2 Material The unit “gauge” for cannulas (referring to the outer diameter) corresponds to the number of processing steps required to produce wire of the same diameter. Thus thick cannulas have low gauge numbers and thin cannulas high ones. The diameter of guidewires is specified in thousandths of an inch (1 in. = 2.54 cm). Sizes of catheters and sheaths are specified using the French scale (one French unit = 0.33 mm in diameter). Note: The catheter size refers to the outer diameter, whereas the sheath size refers to the inner diameter: A 5 French catheter fits into a 5 French sheath, which in turn has an outer diameter of ~7 French, depending of course on the material. This occasionally causes some confusion, such as when the recommended material for an intervention includes a 6 French guiding sheath or, alternatively, an 8 French guiding catheter. Vascular access to an artery or a deep vein is best established with a simple open 18 gauge cannula measuring 7 or 9 cm in length (Fig. 2.1). A 19 gauge, thin-walled cannula is sufficient for 0.035 in. wires. The cannula must be sharp; a blunt cannula compresses the vessel instead of passing through the proximal wall into the lumen. For a long time three-piece Seldinger cannulas were used. A double mandrin sealed the lumen of the cannula during the puncture. Because no blood escaped, it was impossible to determine when the tip of the needle had entered the vascular lumen. Often the needle was advanced through the distal wall of the vessel as well. This was nearly unavoidable because the cannulas were almost invariably blunt; they were reused time and again and only occasionally resharpened. Finally the double mandrin was removed and the cannula withdrawn until blood flowed. The puncture of the distal wall that usually occurred was both unnecessary and harmful. Thankfully, this puncture technique has largely fallen from favor. Some physicians use cannulas with plastic sheathing. This puncture technique is really only suitable for superficial vessels that can be punctured at a very acute angle. In deeper vessels it is often necessary to shift the cannula to a more acute angle once it enters the vascular lumen. This makes it easier to advance the guidewire into the vessel. Naturally this cannot be done with a plastic cannula. In small superficial arteries (brachial and radial arteries), one may prefer not to use the comparatively large 18 or 19 gauge cannulas. Alternatively, one may make the puncture with a small cannula (21 gauge = 0.8 mm outer diameter, preferably only 4 cm long) and insert a 0.018 in. wire. The track is then expanded with the dilator of the appropriate sheath to permit insertion of catheters of 5, 6, or 7 French diameter depending on the sheath size (e.g., the Cook KCFN [Cook Medical, Bloomington, IN, USA], Fig. 2.2). The advantage of this technique is that it requires significantly less force to insert the cannula through the wall of the vessel. This means that the artery is less likely to slip away from the cannula, it will not collapse as easily before the cannula, and perforation of the distal wall may be more easily avoided. Fig. 2.2 Sheath of 5, 6, or 7 French diameter with a dilator for a 0.018 in. nitinol wire that fits a 21 gauge cannula (Cook Medical). An 18 gauge catheter cannula 18 cm long (Fig. 2.3) can be very helpful in various situations: not the metal cannula but the plastic sheathing that has the same diameter as the customary puncture cannulas but is longer and more flexible. (A 4 French dilator may used in a similar manner. It is only slightly thicker, although less flexible.) Following are three examples: • A vessel wall is so hard that it is impossible to introduce a sheath over the normal wire. This is almost always remedied with the aid of an Amplatz guidewire (Boston Scientific, Natick, MA, USA). Introduce the long plastic cannula over the normal wire and then insert the Amplatz guidewire through this cannula. • In antegrade catheterization the wire comes to rest in the deep femoral artery. Before a larger hole is opened in the artery it should be determined whether the puncture has come too close to the deep femoral artery or has even punctured that artery. Switch to the long flexible plastic cannula. It is now possible to verify correct access far more reliably than with the rigid metal cannula. • When a patient has trophic changes in one or both feet (stage IV occlusive disease according to Fontaine), it is important to determine whether stenoses or occlusions of the arteries of the leg are present and require treatment. When the inguinal pulses are strong and there is no other sign of iliac arterial stenosis, the examination can be limited to angiography of the lower legs using an antegrade approach in anticipation of possible intervention: – Perform the puncture with the normal steel cannula. – Then switch to the plastic cannula over a short wire. – Perform angiography. – Determine whether intervention is indicated. The guidewire acts as the “rail” over which the catheter (according to Seldinger 1953) or sheath is introduced into the vessel (see Chapter 3). It allows curved and straight catheters to glide through vessels while preventing the catheter tip from injuring the vascular wall. Catheters or sheaths can be guided around curves and across bifurcations only with the aid of guidewires. They are the most important instrument in the interventional management of stenoses and occlusions. Every guidewire is composed of a metal core that determines its stiffness and flexible cladding. This is because a bare steel wire 0.9 mm in diameter would be far too stiff for most functions. The 0.035 in. wire (see Table 2.1 for units) is the most common wire thickness used in peripheral vessels today. Its core is a steel wire with a diameter between 0.35 and 0.44 mm (Fig. 2.4). It achieves its diameter of 0.9 mm because an outer wire 0.23 to 0.27 mm in diameter is wrapped around the core much like on the string of a guitar or piano. This type of wire is referred to as spring wire. Most wire designs have a fine straplike “safety wire” running longitudinally beneath the spring coil. This safety wire is fused to the spring coil at both ends and to the core as well at the back (proximal) end. This is necessary because the soft tip of the wire usually projects beyond the distal end of the core and is only held by this safety wire. The surface of the steel wire is usually covered with a thin coating of Teflon. This coating doubles the lubricity of the wire when moistened (Schröder 1993c). In between these normal spring wires and the extremely stiff but rarely used wires (such as Back-up Meier [Boston Scientific] or Lunderquist [Cook Medical]) there is a third class of wires known as Amplatz wires. This type is used when a particularly stiff wire is needed. Here the core is wrapped not with a round wire but with a flat steel band that allows a thicker core to be used with the same outer diameter (Fig. 2.5). A 0.035 in. Amplatz wire with a 0.55 mm core is six times as stiff as a standard wire with a 0.35 mm core. Stiffness is directly related to the 4th power of the core diameter (Schröder 1993a). In the very slender catheter systems initially developed for cardiology and now increasingly used in peripheral interventions the wires must be correspondingly thin. Their stiffness need no longer be reduced artificially by wrapping. An 0.018 in. wire of this sort with a diameter of 0.46 mm is as stiff as the average 0.038 in. spring wire. A 0.014 in. wire with a diameter of 0.35 mm corresponds to a 0.035 in. spring wire. Yet even these wires are wrapped. This wrapping is at the tip, which has the desired flexibility only when the core is drawn out very thinly and therefore must be protected by a spring coil or plastic sheathing. A very soft tip is desirable where the wire’s only function is to guide a catheter through open or possibly stenosed vessels atraumatically. This can be different where a wire with a straight tip (and therefore with the least possible resistance) is needed to guide a catheter through an occluded segment of a vessel (see Chapter 5, Superficial Femoral Artery, p. 159, and Arteries of the Lower Leg, p. 184). For this reason 0.014 in. wires are now supplied with varying tip thicknesses (such as the Cook CMW – 14 Series, 6–25 G). They are each designed for a different “tip load,” meaning the maximum resistance (gram) the straight wire can encounter without bending. Even more important is the design of the transition between the soft tip and the stiff shaft. Wires that have no such transition are nearly useless (see Chapter 3, Crossover Catheterization, p. 60). Here is an example for the effect of the gradual transition from a soft tip to a stiff shaft: Assume the curved end of a catheter has been introduced from the right iliac arteries across the bifurcation into the left common iliac artery. To advance the catheter further requires one to first advance a wire into the left iliac arteries. The soft tip of the wire follows the curvature of the catheter. If the stiff shaft of the wire then follows without any transition (Fig. 2.6a), the catheter will kink, and both catheter and wire will advance into the aorta. However if the wire only gradually becomes stiffer, then the wire can continue to follow the curvature of the catheter (Fig. 2.6b). Gradually it will expand this into a wider arc that even the increasingly stiff shaft of the wire can follow. This arc will finally become so wide that it will no longer fit into the aorta. Consequently, the wire (and the catheter) will only be able to advance into the contralateral iliac arteries (Fig. 2.6c). The length of this transition should be no less than 5 cm for standard wires. Significantly longer transitions (e.g., Bentson, long taper) are helpful for passages through certain difficult curves. Example: When one wants to use such a wire to guide a catheter across the aortic bifurcation, there is often a mismatch between the stiffness in the part that has passed the curve and the part being flexed in the bifurcation. The part of the wire that has passed the curve must continue to support the path around the curve. It may prove too soft to do this if the area of increasing stiffness is limited to just a few centimeters. The problem can be avoided by using a wire with a much longer transition (e.g., Bentson, long taper). However, there is often not enough space for this beyond the curve (e.g., in a renal artery). The obvious solution is to design the wire so that the core diameter (which determines stiffness) increases linearly from the tip to the shaft. This is exactly how most guidewires are designed. This design overlooks one thing: It results in stiffness that increases as the 4th power of the distance from the tip, which is certainly not optimal for every application. For such cases it may be best to have a wire in which it is not the core diameter (Fig. 2.7a) but the stiffness that increases linearly from the tip (Fig. 2.7b). Then the cross section of the wire in the transitional segment would have the shape of a parabola (4th power). The diameter of the core would be proportional to the 4th power root of the distance from the tip of the wire: Of course intermediate forms between these two extremes are conceivable. Fig. 2.6 Transition between soft tip and stiff shaft. a Wire with abrupt transition to stiff segment. b Wire with gradual transition to stiff segment. c Later phase of catheterization using wire with gradual transition to stiff segment. It is easy to put a bend in the tips of thin 0.014 or 0.018 in. steel wires (see Fig. 2.10). However, the tip can just as easily deform when it encounters resistance, which can quickly render such a wire unusable. A few special wires, such as the ones included in a puncture set for the radial artery or thin wires for catheterizing peripheral vessels (e.g., Terumo Glidewire Advantage, Terumo Interventional Systems, Somerset, NJ, USA) have a tip with a nitinol core, which is much more resistant to deformation (see below). Terumo introduced a completely different type of guidewire in the late 1980s. Its core is not made of steel but of nitinol, an alloy of nickel and titanium. Instead of being wrapped the wire has plastic sheathing with a hydrophilic outer coating, giving it excellent lubricity. Wires of the same material are now available from other companies as well. A nitinol wire is significantly less stiff (by a factor of 4, Schröder 1993a) than a steel wire of the same core diameter. This can be good or bad depending on the application. One clear advantage is that the wire is largely resistant to kinking. The greatest advantage is the hydrophilic coating. Wires with this coating have three times the lubricity of Teflon-coated wires when moistened (Schröder 1993c). That makes this “glide” wire the instrument of choice for catheterizing high-grade stenoses or occluded segments of vessels. It is also very helpful when guiding catheters or sheaths around difficult curves or across bifurcations. One disadvantage of glide wires is their vulnerable surface. For this reason one should avoid using them in combination with sharp cannulas when establishing vascular access. As the wire is withdrawn through the cannula, the cannula could cut into the wire’s plastic sheathing. Fig. 2.7 With a linear increase in core diameter, stiffness increases as the 4th power of the distance from the tip of the wire. To achieve a linear increase in stiffness, one needs a core with a diameter that increases as the 4th power root of the distance from the tip of the wire. a Linearly increasing core diameter. b Linearly increasing stiffness. The statement that nitinol wires are not deformable overlooks one interesting possibility: If one sharply kinks the wire, the wire will no longer be perfectly straight at that point (Fig. 2.8). A slight kink will remain, which will then be as stable as the wire’s previous straight shape (Fig. 2.8c, Schröder 1993b). Several such kinks create a bend. This can be very useful in certain situations, such as when guiding a balloon catheter past the deep femoral artery into the superficial femoral artery in crossover catheterization (see Chapter 3, Crossover Catheterization, p. 60). This method is also useful when you want to steer a bent glide wire from the brachiocephalic trunk into the right subclavian artery. In this case the original bend in the wire will often be too slight compared with the diameter of the brachiocephalic trunk (Fig. 2.9). Fig. 2.8 Shaping a nitinol wire. a Shaping a nitinol wire by putting a sharp bend in it. b Continuation of a. c Slight bend that keeps its shape: result of a and b. Fig. 2.9 Catheterizing the right subclavian artery. a At first it is not possible to catheterize the subclavian artery from the brachiocephalic trunk. b Additional bend in the glide wire. c A more pronounced bend causes the tip of the wire to enter the right subclavian artery. Glide wires are usually available as straight wires or slightly curved at the tip. Aside from the standard type there is also a stiff version. With a core diameter of 0.7 mm this wire is 5 times as stiff as the standard version with a core diameter of 0.46 mm (Schröder 1993a). Balloon catheters are almost always straight and can only be steered around curves or selectively introduced into certain branches with the aid of guidewires. This selective catheterization requires a wire with a suitable bend at its tip and rotational stability (Fig. 2.10). The spring wires typically do not have a solid connection between their wrapping and core and are therefore not suitable for actively steering around certain curves. However, this works very well with all wires that are not wrapped with a spring. Such wires include the thin steel wires (0.018, 0.014, and 0.012 in.) and glide wires. Fig. 2.10 To shape the tip of a steel wire, draw it between your thumb and a tightly held cannula. The greater the pressure, the more pronounced the bend. Like stiffness, the rotational stability of a wire is also proportional to the 4th power of its diameter (Schröder 1993a). An 0.018 in. wire has nearly 3 times the rotational stability of a 0.014 in. wire. The thin steel wires are usually supplied with a twist grip that makes it far easier to steer the wire precisely. Every wire in its tube should be moistened with saline solution prior to use. Inserting a curved guidewire into a cannula or catheter is greatly facilitated by the introducer supplied with every curved-tip catheter. It is usually very tightly inserted in the wire tube (see Fig. 2.12). When it is pulled out, it often comes out of the tube and off the wire all at once, so that one has to reinsert the curved tip. To avoid this, one should first withdraw the wire 10 to 15 cm out of the tube and then pull the introducer out of the tube. Then the introducer is pushed to the tip of the wire to extend the curve. When inserting the wire into a cannula or catheter, one grasps the wire a few centimeters behind the introducer (Fig. 2.11). This way one can bring the extended wire into a sufficiently stable position within the cannula or catheter in a single motion without having to change one’s grip on the wire (Fig. 2.11b). There are two types of introducers: • Those whose proximal end lies within the wire tube have a narrow lumen that is in contact with the wire along its entire length. When one inserts the wire through the valve of a sheath with this device, there is only slight blood loss. Fig. 2.11 Inserting the wire with an introducer. a Grasp the wire a few centimeters behind the introducer. b Insert the extended wire into the cannula or catheter in a single motion without changing your grip. • The glide wires usually come with an introducer whose proximal end lies over the end of the wire sleeve. This model is wide at its proximal end and narrows toward its tip like a funnel (Fig. 2.12). When one inserts the wire through the valve of a sheath with this device, the resistance to retrograde blood flow is significantly less and blood loss is greater. The elastic J tip in curved spring wires is created by a bend in the safety wire. The curvature also requires a little play between the coils of the wrapping. The safety wire is 1.5 to 2 mm longer than the tightly wound wrapping. This ensures the necessary play for the curvature (Fig. 2.13). Pulling apart the wrapping at another place on the wire will push the tip together and extend the curve. This means that, to insert the wire into a catheter without an introducer, one spreads the wrapping apart between the fingers (Fig. 2.13d). This will make the tip nearly straight. Fig. 2.13 Extending the end curve of a J wire. a Curved J wire: play between the coils of the wrapping. b J wire with tip extended. c To extend the J wire, it is grasped between the fingers. d The wrapping is spread apart by moving the fingers in opposite directions. Following are common names for a few special guidewires: Bentson: long soft tip (6 cm and long transition to stiff shaft [16 cm]). Long taper: similar characteristics to Bentson. Rosen: J wire with tight end curve, radius of curvature 1.5 mm, relatively stiff. Amplatz (Boston Scientific): stiff wire, see earlier discussion. Terumo (Terumo Interventional Systems): the original glide wire. A sheath is not generally required for simple angiography using only one catheter. This assumes that the tip of the catheter is in close contact with the guidewire so its passage into the vessel is relatively atraumatic (see Chapter 3, Angiography, p. 80, and Fig. 3.76, p. 84). Use of a sheath is recommended where one plans to change catheters several times or where one expects to encounter a very hard vascular wall. A sheath is absolutely indicated to protect the vascular wall in every intervention with a balloon catheter, stent, or similar device. The sheath is a thin-walled catheter with a valve and a lateral connection at its proximal end. The sheath is introduced into the vessel via a dilator that slides along the guidewire (Fig. 2.14). The most important quality criteria are smooth transitions with steps as little as possible between guidewire and dilator and between dilator and sheath. This requires a very strong material that retains its strength even when extruded to a thin layer and that will not crack upon encountering the resistance of a hard vascular wall. For the same reason the tip of the sheath must be in close contact with the dilator. The fact that it has a sharp distal end does not pose a problem. This is because the sheath is only advanced with the dilator in place within it. Fig. 2.15 Schematic diagram of a threaded connection between the sheath and dilator (Boston Scientific). There must also be a reliable connection between the sheath and dilator. Otherwise the resistance of the vascular wall upon entering the vessel could cause the dilator to be pushed out of the sheath: One would push the sheath but the resistance would act on the dilator. The press fit connection between dilator and sheath that most sheaths have is not always sufficient for this task. Only a threaded connection between the two components is reliable (Fig. 2.15). “Soft tip” sheaths (Fig. 2.16) should not be used. They have a higher step-off between dilator and sheath tip, making it hard to push them through a hard vascular wall. And because they are also soft they can become significantly deformed, tearing an unnecessarily large hole in the vascular wall. The valve prevents blood from escaping when there is no catheter in the sheath. It also seals the sheath against the catheter or guidewire. The sheath is flushed through the lateral connection. This connection also allows injection of contrast agent or medications. However, forceful injection can cause part of the fluid to spray out through the valve. This situation can be avoided by introducing the dilator or catheter and injecting through its lumen. Catheters are specified by their outer diameter but sheaths by their inner diameter (i.e., according to the catheter that fits in the sheath). A 6 French sheath is ~2.2 mm wide. Its outer diameter depends on the wall thickness but will usually be around 2.8 mm (8.3 French). Sheaths with a length of 9 to 11 cm are customarily used in daily routine. The length of 11 cm has an advantage over 9 cm because, in antegrade catheterization of the common femoral artery, it will better ensure a stable position within the superficial femoral artery. Sheaths are available in various shapes and sizes for specific tasks. Balloon-expandable stents in particular are most securely placed when they are advanced to the stenosis within a sheath. Treating iliac arterial stenosis in this manner requires a sheath 25 cm in length. Sheaths with a length of 40 cm are customarily used in crossover interventions. The sheath must have the following characteristics to be advanced across an acute-angle bifurcation (Fig. 2.17): • Either the dilator and sheath have a suitable bend (e.g., Cook Medical’s Balkin sheath, Figs. 2.17 and 2.18), • or a flexible dilator projects a few centimeters beyond the tip of the sheath. The dilator is first advanced across the bifurcation. It splints the bifurcation so the stiffer sheath can be advanced. (This is the principle of gradually increasing stiffness, as with the guidewires, e.g., the Arrow sheath [Arrow International, Inc., Reading, PA, USA]; Figs. 2.17 and 2.19). Fig. 2.18 Crossover catheterization with the Balkin Up and Over sheath from Cook Medical. a The wire lies deep within the contralateral iliac arteries. b The curved dilator follows the wire across the bifurcation. c The bend in the sheath facilitates advancing it into the contralateral iliac arteries. Because of their tendency to kink at a bifurcation, crossover sheaths usually have a spiral metal reinforcement in their wall. This makes them relatively stiff but also makes it easier to put a bend in them prior to insertion (even without steam heating) that will facilitate crossing the bifurcation. Sheaths of 6 French diameter were originally common for crossover catheterization. Now sheaths of suitable length are also available in 5 and 4 French diameters. These of course are more easily advanced across an acute-angle bifurcation. Sheaths 40 cm in length are normally used for interventions in the arteries of the lower leg. Guiding sheaths with various curvatures are supplied for interventions in the renal arteries (see Chapter 4, Renal Arteries, p. 121). The width and curvature of the aorta are the decisive criteria for adapting the sheath to the angles of the renal artery origins. Fig. 2.19 Crossover catheterization with the Arrow shealth (Arrow International) or OptiMed Epsylar. a The flexible dilator follows the wire across the bifurcation. b The dilator splints the bifurcation so the shealth can be advanced. Aspiration thrombectomy, at least when performed with a simple aspiration catheter, requires a sheath with a removable valve. Such sheaths are supplied in the appropriate length by OptiMed, Ettlingen, Germany; Angiomed GmbH, Baden-Würtemberg, Germany; or Terumo. The catheter is the most important instrument in every vascular intervention: It transports contrast agent. Together with the guidewire it provides an access path through almost every curve and bifurcation. And it acts as the carrier for balloons, stents, and other instruments. For nearly 3 decades angiography was performed without sheaths. For this reason it was only natural that the catheter tip was in close contact with the wire to ensure atraumatic entry into the vessel. Sheaths came into regular use only with the advent of interventional radiology. They would not normally be required for simple angiography if every manufacturer were to pay more attention to an optimally shaped catheter tip (see Fig. 3.76), and if every catheter had a tip like the pediatric catheter, the “self-dilating tip” of which is explicitly mentioned in the catalog. Note that a 4 French sheath creates an opening in the vascular wall twice as large (in terms of area) as the one a 4 French catheter alone would require. Manufacturers often specify possible flow rates of contrast agent (mL/s). This will depend on the following catheter characteristics: • Inner diameter • Length • Pressure-bearing capacity of the catheter wall All other conditions being equal, Poiseuille’s law indicates that the possible flow rate of contrast agent is directly related to the 4th power of the inner diameter. This means that doubling the inner diameter would allow a 16-fold increase in the flow rate. When the catheter length is doubled, the possible flow rate decreases by half, all other conditions being equal. (The flow rate can also be significantly increased by warming the contrast agent to body temperature and thus decreasing its viscosity.) Digital subtraction and the option of increasing contrast electronically have made it possible to work with significantly lower contrast flow rates than were required earlier in analog imaging. For this reason the maximum possible contrast flow rate only becomes a problem in very narrow catheters. The diagnostic and therapeutic instruments are steered through the vascular system using curved catheters and with the aid of guidewires. This is only possible where the curve at the distal tip of the catheter can be steered in a certain direction by rotating the proximal end of the catheter. This in turn assumes that the catheter has sufficient rotational stability (torsional strength). Rotational stability (torsional strength) is primarily a function of catheter diameter. It is approximately proportional to the 4th power of the outer diameter (more precisely as the difference between the 4th powers of outer and inner diameter, Do4–Di4). The material also plays a role. Older measurements have shown that the rotational stability (torsional strength) of Teflon, polyethylene, and nylon is approximately in the proportion of 1:2:4 (Schröder and Weber 1992). The same study revealed that wire reinforcement in the catheter wall can increase rotational stability (torsional strength) by a factor of 3. One material-related characteristic of catheters that has very important practical implications is the stability of the bends that are used to determine the catheter’s path through the vessel. These bends become less pronounced when the catheter is kept extended for a long time. Warming also has an unfavorable effect. On the other hand, one can use heat (steam) to change the shape of this thermoplastic material (see later discussion). The key quality criteria for a general aortogram include good contrast with minimal use of contrast agent and unobscured visualization of the vascular anatomy. Renal arterial stenosis is the most important example. It is often associated with impaired kidney function that can be exacerbated by large quantities of contrast agent. Therefore the contrast agent should be very selectively injected into the short segment from which the renal arteries (or other aortic branches of interest) arise. One should take particular care to prevent part of the contrast agent entering the aorta superior to the renal arteries. There are two reasons for this. First, it avoids a loss of contrast. Second, it also avoids contrast entering the superior mesenteric artery where it could obscure segments of a renal artery within the region of interest. The pigtail catheter most often used for this purpose (Fig. 2.20a) fails to fulfill these requirements satisfactorily. The end of its distal circular curve through which 30 to 50% of the injected contrast agent exits is directed cranially on most catheters. This results in loss of concentration and obscured anatomy. Part of the contrast agent is separated from the rest of the bolus so that the maximum possible contrast is not achieved. Fig. 2.20 Catheters for aortography. a Pigtail catheter. b Racket catheter with end hole in favorable position. c Racket catheter with end hole in unfavorable position. d Catheter with a 180° bend. Some “tennis racket” catheters avoid this problem, some do not. The racket, or tennis racket, catheter shown in Fig. 2.20b has a suitable shape: The hole at the distal end emits the contrast agent at the same level as the side holes. Fig. 2.20c on the other hand shows the end hole in the same position as on most pigtail catheters. All catheters with a 180° bend (Fig. 2.20d) reliably avoid this problem. Such models include Sos Omni Flush (AngioDynamics, Latham, NY, USA), ContraFlush (Boston Scientific), Universal Flush (Cordis, Miami, FL, USA), and VCF (Cook Medical). (The author has described a catheter that can be used to precisely inject contrast agent in the direction of the renal arteries in German Registered Design No. 20 2009 011 942.4.) Modern digital subtraction angiography systems are able to generate a single summation angiogram from a series of sequential images (see Fig. 3.72). This means it is no longer necessary to fill a 30 or 40 cm segment of the aorta completely. In the interest of using contrast agent sparingly it is best to follow Sos and Trost’s recommendation (Sos and Trost 2008) by reducing the number of side holes and concentrating on a short segment (Fig. 2.21b). A pigtail catheter is not suitable for precision steering around curves such as from right to left at the aortic bifurcation or from the left subclavian artery into the descending aorta. This is because the tip of the pigtail catheter points straight ahead in most cases (Fig. 2.22). The pigtail opens only when a stiff segment of the wire lies within the curve. And by then the wire is so far into the aorta that it can no longer be used to guide the catheter across the bifurcation or into the descending aorta. So-called sizing catheters (Fig. 2.23) are used wherever precise measurements of vascular diameter and the length of certain segments of the vessel are needed. These are usually aortography catheters bearing metal markings on their shaft at precisely defined intervals.
Size Specifications for Cannulas, Guidewires, Catheters, and Sheaths
Cannulas
Guidewires
“Glide” Wires
Wires for Steering Around Curves
Sheaths
Size Specifications for Catheters and Sheaths
Sheaths for Specific Tasks
Angiography Catheters
General
Transport of Contrast Agent
Rotational Stability (Torsional Strength)
Catheter Shape
Catheters for Aortography
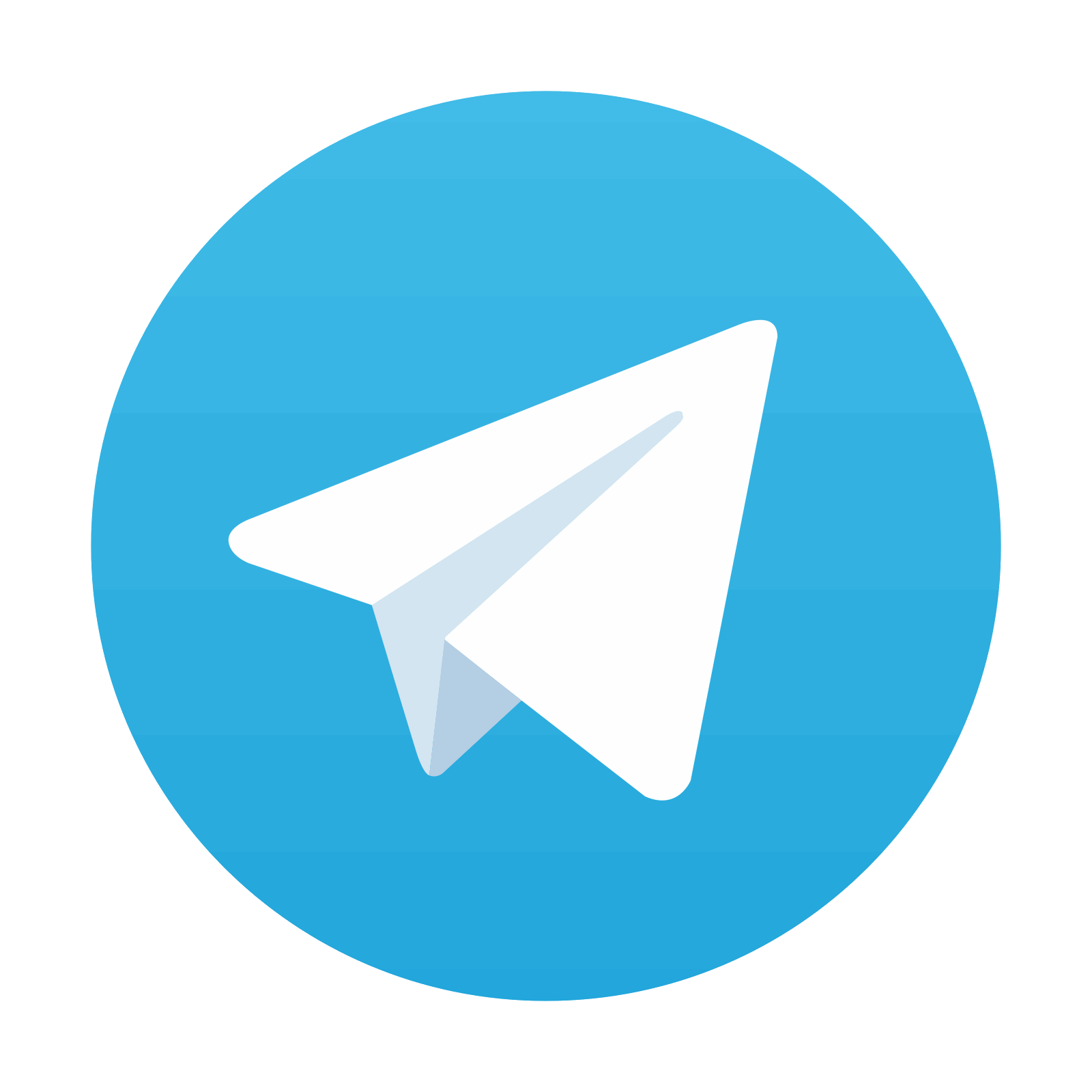
Stay updated, free articles. Join our Telegram channel

Full access? Get Clinical Tree
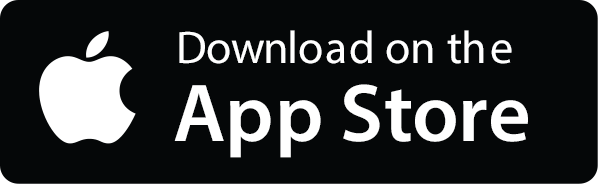
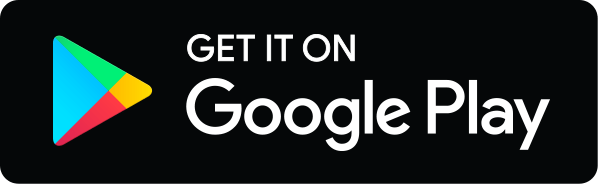