Abstract
The care of neonates, infants, children, and adults with acquired and congenital heart disease (CHD) recovering from cardiac surgery presents one of the most complex and rapidly evolving challenges for the cardiac intensive care provider. Successful pediatric cardiac surgery requires a comprehensive, well-coordinated team-based mode of care delivery that incorporates a broad knowledge base with efficient and sound clinical judgment. Management of the postoperative patient should reflect a firm understanding of acquired heart disease and CHD, cardiopulmonary anatomy and physiology, surgical interventions, cardiopulmonary bypass, advanced technical skills, and pharmacology. This chapter aims to provide a comprehensive overview of the care of the postoperative patient with a focus on complications and those evidence-based management strategies that can minimize the morbidity and mortality experienced in the postoperative period.
Key words
cardiac surgery, congenital heart disease, complications, hemodynamic monitoring, cardiopulmonary interactions, low cardiac output syndrome, arrhythmias
The critical care of neonates, infants, children, and adults with acquired heart disease and/or congenital heart disease (CHD) recovering from cardiac surgery has evolved over the last 40 years, paralleling improvements in pediatric cardiac surgical techniques, pediatric cardiac anesthesia, pediatric cardiac nursing, and cardiology. Although the complexity and heterogeneous nature of CHD have not changed, our understanding of the physiologic derangements that occur in the postoperative period has grown along with the subspecialization of pediatric cardiac critical care practitioners. Identifying risk factors for postoperative complications continues to remain a focus among cardiac critical care practitioners, cardiothoracic surgeons, cardiologists, anesthesiologists, and cardiac nurses. Yet translating our understanding of risk factors into effective and efficient management strategies remains the next frontier for the practice of pediatric cardiac critical care.
The recovery period after cardiac surgery represents one of the highest-risk periods for patients with CHD requiring palliative or corrective surgery. Successful congenital heart surgery requires a comprehensive, well-coordinated team-based care delivery model that incorporates several key elements of postoperative care: a broad knowledge base of the patient’s preoperative condition; precise anatomic diagnosis of the congenital heart defect; pathophysiologic impact of the defect(s) on cardiopulmonary and other organ system function; noncardiac medical and surgical history; anesthetic agents used in the operating room; details of the operative procedure and cardiopulmonary bypass (CPB) strategy; intraoperative complications, including dysrhythmias and bleeding; real-time interpretation of vital signs, hemodynamic data, and physical examination results; pharmacology of drugs effecting cardiovascular homeostasis; management of respiratory support devices; and indications for advanced procedures such as pacing and mechanical circulatory support.
Anticipating postoperative complications is as much an art form as it is a science. Pattern recognition and the complex incorporation of bedside physiologic data with limited evidence-based practice strategies constantly challenge the practitioner. This chapter aims to inform cardiac critical care clinicians with an informative appraisal of the current state of postoperative care for the patient with acquired heart disease and CHD, with a focus on the management of postoperative complications and conditions.
Cardiopulmonary Bypass in Neonates, Infants, and Children—Impact on Postoperative Care
CPB stimulates complement activation and the release of endothelial, parenchymal, and inflammatory cell-derived mediators, leading to a proinflammatory cascade. This was termed the systemic inflammatory response syndrome (SIRS) by the American College of Chest Physicians and Society of Critical Care Medicine in 1992; we now know that noninfectious triggers such as exposure of blood elements to the nonendothelial lining of the CPB circuit stimulate a SIRS state. The hallmark of SIRS is an increase in microvascular permeability and the development of interstitial edema. In addition to SIRS, ischemia-reperfusion injury (IRI) contributes substantially to the overall inflammatory state, as well as to the injury of the organ(s) that undergo reperfusion. In an additive fashion SIRS and IRI induce myocardial injury, causing ventricular systolic and diastolic dysfunction and parenchymal lung injury, causing pulmonary edema and impairing respiratory mechanics and gas exchange. In addition, IRI injures the pulmonary endothelium, contributing to a state of heightened vascular reactivity. The SIRS response also impacts other organ functions, contributing to central nervous system (CNS), kidney, mesenteric, and endocrine injury and dysfunction.
Postoperative Cardiac Dysfunction—Pathophysiology
The interplay of the inflammatory response to CPB, IRI, cardiac surgery, and age and developmental status place the patient at risk for developing a low cardiac output syndrome (LCOS) following surgery. LCOS is a historical term that seeks to encapsulate the transient myocardial injury and dysfunction resulting from the effects of CPB, direct myocardial trauma, and the lingering impact of preoperative ventricular volume and pressure loads. This transient depression in cardiac function and cardiac output (CO) generally improves after the first 24 hours. Table 33.1 highlights the hemodynamic profile of conditions that can lead to cardiovascular instability in the postoperative period.
HR | CVP | Pulse Pressure | MAP | Urine Output | Capillary Refill Time | |
---|---|---|---|---|---|---|
Hypovolemia | ↑ | ↓ | Normal to narrowed | ↓ | ↓ | ↑ |
Tamponade | ↑ | ↑ | Narrowed | ↓ | ↓ | ↑ |
LCOS | ↑ | ↓/↑ | Narrowed | ↓ | ↓ | ↑ |
Contributing factors to the development of postoperative LCOS include intravascular volume depletion secondary to excessive ultrafiltration, hemorrhage, excessive diuresis or inadequate fluid administration, and excessive capillary leak post CPB. Complicated surgical procedures associated with long CPB times may result in myocardial swelling and/or excess bleeding. Both sequelae may prohibit immediate sternal closure. Hemodynamic instability or ongoing bleeding can be managed more efficiently with delayed sternal closure, although mechanical ventilatory strategies must reflect the changes in functional residual capacity (FRC) and respiratory compliance that occur with sternal closure. Delayed sternal closure can be associated with transient respiratory deterioration and a similar decrease in cardiac output, often requiring a temporary escalation of ventilatory and vasoactive support and increase in intravascular volume.
Despite several working definitions for LCOS, a lack of consensus and variability in diagnostic criteria have limited scientific query into its epidemiology. Current definitions place emphasis on therapeutic interventions (e.g., inotrope dosing), yet efforts to refocus diagnostic criteria on patient-specific markers of impaired oxygen delivery have also gained traction, suggesting LCOS could be rephrased as “post–cardiac surgery shock” or “CPB-induced shock.”
Hemodynamic Monitoring in the Postoperative Period
The transition of care from the cardiac operating room to intensive care unit (ICU) occurs at a time of significant hemodynamic vulnerability, often accompanied by a complex interplay of cardiopulmonary pathophysiology and pharmacologic manipulation(s). At its core the goal of postoperative care is to optimize oxygen and substrate delivery to the myocardium and key organs while minimizing oxygen demands and other acquired comorbidities. This overarching principle of postoperative care is translated into intense vigilance and focus on invasive and noninvasive markers of cardiac function, CO, systemic oxygen delivery, and tissue oxygenation. Qualitative and quantitative determination of these parameters can be accomplished by routine physical examination aided by the interpretation of hemodynamic data, including transthoracic echocardiography, and indicators of tissue oxygenation such as serum lactate levels, near-infrared spectroscopy (NIRS), and venous oximetry. Additional parameters that are essential to determining the hemodynamic profile include the central venous pressure (CVP)/right atrial pressure (RAP), heart rate, blood pressure, and in some patients a left atrial pressure. The monitoring of urine output also provides some indication of CO and renal perfusion.
Several studies have demonstrated the limitations of the standard assessment of cardiac function, CO, and tissue oxygenation, which relies on conventional “hemodynamic” monitoring (CVP, heart rate, blood pressure, and urine output) and the physical examination. Several investigations have demonstrated discordant values between estimations and measurements of the aforementioned parameters, which occur irrespective of training and level of experience. The clinician should have a low threshold for using adjunctive monitoring modalities, including NIRS and venous oximetry, serial lactate levels, and transthoracic echocardiography. Several studies in adults and children have demonstrated the utility of venous oximetry in assessing the adequacy of tissue oxygenation. It should be appreciated that oxygen extraction increases well before serum lactate production exceeds clearance and levels begin to rise. NIRS has been studied extensively in animals and clinical studies validating regional cerebral oxyhemoglobin saturation with jugular and superior vena cava saturations. In some cases the use of transpulmonary or pulmonary artery thermodilution may be indicated, providing a measurement of CO as well as enabling the derivation of pulmonary vascular resistance (PVR) and systemic vascular resistance (SVR). The pulmonary artery catheter also enables the clinician to determine the left ventricular filling (LV) pressure and, if pulmonary hypertension (PH) is present, differentiate between pulmonary venous hypertension and/or pulmonary arteriolar disease. Please refer to Chapter 22 for further in-depth discussion of monitoring systems in the cardiac ICU (CICU).
Postoperative Cardiac Dysfunction—Medical Management
Several pharmacologic agents are available to aid in the manipulation and optimization of ventricular loading conditions and, if necessary, to provide inotropic support. The mechanisms of action and side-effect profile of each vasoactive agent should be weighed on an individual basis for each lesion and/or condition in the postoperative period ( Table 33.2 ). Catecholamines include dopamine, dobutamine, norepinephrine, epinephrine, and isoproterenol, all of which increase heart rate, inotropic state, and myocardial oxygen consumption. Atrial and ventricular dysrhythmias can be seen with higher frequency when using these agents. Moderate to high doses of dopamine, epinephrine, and norepinephrine vasoconstrict venous capacitance vessels (increasing systemic venous return and ventricular end-diastolic pressure) and arterial resistance vessels, which will decrease stroke volume if systolic function is impaired. Phosphodiesterase type 3 inhibitors (e.g., milrinone) and dobutamine provide inotropic support while vasodilating venous capacitance and arterial resistance vessels. Milrinone has less chronotropic effect than catecholamines and is immune to adrenergic receptor desensitization. If systolic function is severely impaired, epinephrine may be indicated because it provides unparalleled inotropic support without increasing SVR at low to moderate doses (<0.03 to 0.20 µg/kg/min). Another consideration is the use of calcium chloride as a continuous infusion, especially in neonates, who are sensitive to exogenous calcium due to an immature sarcoplasmic reticulum. Calcium offers the benefit of increased contractility without chronotropy. Although not approved by the US Food and Drug Administration, levosimendan is a calcium sensitizer with inotropic properties used in international centers. Levosimendan provides an alternative pharmacologic approach for patients experiencing postoperative cardiovascular dysfunction/shock and/or heart failure. The effects of pure vasodilating agents such as nitroprusside and nitroglycerin are limited to altering ventricular loading conditions. Both agents vasodilate venous capacitance and arterial resistance vessels, with nitroglycerin having a significant impact on SVR at moderate to high doses (>3 µg/kg/min).
RECEPTOR BINDING | ||||||
---|---|---|---|---|---|---|
Drug | Dose Range | α1 | β1 | β2 | DA | Major Side Effects |
Dopamine | 2-20 µg/kg/min | +++ | +++ | ++ | ++++ | Hypertension, ventricular arrhythmias, cardiac ischemia, tissue necrosis with extravasation |
Dobutamine | 2-20 µg/kg/min | + | ++++ | +++ | N/A | Tachycardia, hypertension, ventricular arrhythmias, cardiac ischemia, hypotension |
Norepinephrine | 0.01-1.0 µg/kg/min | ++++ | +++ | ++ | N/A | Arrhythmias, bradycardia, peripheral ischemia, hypertension |
Epinephrine | 0.01-0.2 µg/kg/min; 1 mg IV Q 3-5 min (max 0.2 mg/kg) | +++ | ++++ | +++ | N/A | Ventricular arrhythmias, severe hypertension, cardiac ischemia, cerebrovascular hemorrhage |
Isoproterenol | 0.01-1.0 µg/kg/min | ++++ | ++++ | N/A | Ventricular arrhythmias, cardiac ischemia, hypertension, hypotension | |
Phenylephrine | 0.01-1.0 µg/kg/min | ++++ | N/A | Reflex bradycardia, hypertension, severe peripheral and visceral vasoconstriction, tissue necrosis with extravasation |
Management of the Shunt-Dependent Single-Ventricle Patient
Patients with single-ventricle physiology undergoing shunt placement and/or first-stage palliation for hypoplastic left heart syndrome (HLHS) are at significant risk for developing postoperative cardiovascular dysfunction and shock. The limitations of the neonatal myocardium, cardiac surgery, the adverse effects of CPB and IRI on the myocardium, and the inefficiencies of a parallel circulation present an enormous challenge to the critical care clinician in the management of these patients.
The immediate postoperative period following the Norwood procedure involves dynamic changes in circulatory function, with risks for pulmonary overcirculation at the expense of systemic oxygen delivery. This tenuous balance between the systemic and pulmonary circulations is often expressed as a ratio of pulmonary perfusion (Q p ) to systemic perfusion (Q s ), or Q p :Q s , with the partitioning of total CO into Q p and Q s determined by the relative resistance in each circulation. As SVR rises, the Q p /Q s ratio increases, which may result in inadequate Q s . It is particularly important to make a timely and accurate assessment of Q s in these patients because as Q s wanes, neurohormonal activation increases, driving further increases in the Q p /Q s ratio and decreases in Q s . The use of NIRS and venous oximetry as discussed previously is useful for assessing the adequacy of Q s ; it is also imperative to understand that the arterial oxygen saturation (SaO 2 ) and blood pressure provide little if any indication of the adequacy of Q s . Using nonselective vasodilators reduces SVR and PVR, increasing stroke volume and simultaneously increasing Q s and Q p (increasing arterial oxygen content), with the net effect being a marked increase in systemic oxygen delivery. In cases of impaired ventricular systolic function, inotropic support in the form of epinephrine may be required, although doses higher than 0.3 µg/kg/min should be avoided due to unwanted vasoconstriction of arterial resistance vessels. If Q s remains inadequate despite the manipulation of vasoactive therapy, then mechanical support in the form of extracorporeal membrane oxygenation (ECMO) may be necessary. Please refer to Chapter 39 for further in-depth discussion of ECMO in the CICU.
Shunt Malfunction
The surveillance of aortopulmonary shunt function in the postoperative period requires an understanding of the causes of arterial hypoxemia: parenchymal lung disease leading to pulmonary venous desaturation, inadequate Q p , and/or low mixed venous saturation due to limited Q s (in the presence of intracardiac and/or pulmonary shunt). Partial aortopulmonary shunt obstruction leads to hypoxemia and impaired carbon dioxide (CO 2 ) elimination due to wasted ventilation, the latter of which is reflected in an arterial to end-tidal CO 2 gradient. Auscultation may be effective in ruling out a complete occlusion, but more advanced imaging is required to accurately confirm the diagnosis. Echocardiography with color Doppler assessment lacks adequate sensitivity to detect shunt malfunction. The definitive assessment of shunt function requires angiography.
Shunt obstruction that occurs immediately after surgery most likely requires reoperation, although some cases that involve narrowing at the proximal/distal anastomotic site may be treated with percutaneous balloon angioplasty. Medical interventions to temporize the patient with acute shunt malfunction include pharmacologic manipulation of SVR to increase the perfusion gradient across the shunt (epinephrine/phenylephrine), increasing the partial pressure of alveolar oxygen (supplemental O 2 ), immediate systemic anticoagulation (50 to 100 U/kg heparin), and the rapid reduction in O 2 demand/consumption (sedation, muscle relaxation, and mechanical ventilation). Surgical and/or catheter based intervention is the ultimate goal although a subset of shunt-dependent patients may require ECMO for immediate rescue after cardiac arrest.
Cardiopulmonary Interactions in the Postoperative Period
Optimizing the care of critically ill children with congenital and/or acquired heart disease requires an understanding of the physiologic principles that govern the interactions between the cardiovascular and respiratory systems. Respiratory mechanics and cardiovascular function are linked primarily through changes in lung volumes and intrathoracic pressure (ITP).
Positive pressure ventilation (PPV) increases ITP, decreasing the transmural pressure of the right atrium, which causes the RAP to increase and the pressure gradient for systemic venous return to decrease. Subjects with low CO due to inadequate ventricular filling (diastolic dysfunction), may benefit from spontaneous respiration or minimizing ITP during PPV. Right ventricular (RV) afterload is also affected by respiration. In addition to blood pH and alveolar oxygen tension, changes in lung volume and ITP also impact RV afterload. As lung volume rises above FRC, PVR increases due to compression of interalveolar vessels and the creation of zone I conditions. This is particularly important to consider in patients with elevated PVR and/or RV dysfunction. Respiration also effects LV afterload through changes in ITP. As ITP rises, the transmural pressure for the thoracic arterial system decreases, the volume within these structures decreases, and as a result the pressure within rises relative to the extrathoracic arterial system, creating a waterfall-like effect, enhancing the egress of blood from the intrathoracic to extrathoracic compartment. The benefits of PPV in patients with systolic heart failure cannot be overstated. The benefits are greatest in those patients with exaggerated negative ITP due to impaired airway/lung mechanics or elevated minute ventilation (as seen in patients compensating for a metabolic acidosis) and/or in patients with impaired systolic function of the systemic ventricle. An additional so-called cardiopulmonary interaction occurs when blood flow is redistributed from the respiratory muscles to other vital organs with the initiation of mechanical ventilation in a limited CO state. Under normal conditions, respiratory muscle oxygen consumption is minimal. However, when the respiratory load is increased (as described earlier), respiratory muscle (pump) metabolic demand rises, requiring a commensurate increase in respiratory pump perfusion. Mechanical ventilation unloads the respiratory pump, decreasing respiratory muscle oxygen demand, allowing for a redistribution of a limited CO to other vital organs.
Extubation Failure
Respiratory failure due to diaphragmatic paresis or paralysis may manifest as extubation failure in neonates and infants because they rely on diaphragmatic contraction for chest wall expansion more than older children. Intraoperative injury to the phrenic nerve (usually left-sided) is most commonly caused by direct trauma, although thermal injuries have also been reported. The diagnosis is confirmed by fluoroscopy or by bedside ultrasonography, demonstrating paradoxical or paretic motion of a raised hemidiaphragm in conjunction with paradoxical abdominal wall motion. In such cases, diaphragmatic plication is recommended should the patient demonstrate increased work of breathing and/or failure to wean from mechanical ventilation.
An infrequent complication from open-heart surgery that parallels injury to the phrenic nerve is trauma to the recurrent laryngeal nerve, which results in unilateral vocal cord paralysis and leads to a hoarse voice or weak cry. Because airway protection is potentially compromised, previously normal feeding neonates can demonstrate signs of aspiration (coughing, gagging, respiratory distress, and hypoxemia) when bottle-fed. Vocal cord dysfunction is transient in the majority of patients, yet consultation with an otorhinolaryngologist or speech-language therapist may help determine if the child will require thickened oral feeds or will be completely dependent on nasogastric (NG) feeds.
Although many postoperative CHD patients remain intubated and mechanically ventilated after surgery, specific patient populations may benefit from early extubation. Older children and those with less complex lesions requiring shorter CPB times have been shown to be successfully extubated in the operating room or within hours of arrival to the ICU. Several criteria should be evaluated to minimize the incidence of respiratory failure following extubation in the postoperative patient ( Box 33.1 ). The patient’s hemodynamic status should be evaluated with the goal of maintaining adequate perfusion at an acceptable heart rate; the patient should demonstrate adequate gas exchange with normal respiratory effort; hemostasis should have been achieved; and the patient’s neurologic status should be evaluated, titrating sedation and analgesia accordingly.
Heart rate: normal for age (± 1 standard deviation)
Rhythm: normal sinus rhythm or with stable source of rhythm generator (temporary/permanent pacemaker)
Cardiac output: adequate markers of oxygen delivery AND minimal pharmacologic support (epinephrine <0.03 µg/kg/min, dopamine <5 µg/kg/min, milrinone <0.5 µg/kg/min)
Oxygenation: adequate per lesion, goal FiO 2 <40%
Respiratory demand: spontaneous breathing, positive end-expiratory pressure (PEEP <8), assessment for obstructive airway disease (bronchomalacia) and/or neuromuscular weakness
Neurologic status: airway protective reflexes intact
Hemostasis : adequate
FiO 2 , Fraction of inspired oxygen.
Pleural and Pericardial Diseases
Pleural effusions may also complicate the postoperative course. Severe cases can compromise cardiopulmonary function and may lengthen the CICU and/or hospital stay. The majority of filtered fluid is reabsorbed by the microvasculature (90%), with the remaining fluid captured by the lymphatic system, which drains into the central venous system via the thoracic duct. An elevated central venous pressure, commonly observed after repair of tetralogy of Fallot and the Fontan procedure, decreases lymphatic clearance and may lead to a transudative pleural effusion. Conversely, exudative pleural effusions are caused by increased microvascular permeability related to nonspecific inflammation. Chylothorax is a variant of pleural effusion that must be considered in the postoperative period, especially in patients with high-risk comorbidities (age <1 year, single-ventricle physiology, extracardiac anomalies, longer CPB time, and thrombosis associated with an upper-extremity central venous line). Causes of chylothorax in the postoperative period include traumatic disruption of the thoracic duct and thoracic duct occlusion due to venous thrombus and/or external compression, leading to elevated central venous pressures with subsequent rupture of thoracic duct. Chylous drainage causes a significant loss of plasma proteins, lymphocytes, and lipids, leading to lymphopenia and impaired immunologic function, coagulation abnormalities, and malnutrition, all of which requires an integrative and intensive multidisciplinary approach to management.
Pneumothorax is an equally serious complication that results from air entry into the pleural space(s), often resulting from surgical trauma or PPV-induced alveolar rupture. Prophylactic percutaneous insertion of catheters in the pleural space is often performed after cardiothoracic surgical procedures in which pleural spaces have been opened. Applying continuous negative-pressure suction (15 to 20 cm H 2 0) to these tubes minimizes potential cardiorespiratory compromise. Tension pneumothorax is a life-threatening form of pneumothorax that requires emergent intervention due to hemodynamic compromise related to the rapid increases in intrathoracic pressure and subsequent limitation of systemic venous return. Needle decompression followed by thoracostomy tube insertion allows for emergent stabilization of cardiopulmonary function.
Pericardial effusions may cause hemodynamic compromise in the early and late postoperative period. Acute and rapid accumulation of fluid in a noncompliant pericardial space in the early postoperative period will lead to a significant decrease in the right atrial transmural pressure, causing the right atrium to collapse and the central venous pressure to rise. Early clinical manifestations include tachycardia with evolution to exaggerated respiratory variation in systolic blood pressure (pulsus paradoxus) with or without associated fever, chest pain/discomfort, irritability, nausea, poor appetite, and intolerance of feeds in the infant. The physical examination reveals a pleural or pericardial rub, and chest radiography confirms a widened mediastinum with occasional accompanying pleural effusions. Mild cases require observation with serial examinations and echocardiograms. However, moderate effusions with symptoms usually require treatment with nonsteroidal antiinflammatory agents and close follow-up. Larger pericardial effusions may require pericardiocentesis to prevent cardiac tamponade, and chronic effusions may require a surgically created pericardial window.
Pulmonary Hypertension
Pulmonary hypertension (PH) presenting in the postoperative period contributes substantial morbidity and mortality to the CHD population. Acute pulmonary hypertensive crises are life-threatening emergencies requiring immediate aggressive treatments to avoid circulatory collapse.
The mainstay of therapy for acute PH involves optimizing RV loading conditions, augmentation of RV contractility, and avoiding triggers that may precipitate and/or contribute to a PH crisis. Caution must be taken when considering volume loading because increases in RV diastolic volume and pressure may reduce LV compliance and filling via encroachment of the interventricular septum on the LV during diastole. In some situations, acute diuresis and/or phlebotomy may be necessary. Table 33.3 lists several pharmacologic treatment options for the management of acute PH in the CICU. Inhaled nitric oxide (iNO) is a specific pulmonary vascular smooth muscle relaxant that can lower pulmonary artery pressure in a number of diseases without the unwanted effect of systemic hypotension. Abrupt withdrawal of iNO can lead to rebound PH, thus requiring a slow wean from support. An additional therapy that may attenuate or prevent a withdrawal phenomenon after weaning iNO includes the use of sildenafil, a phosphodiesterase type 5 inhibitor. Pretreatment with sildenafil, which is well tolerated and available as an oral preparation, produces acute and relatively selective pulmonary vasodilation while acting synergistically with iNO. Hyperventilation was previously proposed as a strategy for the management of PH but is no longer used because it causes a compensatory increase in SVR, decrease in cardiac output, and reduction of cerebral blood flow. However, adequate ventilation and avoidance of hypercapnia remains important. In addition to avoiding known triggers, analgesia and sedation in the ICU play a large role in avoiding or treating postoperative PH crises. Please refer to Chapter 71 for further in-depth discussion of PH in the CICU.
Drug | Dose | Comment |
---|---|---|
Epoprostenol intravenous | Start with 1-2 ng/kg/min, increase gradually to 60 ng/kg/min IV | Caution: systemic arterial hypotension Need to change drug vial/delivery system every 12-24 h |
Iloprost inhaled, IV | 0.25 µg/kg inhaled, max. 10 µg; 6×/day or 1-5 ng/kg/min IV | Caution: systemic arterial hypotension |
iNO | 2-40 ppm inhaled | |
Sildenafil intravenous | 0.4 mg/kg bolus over 3 h (optional), then 1.6-2.4 mg/kg/day continuous infusion | Do not exceed 30 mg/d. Higher sildenafil doses up to 7.2 mg/kg/day IV have been used in newborn infants with PPHN associated with congenital diaphragmatic hernia |
oral | Weight 8-20 kg: 10 mg TID Weight >20 kg: 20 mg TID | In children weighing <8 kg, dosage of 1 mg/kg TID a |
Epinephrine | 0.01-1 µg/kg/min IV | Inotropy. Increases myocardial oxygen consumption. Dose-dependent effects on SVR |
Norepinephrine | 0.01-1 µg/kg/min IV | Increases SVR |
Vasopressin | 0.0003-0.002 IU/kg/min IV | Probably does not increase PVR (advantage vs. norepinephrine) |
Dobutamine | 5-20 µg/kg/min IV | Increases myocardial oxygen consumption, tachycardia |
Isoproterenol | 0.05-2 µg/kg/min IV | Caution: dose-dependent vasodilation may occur due to unopposed beta 2 -agonism |
Milrinone | 0.375-1.0 µg/kg/min IV | Caution: systemic arterial hypotension Lowers PVR |
Levosimendan | 0.1 µg/kg/min IV | Caution: systemic arterial hypotension Lowers PVR |
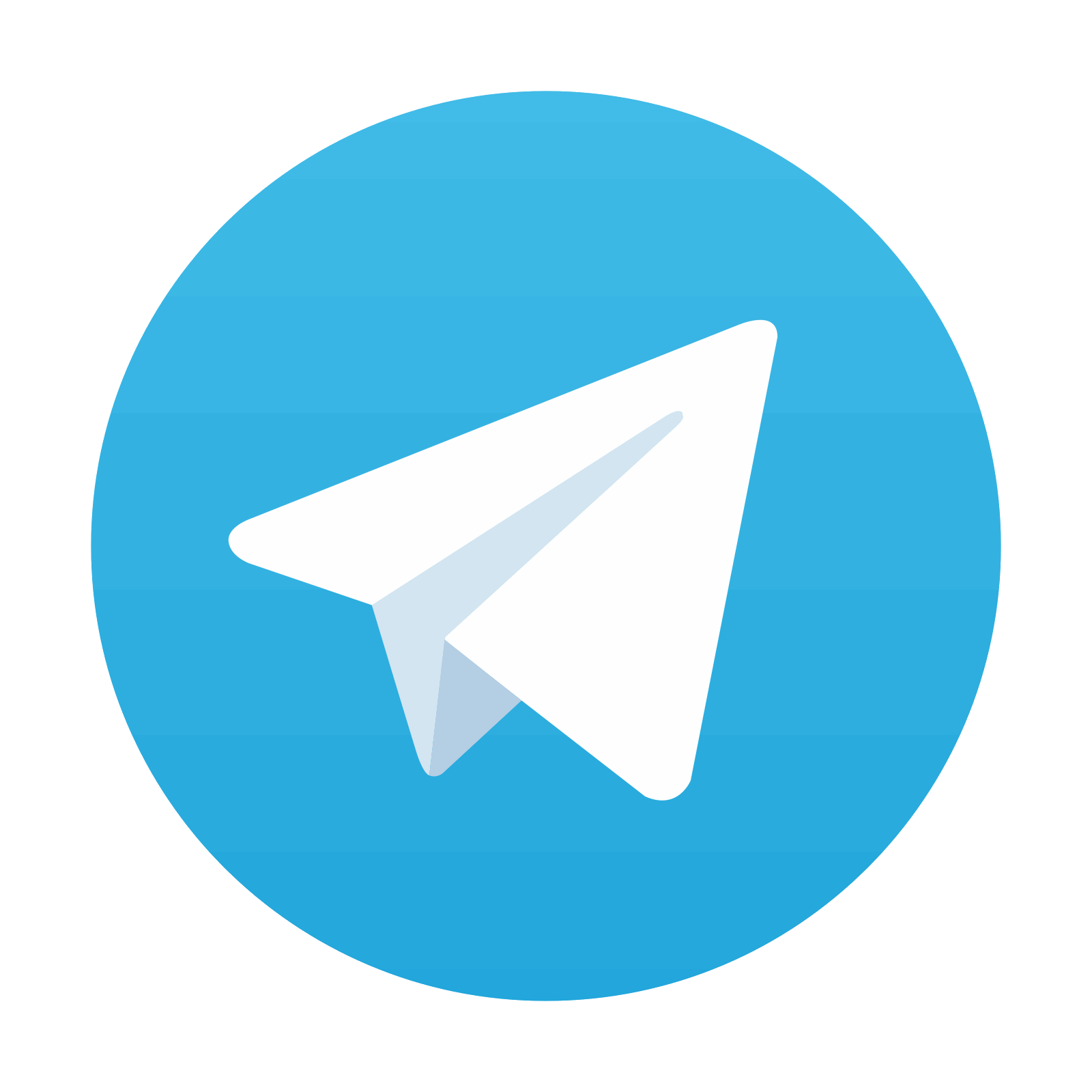
Stay updated, free articles. Join our Telegram channel

Full access? Get Clinical Tree
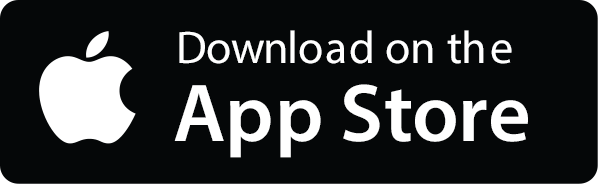
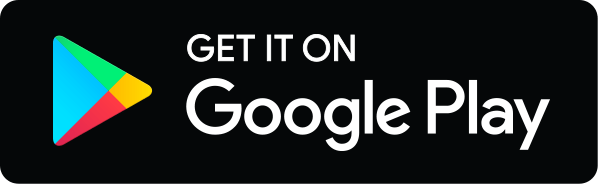
