S. Di Saverio, G. Tugnoli, F. Catena, L. Ansaloni and N. Naidoo (eds.)Trauma Surgery2014Volume 1: Trauma Management, Trauma Critical Care, Orthopaedic Trauma and Neuro-Trauma10.1007/978-88-470-5403-5_2
© Springer-Verlag Italia 2014
2. Management of the Polytrauma Patient
(1)
Department of Surgery, Nelson R Mandela School of Medicine, University of KwaZulu-Natal, 719 Umbilo Road, Durban, Republic of South Africa
Abstract
Major hemorrhage results in a switch from aerobic to anaerobic metabolism with a profound depletion of ATP. The primary goal of resuscitation is to revert to aerobic metabolism by addressing three major physiological disturbances, namely, hypoxia, hypoperfusion, and hypothermia. Early intubation and mechanical ventilation are essential interventions although protective lung ventilation should not be employed during the acute stage. Excessive use of clear resuscitation fluids should be avoided, and a massive transfusion protocol using blood and blood products in appropriate ratios must be instituted. Hypothermia is an independent predictor of mortality and rapid core rewarming is essential. Computerized scanning with angiography is the gold standard for the identification of injuries but should only be undertaken if physiology allows. Acute surgical intervention must address only life- or limb-threatening injuries and definitive fracture fixation undertaken at a later stage.
Traumatic brain injury (TBI) and hemorrhage account for the vast majority of early trauma deaths [1], the latter contributing to severe secondary brain injury. Primary TBI is irreversible, whereas the effects of hemorrhage on both cerebral and other body systems may be countered by aggressive and appropriate resuscitation which is the first critical step when managing polytrauma. The goal of resuscitation is to normalize oxygen delivery (DO2) and oxygen consumption (VO2) and restore aerobic metabolism. Thereafter, the management consists of identifying injuries, prioritizing surgical management, and preventing or supporting organ dysfunction.
The switch from anaerobic to aerobic metabolism is a crucial goal and is underscored by a few simple biochemical facts. Adenosine triphosphate (ATP) is virtually the sole energy source for the myriad of life-sustaining human cellular reactions and is produced during oxidative phosphorylation within the mitochondria by Krebs cycle. At any one time the body contains only 100 g of ATP, yet the average daily requirements are between 100 and 150 kg. This means that at least 200 mol of ATP (each mole equals 0.5 kg) must be manufactured each day which, in molecular terms, amounts to 12 × 1025 molecules of ATP. Each cell contains about one billion molecules of ATP which are recycled every 20–30 s. During anaerobic metabolism, ATP production falls by more than 90 % and from the above simplified overview, it is patently obvious why such a metabolic state is catastrophic.
The initial priority is to prevent early death from the physiological mayhem of anaerobic metabolism by appropriate resuscitation and surgical intervention, with the secondary goal of preventing late deaths from multiple organ dysfunction (MOD). Interventions undertaken within the first few hours impact heavily on the incidence of MOD, and rapid decisions need to be made, sometimes in the presence of limited information. At every decision point the risk of a particular course of action needs to be weighed against the benefits; acute benefit outweighs later risk. For example, although the use of intravenous contrast during computerized scanning carries the risk of renal damage, it is of enormous benefit in identifying injuries and planning management.
2.1 Converting Anaerobic to Aerobic Metabolism
Although the lethal triad of hypothermia, acidosis, and coagulopathy is a well-accepted consequence of severe hemorrhage [2] and inadequate DO2, this is a later phase and the forerunners of this have been recognized as hypoxia, hypoperfusion, and hypothermia, the “triple H syndrome” [3]. All three parameters induce a lactic acidosis, have an independent association with mortality, and their correction must be the main aim of resuscitation.
2.1.1 Hypoxia
Hypoxia is not synonymous with an abnormal PaO2 or SaO2 on blood gas analysis and encompasses the inevitable oxygen debt and accumulation of lactic acid from anaerobic metabolism. The aims of resuscitation are to prevent further accumulation of oxygen debt and institute rapid repayment [4]. Of prime importance is the realization that despite normalizing DO2, an oxygen debt must still be repaid as indicated by a persistently elevated lactate or base deficit.
Despite an acceptable level of arterial oxygen saturation, endotracheal intubation and mechanical ventilation should be employed liberally in patients with a lactic acidosis. The lungs are the main buffering mechanism by which humans compensate for an acute metabolic acidosis, renal compensation being slower and later, and removing the work of breathing may help substantially. The drug for induction and intubation must be carefully selected in order to avoid further cardiovascular instability, and ketamine and etomidate are the agents of choice despite the reported association of adrenal insufficiency with the latter [5]. This is a late and only potential risk, whereas the effects of hypotension are definite and acute.
Recently the concept of protective lung ventilation (PLV) has been advocated for the critically ill and injured [6], but this is not universally applicable in major trauma, especially during the acute phase. An adequate minute volume is required to buffer a metabolic acidosis; to avoid rises in intracranial pressure following TBI, hypercarbia must be prevented and tidal volumes may need to be higher than those proposed for PLV; and in patients with blunt thoracic trauma and a pulmonary contusion, a substantial proportion of damaged lung may be recruited by initially using higher tidal volumes between 8 and 10 ml/kg. Once recruited the tidal volume may be reduced as necessary, and lung volume maintained with positive end expiratory pressure (PEEP).
2.1.2 Hypoperfusion
Major hemorrhage results in intravascular volume depletion and a significant drop in cardiac output and DO2. Short periods of hypotension although causing cellular apoptosis result in complete recovery of ATP production, whereas protracted shock episodes cause cell necrosis and irreversible organ damage [7]. The conventional approach has been to restore intravascular volume with clear fluids, either crystalloid or colloid, and augment DO2 later with blood transfusion. Although restoring intravascular volume and perfusion, clear fluids do not carry oxygen, and a proactive rather than reactive philosophy has recently been adopted with the early use of blood and blood products and limitation of clear resuscitation fluids. To this end a massive transfusion protocol must be established [8] whereby the necessary products are released urgently and as required with the aim of achieving a set ratio of packed red blood cells (PRBCs) to plasma and platelets. This has the desired effect of rapidly optimizing DO2 and counteracting the effect of the acute coagulopathy of trauma. Experience from military conflicts suggested initially that PRBCs, plasma, and platelets should be administered in a 1:1:1 ratio [9], but evidence from the civilian environment indicates that a less aggressive plasma volume is superior with a PRBC to plasma ratio of 2:1 being preferred, thereby minimizing complications without affecting survival [10]. There is no role for supranormal DO2, and the use of permissive hypotension is only applicable in the setting of uncontrolled hemorrhage where theater is immediately available. Sustained hypoperfusion results in irreversible organ ischemia and a physiological point of no return.
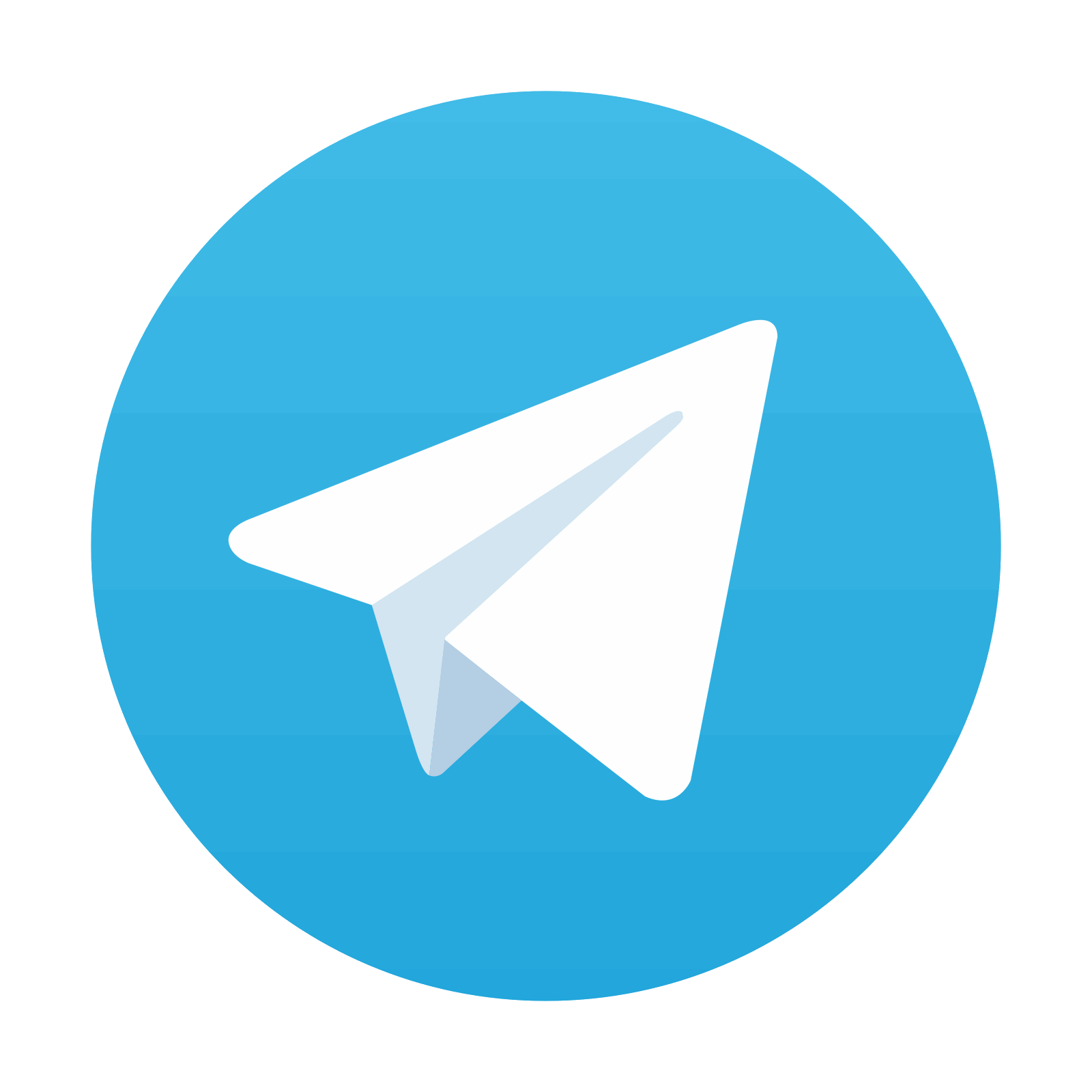
Stay updated, free articles. Join our Telegram channel

Full access? Get Clinical Tree
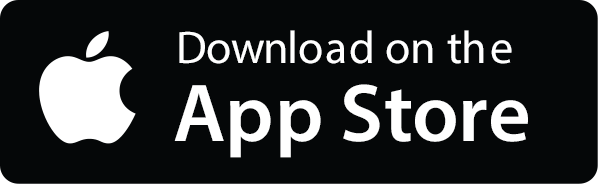
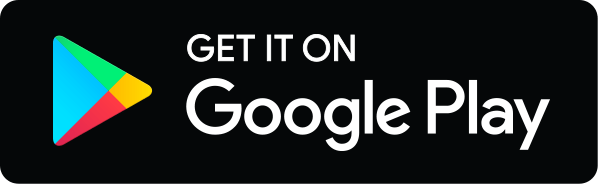