Magnetic and Robotic Navigation
Bruce D. Lindsay
Mitchell Faddis
The advent of ablation procedures for supraventricular and ventricular arrhythmias accelerated the development of technology to facilitate catheter manipulation and imaging. Deflectable catheters, electroanatomic imaging, and intracardiac echocardiography serve as examples of this effort. The early work on catheter-based ablation of arrhythmias focused on elimination of accessory pathways, dual AV nodal physiology, or typical isthmus-dependent right atrial flutter. These arrhythmias demand significant skills for catheter manipulation, but the ability to direct a catheter to the target rarely proves difficult for an experienced electrophysiologist.
As electrophysiologists began to ablate more complex arrhythmias, the limitations of commercially approved catheter designs placed greater demands on the skills of the electrophysiologists. Catheter deflection occurs in a fixed radius in a single plane restricting the freedom of movement. Side-to-side catheter motion is determined by transmission of torque, which may be limited by tortuosity of vessels and the orientation of the catheter within the cardiac chamber. In addition, stable endocardial contact with the catheter may be compromised by cardiac or respiratory movement. These limitations are particularly important for ablation of complex arrhythmias such as ventricular tachycardia, atypical atrial flutter, or atrial fibrillation, which depend on accurate control of the catheter for focal or linear lesions. Radiation exposure to patients and physicians and the orthopedic effects of prolonged ablation procedures raise additional concerns about safety and long-term consequences. Advances in technology offer potential solutions to many of these issues. The objectives of developing new technologies to facilitate complex ablation procedures include:
Precise and stable catheter navigation.
3-D imaging systems to track the position of the catheter and guide effective ablation of tissue.
Improved delivery of energy for more effective delivery of ablative lesions.
Reduced radiation exposure.
Shorter procedures.
Cost effectiveness.
Although new technologies generally increase the cost of a procedure when they are introduced, the costs may be justified if they improve outcomes. The concept of remote catheter navigation is appealing for the operator because these systems may reduce radiation exposure to the physician and the risk of developing orthopedic problems related to prolonged use of protective lead aprons during protracted cases. They also may facilitate analysis of intracardiac electrograms and 3-D images because the catheter navigation and analysis can be performed from a work station where the operator is seated.
Two technologies developed to meet these objectives include the magnetic navigation system designed by Stereotaxis, Inc., and a robotic-controlled catheter system manufactured by Hansen Medical. While neither is approved specifically for ablation of atrial fibrillation at this point, the impetus to develop these technologies is to use them for complex ablation procedures. It is likely that over the next 5 years the potential utility of these remote navigation systems will be determined. But at the present time, prospective studies are not available to compare these technologies or to demonstrate definitively that either system shortens procedure time, improves outcomes of ablation, or improves the safety profile of these and other complex ablation procedures. Nonetheless, preliminary evidence suggests that these goals will be achieved.

Principles of Magnetic Navigation
The fundamental concept underlying magnetic navigation is that the catheter aligns itself with a magnetic field vector. This principle can be illustrated by holding a compass in one hand and a large magnet in the other. As the magnet is moved toward the compass, the needle of the compass will align itself with the magnet. The system does not pull the catheter forward. In order to advance or retract the catheter the operator can use a mechanical device that is attached to the catheter near the vascular access site and is controlled by a joystick at the work station.
The magnetic navigation system developed by Stereotaxis, Inc., uses two large fixed magnets located on either side of the patient that rotate to generate a composite field vector. The computer work station allows the operator to select the desired field vectors. The maximum field strength is in the range of 0.08 tesla, which is less than one tenth of an MRI scanner. Once the vector is selected, the operator advances the catheter, which tends to move along the line of the field vector to the target.
As shown in Figure 7.1, the catheter deflection depends on the strength of the magnetic field, the angle of the field vector relative to the shaft of the catheter, the length of the magnet, and the ferromagnetic materials that comprise the magnet. The maximal force on the catheter occurs when the field is perpendicular to the catheter, and the force decreases to zero when the catheter is parallel to the field vector. Accordingly, the catheter aligns with the magnetic field because this is the path of least resistance. In order to optimize field strength and maintain flexibility, the catheter employs three small magnets in a linear array.
Early Development
The concept of magnetic navigation had its roots in neurosurgery. The initial work by Rogers Ritter examined the possibility that a flexible implement could be introduced through the skull via a burr hole and advanced around critical structures to a target deep in the brain using a magnetic field to steer the implement (1). The potential advantage was that by changing the magnetic field vector, the implement could
be advanced without following a straight line, thereby avoiding damage to selected tissue within the brain. The course by which the implement was advanced depended on registering fiducial points attached to the skull (radio-opaque screws) to a CT scan. The first neurosurgical clinical work was centered at Washington University School of Medicine. The authors were approached by Stereotaxis, Inc., to determine whether this technology had implications for cardiology, which marked the onset of a second line of investigation for the company that began in 1998.
be advanced without following a straight line, thereby avoiding damage to selected tissue within the brain. The course by which the implement was advanced depended on registering fiducial points attached to the skull (radio-opaque screws) to a CT scan. The first neurosurgical clinical work was centered at Washington University School of Medicine. The authors were approached by Stereotaxis, Inc., to determine whether this technology had implications for cardiology, which marked the onset of a second line of investigation for the company that began in 1998.
There were obvious challenges to development of magnetic navigation in the heart as opposed to the brain. The x-ray system required flat plate technology before this was commercially available, and the system that was used in the prototype navigation system provided rudimentary images. While it sufficed to record metallic screws in the heart, it was inadequate for cardiac navigation.
The first animal experiments were conducted with radio-opaque plaques sewn to the epicardium so that targets could be visualized to assess navigation. Secondly, there were no catheters designed for magnetic navigation. Early work explored the feasibility of using catheters with central lumens and stylets, not unlike pacemaker leads, but more consistent results were obtained with leads resembling conventional ablation catheters. The main difference was that the catheters were much softer and more flexible because deflection mechanisms were not required. Another area of development was the magnet system. The first prototype, which was used for the initial cardiac experiments in animals, is shown in Figure 7.2. It was developed for neurosurgery and consisted of an orthogonal array of electromagnets that were cooled by liquid nitrogen. The bore of the magnet was small, which limited the size of animals used for magnetic cardiac navigation. The expense and engineering support required for this system would be challenging for commercial release. The software used to direct the magnetic field was not optimal for choosing a composite field vector and there were delays of 10 to 15 seconds whenever the field vector was changed.
Although the initial prototype was used successfully for proof of concept in animals, a larger magnet was required to encompass the torso of a human and higher-fidelity fluoroscopy was mandatory. The second prototype, shown in Figure 7.3, met these requirements, but it had other characteristics that were not endearing to the investigators. The electromagnets were cooled by liquid helium. The helium was compressed by pumps that created substantial background noise, which made communication difficult. The patient was inserted into the donut of the magnet, which was prone to cause claustrophobia, imposed an additional constraint on monitoring and communication, and limited the size of the patient. Nonetheless, the initial human trials were conducted successfully without any significant complications.
Figure 7.4 illustrates the third design, which replaced electromagnets and the need for helium to cool the system. This design, which is currently in use (Niobe II, Stereotaxis, Inc.), employs large fixed magnets, housed on either side of the patient, which the operator controls by a computer interface. This system functions silently and can adjust the field vector in 1 to 2 seconds. There is no barrier to communication. The system accommodates relatively large patients without inducing claustrophobia, but patients who are broad shouldered or exceed approximately 280 lbs may be not fit well between the magnets. There have been substantial improvements in catheter and software design to improve catheter navigation. Figure 7.5 shows the workstation where the physician sits while operating the system.
The initial animal studies tested the feasibility of magnetic navigation and identified areas for further development (2). Three questions were evaluated regarding the safety and efficacy of the catheter. First, would electrical interference induced by the magnetic field limit the fidelity of recording standard surface ECGs or intracardiac electrograms? Second, would the magnetic catheter apply excessive force that could result in injury or perforation? Third, would the catheter meet demands for accurate navigation, intracardiac recording, and radiofrequency ablation of cardiac tissue in vivo?
![]() Figure 7.4. The Niobe II system was approved for commercial release. It uses fixed magnets that are housed within the encasements positioned on either side of the patient. See color insert 1. |
![]() Figure 7.5. A: Computer interface with x-ray images, the CARTO electroanatomic image, and the Stereotaxis images shown. The control box for the catheter advancer system is designated by an arrow. B: An updated display, which is controlled by a single keyboard and mouse, integrates x-ray images, the CARTO image, and the Stereotaxis image on a single large plasma screen. See color insert 1. |
The results of initial studies showed that the magnetic field induced an electrical potential that was superimposed on the ECG recorded on the body surface. The resultant distortion of the surface ECG affected the T-wave predominantly. It is attributed to a potential generated by blood flow in the aorta by the magnetic field. The temporal distribution of the interfering signal component does not compromise analysis of the p-wave or QRS morphology. Intracardiac recordings with conventional filter settings were qualitatively unaffected by the magnetic field. As shown in Figure 7.6, intracardiac signals could be recorded without substantial degradation of the signal.
The maximal endocardial contact force exerted by the magnetic catheter was determined to be less than the maximal force applied by a conventional catheter, and attempts to intentionally perforate the heart with the magnetic catheter did not result in endocardial injury or perforation. The third objective of the study confirmed accurate navigation of the catheter to all four cardiac chambers. In addition, navigation to the pulmonary veins was achieved by transseptal and retrograde approaches.
From its inception, the ultimate goal of magnetic navigation in the heart was to facilitate ablation of atrial fibrillation. A second series of animal experiments focused on electrical isolation of pulmonary veins in seven canines using an ostial segmental ablation strategy (3). A remote control mechanism to advance or retract the catheter, which is referred to as the catheter advancer system, is illustrated in Figure 7.7. The
procedures were performed using an irrigated catheter. All targeted pulmonary veins were successfully isolated without evidence of stenosis during long-term follow-up. Figure 7.8 demonstrates the appearance of the pulmonary vein ostium 3 months after ablation, and Figure 7.9 shows the histological appearance of transmural ablation lesions when the animal was sacrificed 3 months later. The study concluded that electrical isolation of the pulmonary veins is feasible with a magnetically guided irrigated catheter. The irrigation technology induced transmural lesions without charring or other complications.
procedures were performed using an irrigated catheter. All targeted pulmonary veins were successfully isolated without evidence of stenosis during long-term follow-up. Figure 7.8 demonstrates the appearance of the pulmonary vein ostium 3 months after ablation, and Figure 7.9 shows the histological appearance of transmural ablation lesions when the animal was sacrificed 3 months later. The study concluded that electrical isolation of the pulmonary veins is feasible with a magnetically guided irrigated catheter. The irrigation technology induced transmural lesions without charring or other complications.
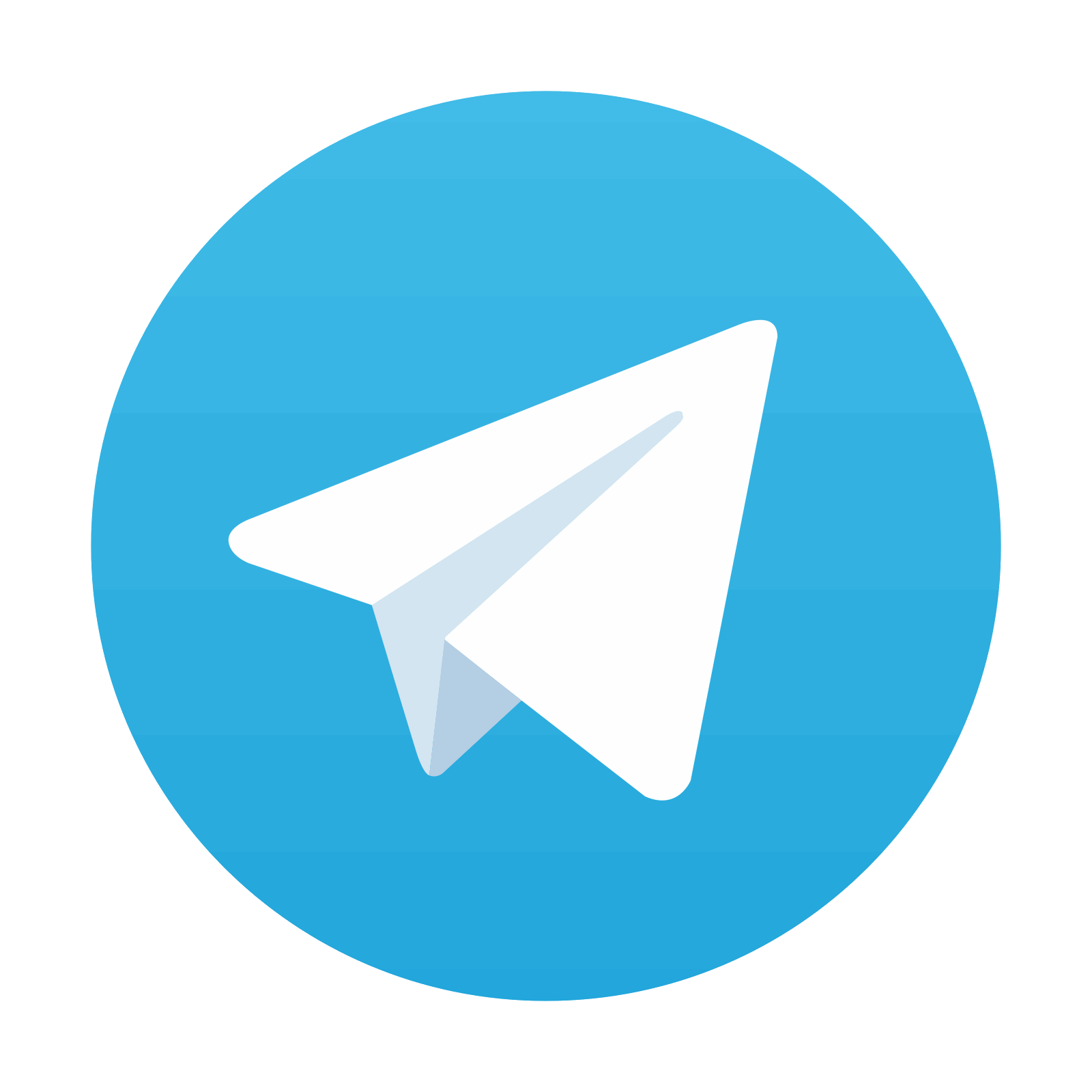
Stay updated, free articles. Join our Telegram channel

Full access? Get Clinical Tree
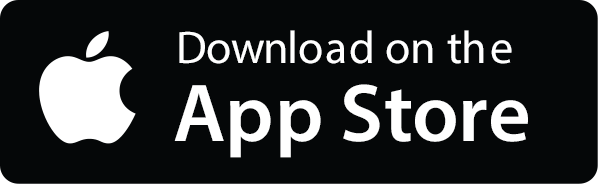
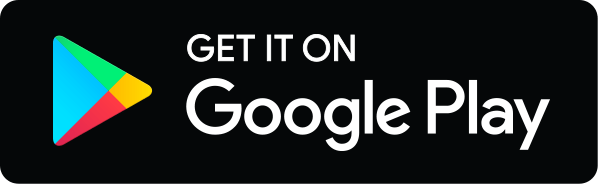