table 2 . As expected, PDA was more common in the early preterm infants, becoming less frequent in the cohorts at more advanced GA. The incidence of other shunt lesions did not change significantly. Left ventricular (LV) diameter and mass increased, while ejection fraction and shortening fraction decreased, remaining within normal limits. LV MPI was not different, but RV MPI was progressively lower in the cohorts at more advanced GA. Data obtained at 36 weeks’ PMA, regardless of GA at birth, showed no difference in any of the aforementioned parameters, apart from RV end-diastolic dimension; there was a small but statistically significant decrease in RV diameter when the early and late preterm cohorts were compared.
Variable | n | PMA at birth (wk) | P ∗ | ||||
---|---|---|---|---|---|---|---|
23–26 ( n = 126) | n | 27–29 ( n = 114) | n | 30–33 ( n = 34) | |||
7 days of age | |||||||
PDA | 110 | 55 (43.7%) | 93 | 35 (30.7%) | 28 | 4 (11.8%) | .002 |
ASD/PFO | 108 | 108 (85.7%) | 89 | 86 (75.4%) | 27 | 27 (79.4%) | .158 |
VSD | 108 | 6 (4.8%) | 94 | 1 (0.9%) | 27 | 1 (2.9%) | .199 |
RVDd | 104 | 0.54 (0.44–0.64) | 91 | 0.59 (0.47–0.70) | 25 | 0.61 (0.49–0.73) | .05 |
LVDd | 107 | 1.12 (1.04–1.29) | 93 | 1.23 (1.12–1.38) | 29 | 1.35 (1.26–1.41) | <.0001 |
LVEF (%) | 106 | 69.9 (65.5–76.6) | 91 | 66.4 (60.3–73.1) | 29 | 63.3 (60.5–69.0) | .0006 |
FS (%) | 107 | 36.8 (33.4–42.2) | 92 | 34.5 (30.2–39.4) | 29 | 31.6 (29.7–36.0) | .0005 |
LV mass (g) | 106 | 1.71 (1.41–2.23) | 92 | 2.20 (1.82–2.60) | 29 | 2.36 (2.14–2.66) | <.0001 |
LV MPI | 113 | 0.33 (0.28–0.44) | 107 | 0.38 (0.29–0.47) | 31 | 0.37 (0.29–0.47) | .416 |
RV MPI | 112 | 0.30 (0.22–0.42) | 98 | 0.26 (0.17–0.39) | 29 | 0.17 (0.05–0.31) | .0007 |
TR jet peak velocity | 14 | 2.52 (2.42–2.61) | 15 | 2.41 (1.95–2.91) | 1 | 2.19 (2.19–2.19) | .56 |
RV pressure estimate | 13 | 26 (24.4–27.2) | 14 | 24.1 (17.3–33.8) | 1 | 19.8 (19.8–19.8) | .449 |
AT/ET | 111 | 0.38 (0.32–0.47) | 99 | 0.39 (0.32–0.45) | 31 | 0.39 (0.36–0.47) | .377 |
Mitral valve E | 90 | 0.44 (0.38–0.53) | 91 | 0.48 (0.40–0.54) | 24 | 0.48 (0.41–0.57) | .329 |
Tricuspid valve E | 89 | 0.38 (0.33–0.47) | 90 | 0.42 (0.36–0.50) | 23 | 0.39 (0.33–0.47) | .116 |
36 weeks’ PMA | |||||||
PDA | 129 | 20 (15.5%) | 114 | 12 (10.5%) | 34 | 2 (5.9%) | .30 |
ASD/PFO | 129 | 100 (77.5%) | 114 | 87 (76.3%) | 34 | 28 (82.4%) | .70 |
VSD | 129 | 4 (3.1%) | 114 | 2 (1.8%) | 34 | 1 (2.9%) | .70 |
RVDd | 114 | 0.84 (0.64–1.04) | 101 | 0.81 (0.66–0.98) | 30 | 0.71 (0.58–0.83) | .03 |
LVDd | 115 | 1.77 (1.59–1.91) | 106 | 1.72 (1.62–1.87) | 31 | 1.72 (1.53–1.84) | .60 |
LVEF (%) | 114 | 68 (63.3–73.0) | 105 | 66.5 (62.4–71.8) | 31 | 64.6 (62.6–69.3) | .10 |
FS (%) | 115 | 35.3 (31.7–39.2) | 106 | 33.9 (31.0–38.2) | 31 | 32.6 (31.2–36.2) | .08 |
LV mass (g) | 114 | 5.36 (4.33–6.91) | 104 | 5.77 (4.54–7.02) | 31 | 4.72 (4.14–5.85) | .10 |
LV MPI | 116 | 0.35 (0.28–0.44) | 108 | 0.33 (0.27–0.41) | 31 | 0.32 (0.19–0.41) | .08 |
RV MPI | 111 | 0.25 (0.18–0.35) | 101 | 0.25 (0.17–0.35) | 29 | 0.20 (0.11–0.30) | .09 |
TR jet peak velocity | 13 | 2.84 (2.71–3.00) | 4 | 2.76 (2.47–3.05) | 1 | 2.43 (2.43–2.43) | .30 |
RV pressure estimate | 14 | 31.9 (27.0–36.0) | 4 | 30.5 (24.4–37.1) | 2 | 12.06 (0.50–23.62) | .20 |
AT/ET | 115 | 0.31 (0.25–0.39) | 104 | 0.33 (0.27–0.43) | 31 | 0.76 (0.64–1.02) | .20 |
Mitral valve E | 65 | 0.86 (0.71–0.93) | 67 | 0.80 (0.68–0.92) | 19 | 0.61 (0.50–0.72) | .50 |
Tricuspid valve E | 59 | 0.65 (0.56–0.74) | 66 | 0.62 (0.54–0.70) | 20 | .60 |
∗ P values were obtained using nonparametric Wilcoxon signed rank tests for paired data.
Comparing data obtained at 7 days of age and 36 weeks’ PMA (not presented in tabular form), LV MPI was significantly lower when measured at 36 weeks’ PMA compared with 7 days of age ( P = .006). RV MPI was lower, but not statistically different ( P = .06). The ratio of RV outflow acceleration time to ejection time was significantly lower at 36 weeks’ PMA ( P < .0001). Both mitral and tricuspid valve inflow peak velocities were significantly increased at 36 weeks’ PMA ( P < .0001 for both tests).
DTI measurements of LV lateral wall, septal, and RV free wall velocities at 7 days of age and at 36 weeks’ PMA are presented in Table 3 . The subjects are grouped by PMA at delivery for the purposes of illustrating the evolution of diastolic myocardial tissue velocity during postnatal development. As shown in Table 3 , when studied at 7 days of age, there was a statistically significant increase in LV lateral wall diastolic tissue velocity (E′) as a function of increasing GA. LV lateral wall A′ decreased with GA, and as such, the lateral wall E′/A′ ratio increased significantly with GA. Median septal and RV free wall E′ and A′ increased with GA but were not statistically different. The septal E′/A′ ratio was found to be significantly different between the two time points. RV free wall E′/A′ increased, without achieving statistical significance. The ratio of mitral inflow peak velocity (E) to peak early diastolic tissue velocity (E′) decreased significantly related to GA. Table 3 also shows data collected at 36 weeks’ PMA, also grouped by GA at birth. The LV and RV free wall diastolic myocardial velocities were not different by the time infants reached 36 weeks’ PMA, regardless of GA at birth.
Variable | n | PMA at birth(wk) | P ∗ | ||||
---|---|---|---|---|---|---|---|
23–26 ( n = 126) | n | 27–29 ( n = 114) | n | 30–33 ( n = 34) | |||
7 days of age | |||||||
PDA | 110 | 55 (43.7%) | 93 | 35 (30.7%) | 28 | 4 (11.8%) | .002 |
LV lateral wall – E′ (cm/sec) | 93 | 4 (4–5) | 81 | 5 (4–7) | 21 | 5 (5–6) | .003 |
LV lateral wall – A′ (cm/sec) | 85 | 7 (6–8) | 80 | 6 (5–8) | 21 | 5 (4–7) | .019 |
LV lateral wall E′/A′ | 85 | 0.60 (0.50–0.80) | 80 | 0.75 (0.60–1.00) | 21 | 1.00 (0.67–1.20) | <.0001 |
Septum – E′ (cm/sec) | 97 | 4 (3–5) | 86 | 4 (4–5) | 23 | 4 (3–5) | .065 |
Septum – A′ (cm/sec) | 88 | 6 (5–7) | 85 | 6 (5–7) | 22 | 6 (5–7) | .679 |
Septum E′/A′ | 88 | 0.63 (0.50–0.75) | 85 | 0.71 (0.60–0.83) | 22 | 0.67 (0.60–0.80) | .037 |
RV free wall – E′ (cm/sec) | 88 | 5 (4–6) | 76 | 6 (5–7) | 22 | 5.5 (5–7) | .445 |
RV free wall – A′ (cm/sec) | 81 | 9 (8–10) | 75 | 9 (8–10) | 21 | 8 (7–9) | .158 |
RV free wall E′/A′ | 81 | 0.63 (0.50–0.70) | 75 | 0.64 (0.56–0.78) | 21 | 0.67 (0.63–0.86) | .055 |
MV E/E′ | 81 | 10.67 (8.17–13.00) | 81 | 9.14 (6.33–11.75) | 19 | 9.20 (6.60–11.60) | .026 |
TV E/E′ | 76 | 0.07 (0.06–0.09) | 70 | 0.07 (0.06–0.09) | 16 | 0.07 (0.06–0.08) | .913 |
36 weeks’ PMA | |||||||
PDA | 129 | 20 (15.5%) | 114 | 12 (10.5%) | 34 | 2 (5.9%) | .268 |
LV lateral Wall – E′ (cm/sec) | 83 | 7 (6–9) | 67 | 7 (6–9) | 23 | 8 (6–10) | .589 |
LV lateral wall – A′ (cm/sec) | 76 | 8 (6–10) | 66 | 9 (7–11) | 23 | 7 (6–9) | .113 |
LV lateral wall E′/A′ | 75 | 0.82 (0.64–1.43) | 66 | 0.76 (0.63–1.13) | 23 | 0.89 (0.75–1.43) | .169 |
Septum – E′ (cm/sec) | 82 | 6 (5–7) | 68 | 6 (5–7) | 21 | 6 (6–7) | .358 |
Septum – A′ (cm/sec) | 75 | 8 (6–9) | 67 | 8 (7–10) | 21 | 7 (6–7) | .009 |
Septum E′/A′ | 75 | 0.71 (0.60–0.88) | 67 | 0.71 (0.57–0.86) | 21 | 0.86 (0.67–1.00) | .031 |
RV free wall – E′ (cm/sec) | 66 | 9 (7–11) | 57 | 9 (7–11) | 13 | 7 (5–8) | .177 |
RV free wall – A′ (cm/sec) | 61 | 11 (9–13) | 56 | 11 (9–13) | 13 | 9 (8–11) | .07 |
RV free wall E′/A′ | 61 | 0.78 (0.62–0.91) | 56 | 0.79 (0.61–1.15) | 13 | 0.80 (0.64–1.20) | .674 |
MV E/E′ | 60 | 12.07 (9.00–14.10) | 55 | 11.17 (9.29–14.60) | 15 | 8.11 (5.83–12.67) | .092 |
TV E/E′ | 41 | 0.06 (0.05–0.09) | 44 | 0.07 (0.05–0.09) | 10 | 0.08 (0.06–0.13) | .334 |
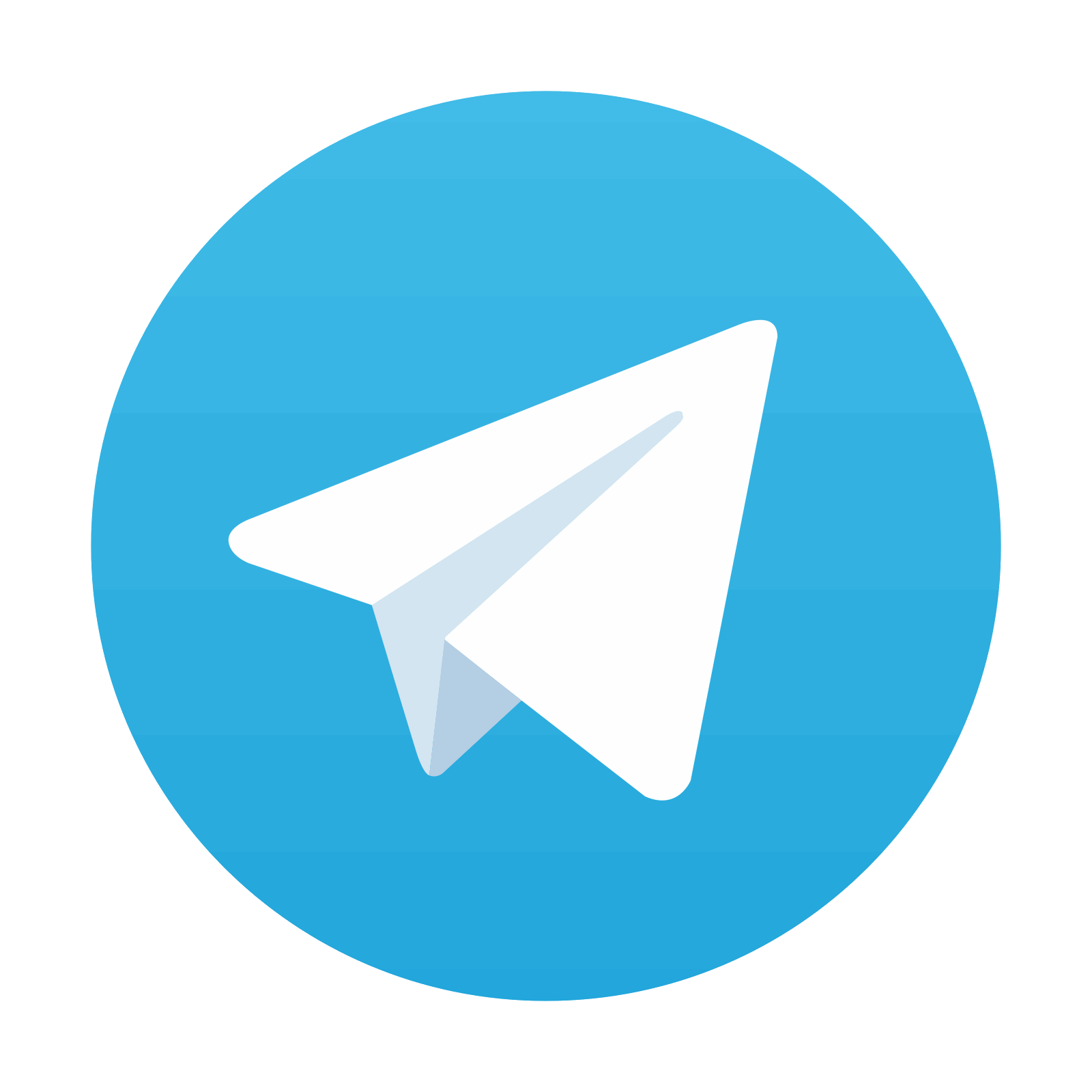
Stay updated, free articles. Join our Telegram channel

Full access? Get Clinical Tree
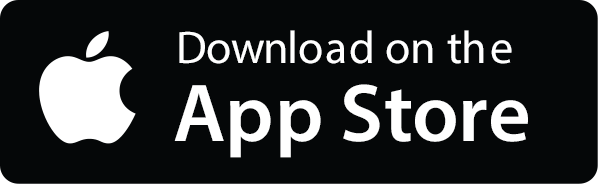
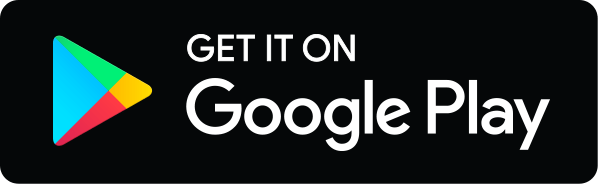
