Magnetic Resonance Imaging
Dietrich Baumgart
Thomas Egelhof
Magnetic resonance (MR) imaging has emerged as a key imaging technique in medical diagnostics in the past 20 years. The dramatic improvement in detailed body organ imaging has been recognized by the Nobel prize granted to Paul Lauterbur and Sir Peter Mansfield in 2003. MR imaging is characterized by its unsurpassed soft tissue contrast with high accuracy. In addition, it allows functional imaging of moving structures, such as the beating heart, or quantification of blood flow. The absence of x-ray exposure is a further substantial advantage and makes MR imaging attractive for repetitive investigations as well as screening purposes. Consequently, the combination of MR imaging (MRI) and MR angiography (MRA) allows simultaneous evaluation of the vessels and of the dependent end-organ function. MR imaging is mainly limited by its costs and availability.
Magnetic Resonance Imaging: Brain, Heart/Lungs, and Abdominal Organs (Brief Overview)
Brain
Magnetic Resonance Imaging in Ischemic and Hemorrhagic Stroke
MRA/MRI imaging allows evaluation of cerebral circulation and brain parenchyma to allow therapeutic decision making in stroke patients.1 Multimodal MRI can be implemented safely in the process of emergency stroke diagnostics and treatment.2 It offers fast and reliable information with respect to the arterial and functional status of the brain. Basis of imaging studies in stroke patients is diffusion-weighted imaging (DWI), which is the most sensitive method for stroke detection, and, combined with perfusion-weighted imaging (PWI), provides information on the functional status of the ischemic brain.1 Additionally MRA then offers information on stroke mechanism and pathophysiology that can guide interventional and medical management.2 Typical protocols of DWI and PWI brain imaging vary according to the individual type of scanners and field strength. On average image acquisition takes <20 to 30 minutes. Figure 3A-1 shows examples of both imaging techniques.
MRA finally provides assessment of intra- and extracranial vascular status in stroke. Extracranial vessels are preferably visualized using 3D gadolinium-contrast techniques. Images are usually acquired in coronal planes with 3D reconstruction.3 On average, image acquisition takes <5 minutes. Alternatively, time-of-flight (TOF) MRA techniques can be used allowing visualization of the cerebral vessels without use of contrast medium. 3D-TOF is preferred over two-dimensional 2D-TOF because of better resolution and lower sensitivity for flow voids. Axial images are reconstructed rendering maximum-intensity projections (MIPs), algorithms to subtract background and to highlight arteries. To assess the target pathology, source images may also be reformatted at different angles and displayed as rotating 3D vascular structures. Figure 3A-2 shows an example of an intracranial TOF.
Intracranial vessels are typically visualized using 2D and 3D TOF imaging sequences. Whereas 2D methods are highly sensitive to slow flow, 3D acquisition allows better sensitivity to a wide range of flows and therefore better visualization of intraluminal flow phenomena. Drawbacks of 3D TOF are longer acquisition time and greater sensitivity to motion artifacts. On average image acquisition takes <5 minutes.
Magnetic Resonance Imaging in Cerebral Angiopathy
There is neuropathological evidence that confluent white matter lesions in the elderly reflect brain damage due to microangiopathy. Cerebral small-vessel alterations are responsible for subcortical lesions associated with cognitive impairments. Small-vessel diseases are classified according to pathology. The most important cerebral microangiopathies are related to long-lasting hypertension. Resulting small-vessel changes may cause ischemic damage to the brain parenchyma and are associated with blood-brain-barrier alterations. Both mechanisms are thought to contribute to the occurrence of white-matter alterations and lacunar infarcts. Modern MR techniques such as fluid-attenuated inversion recovery (FLAIR) and DWI sequences allow noninvasive detection and progress of white-matter lesions (WMLs). There is evidence for an association between long-standing hypertension and WMLs. In the Ansan Study on suboptimally controlled hypertension, despite a multimodal antihypertensive medication, isolated systolic hypertension before medication and untreated systolic and diastolic hypertension were all significantly and independently correlated with the presence and severity of WMLs.4
Magnetic Resonance Imaging in Intracranial Aneurysm
Intracranial aneurysms are common. Autopsy studies have shown that the overall frequency in the general population ranges from 0.8% to 10%.5 Management of intracranial aneurysms has improved significantly during recent years because of major advances in endovascular techniques and microsurgery.5,6 Digital subtraction angiography is still the gold standard for diagnosing cerebral vessel disease, but MRI and MRA are offering complementary data essential for treatment decisions: size and anatomic localization in respect to surrounding tissue or intraluminal thrombus. TOF MRA is basically a fast gradient-echo technique that makes it possible to visualize the arterial lumen. A second technique, contrast-enhanced MRA (CE-MRA), is less affected by flow artifacts and uses gadolinium-containing contrast medium to enhance vessel contrast. Figure 3A-3 shows typical MRA/MRI findings in a patient with intracranial aneurysm.
Heart/Lungs
Cardiac imaging of the beating heart by magnetic resonance technology has long been hampered by the lack of technical solutions to overcome motional artifacts. However, the advent of rapid gradients and fast sequences, as well as multidetection array coils, has now made cardiac magnetic resonance tomography (cMRT) an important tool in the cardiovascular diagnostic workup. Advantages of cMRT include comprehensive and operator-independent visualization of cardiac structures and function free from ionizing radiation. However, as yet, multislice computed tomography (MSCT) and coronary angiography render an up to 10-fold higher resolution of cardiac structures and especially the coronary arteries.
Nevertheless, MRT is best suited to precisely answer a number of questions concerning cardiac anatomy, function, and viability.7 Both acute myocardial infarction and myocardial scar tissue can be detected using T2 weighed sequences and late enhancement techniques. cMRT is the best volumetric technique for quantification of both ventricles and atria. Dissections as well as aneurysms can be diagnosed noninvasively with great precision. Thus far, coronary arteries cannot
be visualized with satisfactory accuracy. However, the refinement of cMRT using navigator sequences as well as the development of intra-arterial contrast media raises hopes for a direct visualization of proximal coronary arteries and stenoses in the future. Figure 3A-4 illustrates typical cardiac MR images.
be visualized with satisfactory accuracy. However, the refinement of cMRT using navigator sequences as well as the development of intra-arterial contrast media raises hopes for a direct visualization of proximal coronary arteries and stenoses in the future. Figure 3A-4 illustrates typical cardiac MR images.
MRT of the lungs has improved over the years but still is lacking in precision compared to CT images. The imaging of the lungs by MRT is complicated by motion artifacts of the heart and respiration. With ECG triggering and breath-hold techniques it is, however, possible to acquire artifact reduced images. Further improvements have been achieved by navigator echo sequences. Nevertheless, CT imaging techniques represent the gold standard in diagnostic imaging of the lungs. In addition, CT imaging techniques are less time consuming compared to MRT.8
On the other hand, MRT images render a much better soft-tissue contrast for diagnostic purposes. At present, the indications for lung or mediastinal MRT are the diagnosis of tumors of the thorax organs and the thorax wall, abscess, retrosternal or mediastinal mass, or thyroid tumors. Although contrast-enhanced x-ray angiography is still considered the gold standard for evaluation of pulmonary vascular pathology, recent years have seen exciting developments in CT and MR angiography of the pulmonary vasculature. With development of parallel imaging and navigator echo techniques, MR image quality has improved substantially. With similar imaging quality, CT techniques still have the advantage of shorter examination times.9
Abdominal Organs
Abdominal ultrasound is the mostly widely available and cost-effective noninvasive imaging technique for diagnosis of abdominal pathologies. However, image quality decreases with increasing body dimensions and the presence of intra-abdominal air.
The acquisition of CT images can be achieved within a short time frame, at reasonable costs, with high spatial resolution, but at the expense of radiation exposure. MR imaging has the advantage of providing the highest accuracy of all available imaging modalities with excellent postprocessing options.3
To diagnose abdominal tumors or metastases and to differentiate malignant from benign lesions requires mostly contrast medium. Body array coils and breathing straps will improve image quality and help to avoid artifacts. Liver, pancreas, kidney, adrenal glands, and retroperitoneal space are the preferably targeted sites for MRT imaging. With maximum-intensity projections it is possible to acquire accurate three-dimensional noninvasive images of the biliary and pancreatic ducts. These techniques represent an adequate alternative to conventional endoscopic retrograde cholangiopancreatographic (ERCP) imaging.3
Magnetic Resonance Panvascular Angiography
Technical Aspects
Basically there are native MRA techniques and MRA techniques using contrast agents for the visualization of vascular structures.
The inflow or time of flight, TOF techniques do not use any contrast medium. In short T1 gradient echo sequences, the relaxation of resting tissue is low associated with a decreased signal whereas the flowing blood provides high signals. This method of contrast enhancement is, however, dependent on the fact that flow is perpendicular to the scanning plane and that flow is sufficiently fast to refresh spins and to enhance the signal. The contrast is further influenced by the flip angle, which can be modified (for review, see reference 3).
The technique is best suited for small vessels in stationary organs. The acquisition time is relatively long, approximately 5 to 9 minutes, making this technique suitable primarily for brain vessel imaging. Turbulent flow reduces the signal, accounting for signal attenuation or signal voids in the vicinity of stenoses. Consequently, stenoses might be overestimated with this imaging technique. TOF angiography can be used with two- and three-dimensional sequences (for review, see reference 9).
Phase-contrast angiography relies on a unique pixel coding based on frequency and phase encoding and does not utilize any contrast agents. Reversing the polarity of the gradient will rephrase the spins of the stationary tissues. In contrast, spins of the moving blood experience a phase delay in proportion to flow velocity allowing reliable flow velocity measurements. Phase-contrast angiography is mostly used to image brain veins. Flow measurements are primarily performed during cardiac imaging. The technique is also suited as a fast “angio-localizer” for subsequent contrast enhanced angiography.
MR angiography of the thorax, abdomen, or iliac vasculature cannot reliably be performed with native MR-angiographic techniques because the acquisition times are too long and breathing and pulsation artifacts will disturb image quality. Only fast 3D-gradient echo sequences with short TR and TE can achieve the necessary acquisition times of 15 to 30 seconds. The strong T1 weight allows imaging only of tissue with a very short T1-relaxation time. Fat tissue has the shortest T1-relaxation time (150 msec). With the help of intravenous contrast medium, the T1-relaxation time of blood can be reduced to 50 msec. Hence, blood gives the brightest signal as long as the contrast bolus is not diluted. Therefore, a successful angiographic imaging can only be performed shortly after the contrast bolus was injected. As the acquisition times are short, measurements should not be performed too early before the contrast bolus has reached the region of interest. Optimal timing is thus crucial for perfect image acquisition. In clinical practice, a small test bolus is given prior to the actual MR angiography and a dynamic sequence registers the advent of the contrast agent in the region of interest. Subsequently,
acquisition of the angiography can be timed exactly to the bolus appearance (for review, see reference 9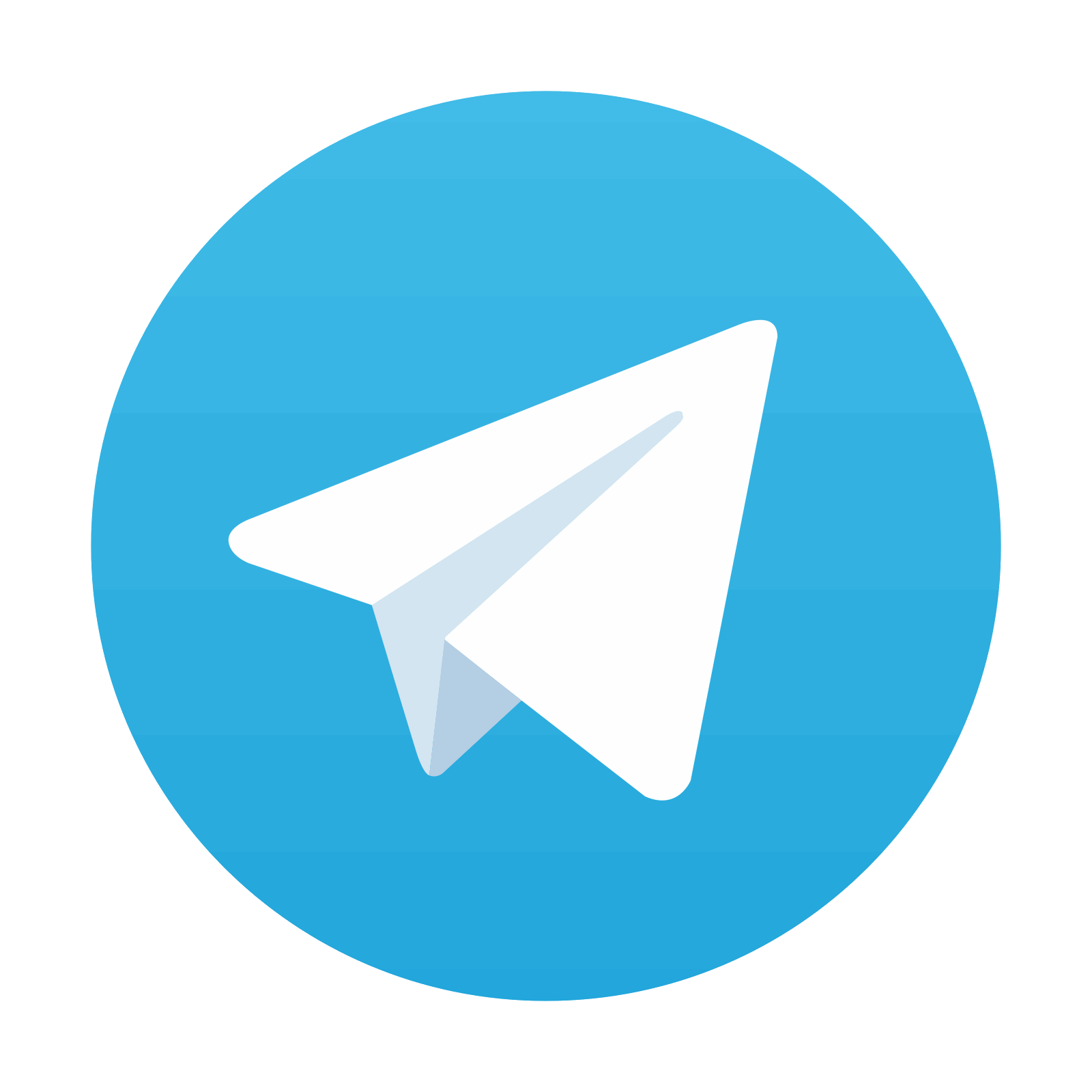
acquisition of the angiography can be timed exactly to the bolus appearance (for review, see reference 9
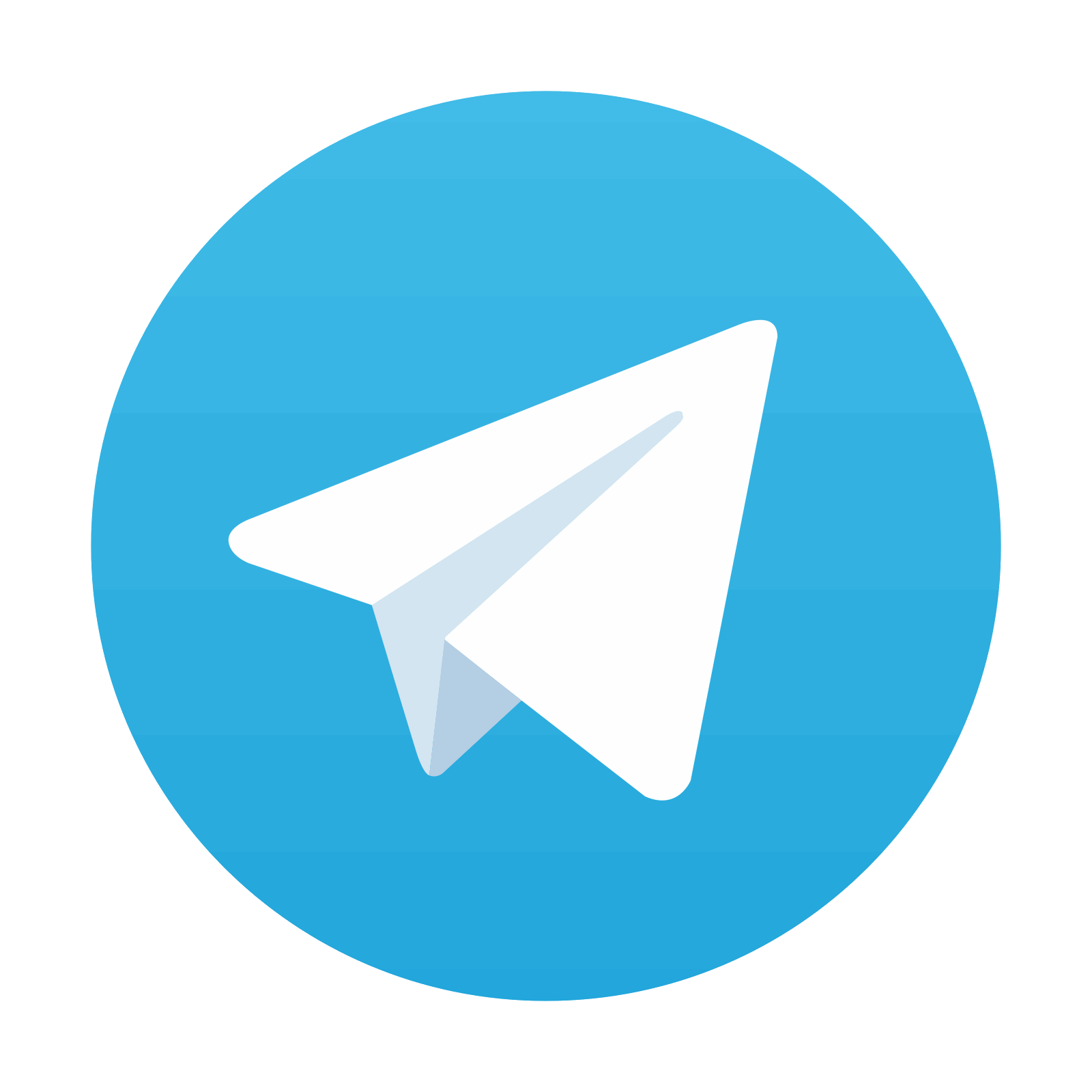
Stay updated, free articles. Join our Telegram channel

Full access? Get Clinical Tree
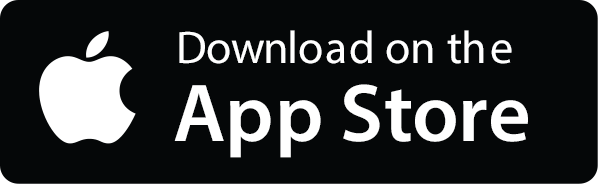
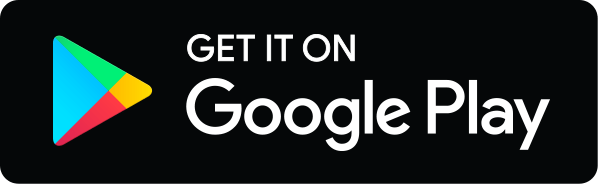
