Abstract
Organized atrial tachycardias (ATs) are broadly categorized as either focal (centrifugal activation originating from a discrete site that incorporates automaticity, triggered activity, and microreentrant mechanisms) or macroreentrant (a relatively large reentrant circuit around a central obstacle). Depending on whether or not the cavotricuspid isthmus (CTI) is critical to the reentry circuit, macroreentrant ATs (MRATs) are divided into two groups: “CTI-dependent” MRAT or “non-CTI-dependent” MRAT.
CTI-dependent MRATs include typical atrial flutter, lower loop reentry, and intraisthmus reentry. Non-CTI-dependent MRAT comprises a heterogeneous group of ATs related to different anatomical and electrophysiological substrates. These arrhythmias are frequently associated with structural heart disease, congenital cardiac defects, previous cardiac surgical procedures, or surgical or catheter ablation procedures for atrial fibrillation.
Medical management of MRAT involves pharmacological rate and rhythm control strategies, as well as long-term anticoagulation for stroke prevention. The choice of rate versus rhythm control strategies typically considers several factors, including severity of symptoms, response to rate-controlling medications, cardiac function, and associated noncardiac diseases. With recent advances in the technology to visualize and modify the arrhythmia substrate, ablation of MRAT has become increasingly successful; nonetheless, MRAT can have complex circuits that demand a thorough knowledge of atrial anatomy and a great level of experience to correlate activation patterns with anatomical landmarks.
Keywords
macroreentrant atrial tachycardia, lower loop reentry, upper loop reentry, dual-loop reentry, perimitral macroreentry
Outline
Pathophysiology, 375
Macroreentry, 375
Dual-Loop Reentry, 375
Localized Reentry, 375
Cavotricuspid Isthmus-Dependent Right Atrial Macroreentry, 377
Non-CTI-Dependent Right Atrial Macroreentry, 378
Left Atrial Macroreentry, 378
Epidemiology, 380
Clinical Presentation, 381
Initial Evaluation, 381
Principles of Management, 381
Electrocardiographic Features, 381
Electrophysiological Testing, 382
Induction of Tachycardia, 382
Tachycardia Features, 382
Diagnostic Maneuvers During Tachycardia, 383
Mapping, 388
Electroanatomic Mapping, 388
Entrainment Mapping, 392
Noncontact Mapping, 395
Practical Approach to Mapping Macroreentrant Atrial Tachycardia, 396
Ablation, 397
Target of Ablation, 397
Ablation Technique, 399
Endpoints of Ablation, 400
Ablation of Perimitral Macroreentrant Atrial Tachycardia, 401
Outcome, 404
Pathophysiology
Organized atrial tachycardias (ATs) are broadly categorized as focal (centrifugal activation originating from a discrete site that incorporates automaticity, triggered activity, and microreentrant mechanisms) or macroreentrant (a relatively large reentrant circuit around a central obstacle). Depending on whether the cavotricuspid isthmus (CTI) is critical to the reentry circuit, macroreentrant atrial tachycardias (MRATs) are divided into two groups: “CTI-dependent” MRAT or “non-CTI-dependent” MRAT ( see Table 11.1 ).
CTI-dependent MRATs include typical atrial flutter (AFL), lower loop reentry, and intra-isthmus reentry. The term “ atrial flutter ” has traditionally been used to refer to a continuously waving pattern on the electrocardiogram (ECG), without an isoelectric baseline in at least one lead, whatever the cycle length (CL). “Typical AF” is reserved for a macroreentrant circuit with the activation wavefront rotating clockwise or counterclockwise around the tricuspid annulus and using the CTI as an essential part of the reentry circuit. “Atypical AFL” is simply a descriptive term for an AT with an ECG pattern of continuous undulation of the atrial complex, different from that in typical AFL. However, the term “atypical AFL” introduces unnecessary confusion, and a mechanistic description of the AT circuit in relation to atrial anatomy is preferred (e.g., perimitral macroreentry, lesional right atrial [RA] macroreentry).
Macroreentry
The mechanism of MRAT is reentrant activation around a large central obstacle, generally several centimeters in diameter, in at least one of its dimensions. The central obstacle can consist of normal or abnormal structures, and can be fixed, functional, or a combination of both. There is no single point of origin of activation, and atrial tissues outside the circuit are activated from various parts of the circuit. The CL of MRAT can vary widely and is not a reliable predictor of the mechanism. When the mechanism of MRAT can be further elucidated through different mapping techniques, description of MRAT mechanisms must be made in relation to atrial anatomy, including a detailed description of the obstacles or boundaries of the circuit and the critical isthmuses.
Dual-Loop Reentry
Although MRAT can manifest as an isolated arrhythmia (single-loop reentry) , it can also occur in conjunction with other reentry circuits (e.g., incisional MRAT and typical AFL). When two atrial macroreentrant circuits coexist and use neighboring anatomical structures, they create the so-called dual-loop reentry. The multiple loops can be engaged simultaneously or encountered sequentially. Not uncommonly, ablation of one tachycardia results in transition to the other; hence, ablation of both circuits is necessary for clinical success.
A figure-eight reentry is a specific type of dual-loop reentry involving two simultaneously coexisting loops with opposite directions of propagation and sharing a common segment of unidirectional activation.
Localized Reentry
The term localized reentry has been used to refer to a reentrant circuit that is localized to a small area (covering a surface diameter of <3 cm) and does not have a central obstacle. These circuits typically form in close proximity to scars or prior ablation lines, where severe local conduction abnormalities allow for very slow conduction to support perpetuation reentry in such a small anatomical path. Typically, low-amplitude, long, fractionated electrograms occupying more than 70% of the tachycardia cycle length (TCL) can be recorded in a small region of the atrium, from which activation spreads out centrifugally to activate the rest of the atrium ( Fig. 13.1 ). Hence, these localized reentry circuits can mimic focal AT, especially if a limited number of endocardial recordings are collected. Although localized reentry circuits are much smaller than macroreentry circuits, they are still significantly larger than focal “microreentrant” AT, whose site of origin cannot be mapped spatially beyond a single point or a few adjacent points with the resolution of a standard 4-mm-tip catheter.

Cavotricuspid Isthmus-Dependent Right Atrial Macroreentry
Lower loop reentry and intra-isthmus reentry are macroreentrant circuits that are confined to the RA and incorporate the CTI as a critical part of the circuit. However, in contrast to typical AFL, the circuit is not peritricuspid ( Fig. 13.2 ). Nevertheless, because the CTI is still a necessary part of the circuit, these arrhythmias are amenable to CTI ablation, as is true for patients with typical AFL.

Lower Loop Reentry
Lower loop reentry is a form of CTI-dependent MRAT with a reentrant circuit around the inferior vena cava (IVC); therefore it is confined to the lower part of the RA (see Fig. 13.2 ). The activation wavefront can rotate around the IVC in a counterclockwise (i.e., the impulse travels within the CTI in a medial-to-lateral direction) or clockwise fashion. A breakdown in the inferoposterior boundaries of the CTI produced by the eustachian ridge and lower crista terminalis causes the circuit to revolve around the IVC (instead of around the tricuspid annulus), across the eustachian ridge, and through the crista terminalis, with slow conduction because of transverse activation through that structure. Alternatively, the circuit can exit at the apex of the triangle of Koch and come behind the eustachian ridge to break through across the crista terminalis behind the IVC and then return to the CTI.
Lower loop reentry often coexists with counterclockwise or clockwise typical AFL and is usually transient and terminates by itself or converts spontaneously into AFL or atrial fibrillation (AF).
Intra-Isthmus Reentry
Intra-isthmus reentry is a reentrant circuit usually occurring within the region bounded by the medial CTI and coronary sinus ostium (CS os) (see Fig. 13.2 ). Circuits in the mid and lateral portion of the CTI can also occur but are less common.
This arrhythmia can be sustained and usually occurs in patients who have undergone prior, and often extensive, ablation at the CTI. Catheter ablation can result in islands of scarred areas and slowly conducting channels that can potentially provide the substrate for this arrhythmia. In one report, intra-isthmus reentry accounted for 21% of cases of “recurrent” AFL following prior successful CTI ablation. Of note, persistence of bidirectional CTI block following ablation of typical AFL does not exclude the possibility of intra-isthmus reentry, since the reentry circuit can exist medial to the line of CTI block. The reentry circuit can be quite small; fractionated potentials spanning one- to two-thirds of the TCL are typically recorded within the CTI.
The atrial activation sequence around the tricuspid annulus electrograms can exhibit spontaneous or pacing-induced shifts from a counterclockwise to clockwise or fusion patterns. In addition, entrainment mapping from the lateral CTI demonstrates a postpacing interval (PPI) longer than the TCL, a finding indicating that the lateral CTI is not part of the reentrant circuit. On the other hand, pacing from the region of the medial CTI or CS os demonstrates concealed entrainment with a PPI equal to the TCL. Linear ablation across the medial CTI, usually at the site of a very prolonged electrogram, can eliminate the tachycardia.
Non-CTI-Dependent Right Atrial Macroreentry
Incisional Right Atrial Macroreentry
Incisional or scar-mediated RA macroreentry circuits are the most common form of non-CTI-dependent RA MRATs. The incision on the RA free wall is a routine access site for corrective surgery for congenital or acquired heart disease. The atriotomy scar, suture lines, and cannulation sites form fixed obstacles that can potentially promote reentry by providing multiple protected isthmuses along with natural conduction barriers (such as valve annuli and caval ostia) and regions of atrial scarring caused by the underlying heart disease. In its simplest form, incisional RA MRAT uses a circuit that rotates around the atriotomy scar, with the lower turning point located between the end of the scar and the IVC, and the upper turning point located between the upper end of the scar or the superior vena cava (SVC). Other obstacles can also be included in the reentry circuit, such as anatomical structures located in the vicinity of the scar (e.g., SVC or IVC) or functional obstacles (anisotropic conduction delay or block).
These patients often exhibit multiple clinical or inducible tachycardias, particularly typical AFL, indicating the complexity of the atrial substrate. Importantly, the atriotomy scar in the lateral or posterolateral RA forms a fixed posterior barrier to conduction in the superoinferior direction between the venae cavae, and a lateral boundary necessary for the development of a peritricuspid macroreentry (typical AFL) by preventing short-circuiting of the tricuspid annulus via the posterior atrium. This explains why typical AFL is the most common AT in this patient population, accounting for more than 70% of all MRATs. Other RA MRATs involving free-wall atriotomy become progressively more common with more extensive atrial incisions.
RA free-wall atriotomy is also widely used to access the left atrium (LA) for surgical replacement of the mitral valve. In fact, RA MRATs are more common than LA macroreentry in this group of patients. Other approaches for mitral valve surgeries involve incisions through the interatrial (Waterston) groove, transseptal incisions, and the superior transseptal approach. The latter surgical approach involves an extensive series of incisions in the lateral and superior RA, the interatrial septum, and the LA roof. Consequently, the superior transseptal approach has been associated with a higher risk of RA MRATs.
Patients with congenital heart disease have a high prevalence of MRAT, particularly after they have undergone reparative or palliative surgical procedures. For MRATs in adults with repaired congenital heart disease, three RA circuits are generally identified: (1) lateral wall circuits with reentry around or related to the lateral atriotomy scar; (2) septal circuits with reentry around an atrial septal prosthetic patch (when present); and (3) typical AFL circuits using the CTI. These arrhythmias are discussed separately in Chapter 14 .
Right Atrial Macroreentry in the Absence of Previous Surgery
Rarely, RA MRATs have been observed in patients without prior surgery in whom the central obstacle is a large area of abnormal atrial myocardium and electrically silent zones, indicating scarring. The mechanism of atrial scarring remains uncertain, but it has been suggested that these low-voltage areas may reflect areas of fibrosis potentially related to inflammatory, infiltrative, or ischemic processes. These patients frequently exhibit multiple clinical or inducible tachycardias. The most common circuit involves macroreentry around the RA free wall, but the vast majority of these patients also have clinical or inducible typical AFL, and many had prior CTI ablation. The electrically silent area forms a fixed posterior conduction barrier, which likely facilitates the development of peritricuspid reentry.
Upper Loop Reentry
This type of MRAT involves the upper portion of the RA, with transverse conduction across the crista terminalis and wavefront collision occurring at a lower part of the RA or within the CTI. The central obstacle of the circuit is composed of the crista terminalis (functional) and the SVC (fixed). The impulse rotating in the circuit can be in a counterclockwise or clockwise direction. The CTI is not an intrinsic part of the reentrant circuit. The lower turnaround point for this circuit is by conduction through a gap in functional block in the crista terminalis. Ablation of this gap can abolish the arrhythmia. Upper loop reentry can exist as a single loop or as a part of a dual-loop (figure-eight) reentry with lower-loop or with free-wall circuits.
Left Atrial Macroreentry
LA MRATs are frequently related to or coexist with AF. Areas of scar and low-voltage zones, associated with local conduction slowing or block, frequently promote the development of MRAT. Scar areas can be a result of previous atriotomy, surgical or catheter ablation, or can be spontaneous, producing electrically silent areas in the atrium.
Incisional Left Atrial Macroreentry
A variety of incisional LA macroreentry circuits have been described in patients with prior cardiac surgery involving the LA or atrial septum. The atriotomy scar forms a fixed obstacle that, in combination with the often intrinsically diseased atrial myocardium (providing functional obstacles) and normal structural obstacles in the vicinity of atrial incisions, can provide the substrate for macroreentry. Following mitral valve surgery, low-voltage areas are often detected anterior to the right pulmonary veins (RPVs), corresponding to LA incisions.
Left Atrial Macroreentry Postablation of Atrial Fibrillation
LA MRAT is a frequent complication of surgical and catheter-based therapies of AF. The incidence and nature of ATs developing post AF ablation are, in large part, determined by the type of ablation performed and by the presence of abnormal LA substrate ( see Chapter 15 ). The incidence seems to be lower following segmental ostial pulmonary vein (PV) isolation than circumferential PV isolation and higher following circumferential or linear LA ablation (more than 30%). The incidence of MRAT is much higher (more than 50%) for approaches of catheter ablation incorporating extensive lesions in the LA to terminate persistent AF. Linear ablation lesions, together with anatomical structures and intrinsically abnormal adjacent atrial myocardium, provide an ideal substrate for reentry. Gaps in the ablation lines further increase the chance for reentry by creating a region of slow conduction (see Fig. 13.1 ).
The most common form of macroreentry occurring after AF ablation is perimitral MRAT (accounting for approximately 40% of all MRATs). Macroreentrant circuits traversing the LA roof account for approximately 20% ( see Fig. 15.60 ). Less common sites of macroreentry involve the right or left PVs, LA septum, and the base of the LA appendage ( Fig. 13.3 ). Not infrequently, multiple macroreentrant circuits and multiple-loop reentrant circuits are encountered. In addition, localized reentrant circuits are not uncommon, and they usually arise from the vicinity of the isolated PVs or linear lesions.

LA MRATs comprise the majority of arrhythmias developing following surgical ablation of AF. Perimitral macroreentry is the most common, but macroreentry circuits incorporating the LA roof, septum, and posterior wall, and circuits around the PVs have also been observed. Localized reentrant ATs comprise a sizable minority of the cases.
Perimitral Left Atrial Macroreentry
This circuit involves reentry around the mitral annulus in a counterclockwise or clockwise fashion ( Fig. 13.4 ). The mitral annulus forms the anterior boundary of the reentry circuit, whereas the posterior boundary is formed by a low-voltage zone or scar in the posterior wall of the LA.

Perimitral MRAT is more common in patients with structural heart disease; however, it has been described in patients without obvious structural heart disease. In the latter group, voltage mapping often shows scar or low-voltage areas on the posterior wall of the LA forming the posterior boundary of the reentry circuit. As noted, perimitral macroreentry is the most common MRAT in patients with prior LA ablation procedures for AF, particularly when incomplete linear ablation across the lateral mitral isthmus is performed.
Circuits Involving the Pulmonary Veins
Various reentrant circuits involve the PVs, especially in patients with AF or mitral valve disease and those with prior catheter ablation of AF (particularly with linear LA ablation lesions), including circuits around one or more PVs (see Fig. 13.3 ) and posterior scar or low-voltage areas. PV-LA conduction recovery is frequently a critical element in these arrhythmias.
Left Septal Circuits
Left septal circuits occur primarily in patients with previous heart surgery, particularly after mitral valve surgery requiring septal incision, and those with prior AF ablation. Rarely, MRATs involving the LA septum have been reported in the absence of prior cardiac surgery. These circuits involve the septum primum, which acts as a central obstacle for the reentrant circuit. The right PV ostia serve as its posterior boundary, whereas the mitral annulus serves as the anterior boundary. Atrial dilation and concomitant antiarrhythmic drug therapy also appear to play a role by the prolongation of left intraatrial conduction, which then allows stable macroreentry circuits to persist. In patients with a history of surgery for atrial septal defects, scars or the patch on the septum can serve as the anatomical substrate of left septal circuits.
Left Atrial Macroreentry in the Absence of Previous Surgery or Ablation
LA circuits can be found in patients without a history of atriotomy or prior ablation. Electroanatomic maps in these patients often show electrically silent (low-voltage or scar) areas in the LA, which act as a central obstacle or barrier in the circuit. The pathogenesis of these areas with no electrical signals is not well established. Potential causes include volume and pressure overload (mitral valve disease, hypertension, heart failure), ischemia (atrial branch occlusion), postinflammation scarring (after myocarditis), atrial amyloidosis, atrial dysplasia, and tachycardia-related structural remodeling. These conditions are frequently associated with diffuse or patchy areas of scarring, which are typically located at the posterior wall (45%), roof (28%), or anteroseptal region (27%) of the LA. The macroreentrant circuits in these patients show considerable anatomical variability and frequently involve multiple simultaneous loops (dual- or triple-loop reentry, or figure-eight reentry).
Epidemiology
MRAT comprises a heterogeneous group of ATs related to different anatomical and electrophysiological (EP) substrates. These arrhythmias are frequently associated with structural heart disease, congenital cardiac defects, previous cardiac surgical procedures, or surgical or catheter ablation procedures for AF. However, MRAT occasionally occurs in a patient with no apparent structural heart disease. Often, these arrhythmias coexist with AF. Not infrequently, these patients experience sinus node dysfunction (SND), which can be symptomatic or hinder pharmacological therapy of the tachycardia.
Although its exact prevalence among cardiac arrhythmias is difficult to establish clinically, MRAT is not a rare arrhythmia. In fact, the incidence of MRAT has been increasing, likely due to aging of the general population, which implies progressive alteration of the atrial electrical properties with development of an arrhythmogenic substrate, and also due to the rapid growth of surgical and catheter-based ablation procedures for treatment of AF.
Clinical Presentation
MRATs are typically persistent. As with AF and typical AFL, patients may present with symptoms related to rapid ventricular response, loss of atrioventricular (AV) synchrony, tachycardia-induced cardiomyopathy, or deterioration of preexisting cardiac disease. In addition, typical AFL and MRAT can contribute to systemic thromboembolic complications and stroke.
The clinical presentation of MRAT can vary widely, from completely asymptomatic to severe hemodynamic compromise. Most patients present with a spectrum of symptoms including palpitations, lightheadedness, fatigue, reduced activity tolerance, and dyspnea. Severe heart failure or acute coronary syndrome can occur in susceptible patients.
Initial Evaluation
Outside typical AFL, the clinician is faced with a wide spectrum of ATs for which therapy and prognosis cannot be defined with routine noninvasive testing, and definitive diagnosis typically requires intracardiac mapping. ECG alone is frequently inadequate to distinguish MRAT from focal AT. Detailed evaluation of cardiac function and anatomy is typically required, especially in patients with congenital heart disease and those with previous cardiac procedures (surgical or catheter based). In addition, detailed knowledge of the congenital anomaly and previous surgical or ablative procedures is very important, such as location of surgical incisions and the presence and location of prosthetic patch material.
Principles of Management
Medical management of MRAT is practically similar to that described for typical AFL ( see Chapter 12 ), including pharmacological rate- and rhythm-control strategies, as well as long-term anticoagulation for stroke. The choice of rate- versus rhythm-control strategies typically considers several factors, including severity of symptoms, response to rate-controlling medications, cardiac function, and associated noncardiac diseases.
With recent advances in the technology to visualize and modify the arrhythmia substrate, ablation of MRAT has become increasingly successful, but can be substantially more difficult than ablation of typical AFL. MRAT can have complex circuits that demand a thorough knowledge of atrial anatomy and a great level of experience to correlate activation patterns with anatomical landmarks. When this type of AT is suspected, such as in patients with congenital heart disease who have had surgery, referral to an experienced center should be considered.
Currently, catheter ablation is recommended in patients with recurrent symptomatic MRAT that is refractory to antiarrhythmic drug therapy, and may also be reasonable as a primary therapy, after carefully weighing potential risks and benefits of treatment options in the individual patient.
Electrocardiographic Features
P wave morphology on the surface ECG is usually of limited value for precise anatomical localization of macroreentrant circuits. Analysis of the P wave can be impeded by partial or complete concealment of the P wave within the QRS complexes or T waves when the AT is associated with 1 : 1 or 2 : 1 AV conduction. In addition, P wave morphology of a spectrum of RA and LA MRATs is highly variable. The presence of complex anatomy secondary to congenital abnormalities, prior atrial surgery, or a large low-voltage zone (secondary to underlying atrial substrate or extensive catheter or surgical atrial ablation) can modify atrial wavefront propagation in a nonuniform manner, resulting in deviated atrial activation vectors or low-amplitude P waves. Furthermore, P waves produced by different underlying substrates may appear similar if the direction of activation of the atrial septum and LA is similar.
The surface ECG morphology is most characteristic (and hence predictive) for establishing a diagnosis of counterclockwise typical AFL. Nevertheless, atypical ECG patterns have been described for typical AFL after AF ablation. Although clockwise typical AFL also has a characteristic appearance, this is more variable, and it can be mimicked by various other MRATs (“pseudo-typical” morphology). On the other hand, AFL may show distinct isoelectric intervals between P waves, especially in the presence of extensive atrial scarring or a localized macroreentrant circuit, similar to focal AT.
Despite significant limitations, certain ECG features have been demonstrated to help distinguish between the various types of MRAT. To distinguish LA from RA origin of the MRAT, lead V 1 is the most useful. In the absence of previous cardiac surgery or catheter ablation (particularly linear ablation lesions in the LA), P waves that are completely negative in lead V 1 are more frequently associated with RA free-wall circuits. Conversely, in the absence of counterclockwise typical AFL, broad-based upright P waves in lead V 1 , especially when accompanied by upright waves in inferior leads or with low-amplitude or isoelectric waves in the other leads, are more frequently associated with LA circuits. LA circuits frequently generate low-amplitude P waves, with some of them having visible waves only in lead V 1 . A decreased amplitude of the P waves in the inferior leads is suggestive of LA origin of MRAT. For ATs post AF ablation, negative P waves in any of the precordial leads favors RA MRAT over LA MRAT with a sensitivity and specificity of 83% and 100%, respectively, and an accuracy of 98%. Among LA MRATs, prominent positive flutter waves in lead V 1 favor LA roof or mitral isthmus–dependent MRATs over other LA MRATs, with a sensitivity and specificity of 80% and 83%, respectively, and an accuracy of 81%.
In general, a positive P wave in the inferior leads can be observed in clockwise typical AFL, upper loop reentry, and LA macroreentry. P wave polarity in lead I can help distinguish upper loop reentry from clockwise typical AFL. A negative or flat flutter wave polarity in lead I favors upper loop reentry, whereas positive polarity with amplitude of more than 0.07 mV indicates clockwise typical AFL.
Right Atrial Macroreentry
Incisional Right Atrial Macroreentry
The surface ECG morphology of free-wall MRAT in a patient with a previous atriotomy is highly variable, depending on factors including the location of the scars and low-voltage areas, the direction of rotation, the presence of coexisting conduction block in the atrium, and the presence of a simultaneous typical AFL. The morphology of the atrial complex on the surface ECG can range from that similar to typical AFL to one characteristic of focal AT ( see Fig. 14.3 ). Often, inverted P waves can be observed in lead V 1 . Depending on the predominant direction of septal activation, RA free-wall MRAT can mimic clockwise or counterclockwise typical AFL.
Upper Loop Reentry
The surface ECG of upper loop reentry closely mimics that of clockwise typical AFL (negative P waves in lead V 1 and positive P waves in the inferior leads) because, in most cases, both arrhythmias share a similar activation sequence of the LA, the septum, and caudocranial activation of the lateral RA. However, negative or isoelectric or flat P waves in lead I favor upper loop reentry over clockwise typical AFL. Conversely, positive P waves in lead I with an amplitude of more than 0.07 mV favor clockwise typical AFL. In addition, the CL of upper loop reentry is usually shorter in comparison with typical AFL.
Lower Loop Reentry
The surface morphology of lower loop reentry is highly variable and can be similar to that of counterclockwise or clockwise typical AFL, but lower loop reentry associated with somewhat higher crista terminalis breaks can produce unusual ECG patterns. Sometimes, the changes are manifested by decreased amplitude of the late positive waves in the inferior leads, probably as a result of wavefront collision over the lateral RA wall.
Intra-Isthmus Reentry
The surface ECG in intra-isthmus reentry commonly exhibits a pattern similar to counterclockwise typical AFL. The same ECG pattern can exist whether the intracardiac atrial activation sequence around the tricuspid annulus exhibits a counterclockwise, clockwise, or fusion pattern. The proximity of the exit site of the reentrant circuit to the CS os promotes more rapid CS and septal activation of the LA, which largely determine inscription of the P wave, regardless of the peritricuspid activation pattern.
Left Atrial Macroreentry
The surface ECG morphology of LA MRAT caused by the different reentrant circuits is variable, and ECG findings are often similar for different underlying substrates, thus making the localization within the LA based on the ECG difficult. LA MRATs are usually associated with prominent positive P waves in lead V 1 and upright (but frequently of low amplitude) deflections in leads II, III, and aVF. However, LA MRAT can result in ECG patterns of focal AT (discrete P waves and isoelectric baseline) because of a high prevalence of generalized atrial disease and slower conduction. Infrequently, LA MRAT can mimic typical AFL on the surface ECG.
Perimitral Left Atrial Macroreentry
Most of these tachycardias show prominent forces in leads V 1 and V 2 , with diminished amplitude in the inferior leads (see Fig. 13.4 ). It has been suggested that a posterior LA scar allows for domination by anterior LA forces. This constellation of findings can mimic counterclockwise or clockwise typical AFL, but the decreased amplitude of frontal plane forces suggests an LA circuit. In patients with prior PV isolation procedures, the surface ECG morphology of counterclockwise perimitral MRAT can be different from that in patients without prior ablation, possibly related to varying degrees of prior LA ablation or scar. In these patients, counterclockwise perimitral MRAT demonstrates positive P waves in the inferior and precordial leads and a significant negative component in leads I and aVL. Furthermore, counterclockwise perimitral MRAT in these patients can have a morphology similar to that of left PV ATs. However, counterclockwise perimitral MRAT is suggested in the presence of a more negative component in lead I, an initial negative component in lead V 2 , and a lack of any isoelectric interval between P waves. Clockwise perimitral MRAT has a limb lead morphology that is the converse of counterclockwise perimitral MRAT and an initial negative component in the lateral precordial leads. Positive P wave in leads I and aVL differentiates clockwise perimitral MRAT from counterclockwise CTI-dependent AFL and left PV AT.
Pulmonary Vein Circuits
Because these circuits are related to low-voltage or scar areas, the surface ECG usually shows low-amplitude or flat P waves. These tachycardias have the most variable surface ECG patterns.
Left Septal Circuits
Because the reentry circuit is on the septum, the surface ECG shows prominent, usually positive, P waves only in lead V 1 or V 2 and almost flat waves in most other leads ( eFig. 13.1 ). This pattern can be caused by a septal circuit with anteroposterior forces projecting in lead V 1 and the cancellation of caudocranial forces. This pattern was 100% sensitive for an LA septal circuit, but the specificity of this pattern for any type of LA MRAT was only 64%.

Electrophysiological Testing
A decapolar catheter (positioned into the CS with the proximal electrodes bracketing the CS os) and a multipolar (20- or 24-pole) Halo catheter (positioned at the tricuspid annulus) are commonly used to map MRAT. The distal tip of the Halo catheter is positioned at 6 to 7 o’clock in the left anterior oblique (LAO) view, so that the distal electrodes will record the middle and lateral aspects of the CTI, the middle electrodes will record the anterolateral RA, and the proximal electrodes may record the RA septum (depending on the catheter used). Instead of the Halo and CS catheters described, some laboratories use a single duodecapolar catheter around the tricuspid annulus while extending the catheter tip inside the CS. Such a catheter would straddle the CTI and provide recording and pacing from the medial and lateral aspects of the CTI.
Induction of Tachycardia
Programmed electrical stimulation protocols usually include atrial burst pacing from the high RA and CS—down to a pacing cycle length (PCL) at which 2 : 1 atrial capture occurs—and single and double atrial extrastimuli (AES) at multiple PCLs (600 to 300 milliseconds) from the high RA and CS (down to the atrial refractoriness). Isoproterenol infusion (0.5 to 4 µg/min) is administered as needed to facilitate tachycardia induction. The goals of EP testing in patients with MRAT are listed in Box 13.1 .
- 1.
To confirm that the tachycardia is an AT
- 2.
To confirm that the AT is a macroreentrant circuit
- •
Resetting response consistent with reentry
- •
Entrainment mapping consistent with reentry
- •
Atrial activation spanning the TCL
- •
- 3.
To exclude CTI-dependent atrial flutter
- •
Entrainment mapping at the CTI
- •
- 4.
To localize the circuit to the RA versus LA
- •
P wave morphology on the surface ECG
- •
Atrial activation sequence in the CS and Halo catheters
- •
Isolated variation of the RA CL
- •
RA activation time <50% of the TCL
- •
Entrainment pacing from different RA sites
- •
- 5.
To define the tachycardia circuit
- •
Electroanatomic mapping
- •
Entrainment mapping
- •
- 6.
To define the critical isthmus in the tachycardia circuit
- •
Entrainment mapping
- •
AT, Atrial tachycardia; CL, cycle length; CS, coronary sinus; CTI, cavotricuspid isthmus; ECG, electrocardiogram; LA, left atrium; RA, right atrium; TCL, tachycardia cycle length.
Tachycardia Features
MRAT is characterized by a constant TCL, a constant P wave morphology, and a constant atrial activation sequence. The atrial activation sequence depends on the origin and type of the macroreentrant circuit.
Although the atrial CL during MRAT typically remains constant, considerable variations in the CL can be observed in the atrium contralateral to atrium of origin of the tachycardia ( Fig. 13.5 ). In contrast, focal ATs frequently exhibit alterations in the CL with speeding (warm-up) and slowing (cool-down) at the onset and termination of tachycardia. Variation in the TCL of greater than 15% has been suggested as a reliable marker of a focal AT. However, a regular AT can be focal or macroreentrant. In addition, focal ATs often manifest as bursts of tachycardia with spontaneous onset and termination, although they can be incessant, and they may accelerate in response to sympathetic stimulus. Several criteria can help distinguish focal AT from MRAT ( see Table 11.5 ).

Atrial activation during MRATs spans the whole TCL. In contrast, intracardiac mapping in the setting of focal ATs shows significant portions of the TCL without recorded atrial activity, and atrial activation time is markedly less than the TCL, even when recording from the entire cardiac chamber of tachycardia origin ( see Fig. 11.5 ). However, in the presence of complex intramyocardial conduction disturbances, activation during focal tachycardias can extend over a large proportion of the TCL, and conduction spread can follow circular patterns suggestive of macroreentrant activation. On the other hand, long isoelectric intervals can occur between P waves during MRATs; especially when mapping is limited to only the atrium contralateral to the origin of the macroreentrant circuit or to only parts of the ipsilateral atrium, a focal activation can be observed, incorrectly suggesting a focal mechanism. This is particularly observed for LA MRATs in the presence of large areas of electrical silence. Nonetheless, a thorough intracardiac activation mapping would reveal atrial activation spanning the TCL.
Occasionally, P wave morphology on the surface ECG resembles AT, but intracardiac recordings show that parts of the atria (commonly the LA) have disorganized atrial activity ( Fig. 13.6 ). Such rhythms behave more like AF than AT, but they may be converted to true typical AFL with antiarrhythmic drugs.

MRAT is usually associated with 2 : 1 AV conduction, although variable AV conduction and larger multiples are not uncommon. Variable AV conduction is the result of multilevel block; for example, proximal 2 : 1 AV block and more distal 3 : 2 Wenckebach block result in 5 : 2 AV Wenckebach block. Macroreentrant circuits can have long CLs (i.e., 250 to 400 milliseconds) in the presence of extensive atrial disease and antiarrhythmic agents, in which case a relatively rapid ventricular response (with 1 : 1 AV conduction) can occur.
Diagnostic Maneuvers During Tachycardia
Atrial Extrastimulation During Tachycardia
A focal mechanism is characterized by dissociation of almost the entire atria from the tachycardia with AES. In contrast, the MRAT circuit usually incorporates large portions of the RA or LA, as demonstrated by resetting. In response to AES, MRATs typically demonstrate an increasing or mixed (flat, then increasing) resetting response. AES usually fails to terminate the AT.
Importantly, AES at short coupling intervals can result in transformation of the tachycardia to a different AT or to AF. To minimize the risk of transformation or interruption of the tachycardia, which would hinder tachycardia mapping, atrial stimulation maneuvers during the tachycardia should be used sparingly and only when needed to confirm the diagnosis, or more precisely, to localize the critical portion of the AT circuit as guided by the initial activation mapping.
Atrial Pacing During Tachycardia
Burst pacing from the CS or along the Halo catheter is started at a CL 10 to 20 milliseconds shorter than the atrial CL, and then the PCL is progressively shortened by 10 to 20 milliseconds. Consistent capture by atrial stimuli and acceleration of all recorded atrial electrograms to the paced rate should be verified before analyzing the tachycardia response to overdrive pacing. The response of AT to overdrive pacing is evaluated for entrainment, overdrive suppression, transformation into distinct AT morphologies or AF, and ability and pattern of termination.
Entrainment.
Overdrive atrial pacing at long CLs (i.e., 10 to 30 milliseconds shorter than the TCL) can usually entrain MRAT. The slower the pacing rate and the farther the pacing site from the reentrant circuit, the longer the pacing drive required to penetrate and entrain the tachycardia. Achievement of entrainment of the AT establishes a reentrant mechanism of the tachycardia and excludes triggered activity and abnormal automaticity as potential mechanisms ( Fig. 13.7 ). Entrainment can also be used to estimate qualitatively how far the reentrant circuit is from the pacing site (see later).


Entrainment criteria.
During constant-rate pacing, entrainment of a reentrant tachycardia results in the activation of all myocardial tissue responsible for maintaining the tachycardia at the PCL, with the resumption of the same tachycardia morphology following cessation of pacing, with the first postpacing ECG tachycardia complex displaying no fusion but occurring at a return cycle equal to the PCL. Unfortunately, it is almost impossible to document the acceleration of all tissue responsible for maintaining the reentrant circuit to the PCL. Also, it should be recognized that the mere acceleration of the tachycardia to the pacing rate and the subsequent resumption of the original tachycardia after cessation of pacing do not establish the presence of entrainment. Therefore several surface ECG and intracardiac electrogram criteria have been proposed for establishing the presence of entrainment ( see Box 5.2 ). Demonstration of one or more of the four criteria proves the presence of entrainment and supports a reentrant mechanism, but their absence does not exclude entrainment or reentry. Pacing at different endocardial sites and at multiple PCLs and recording activation sequences from multiple intracardiac locations are often required to demonstrate one or more of the entrainment criteria and, hence, establish the diagnosis of macroreentrant tachycardia.
Entrainment with fusion.
During entrainment of MRAT, fusion of the stimulated impulse can be observed on the surface ECG, but it is easier to recognize on intracardiac recordings from the Halo and CS catheters. The stimulated impulse has a hybrid morphology between the fully paced atrial impulse and the tachycardia impulse. The ability to demonstrate surface ECG fusion requires a significant mass of atrial myocardium to be depolarized by the paced stimulus and by the tachycardia. The closer the stimulation site is to the exit of a reentrant circuit, the less likely entrainment will manifest ECG fusion.
It is important to understand that overdrive pacing of a tachycardia of any mechanism can result in a certain degree of fusion, especially when the PCL is only slightly shorter than the TCL. Such fusion, however, is unstable in focal tachycardias during the same pacing drive at a constant PCL because pacing stimuli fall on a progressively earlier portion of the tachycardia cycle, thus producing progressively less fusion and more fully paced morphology. Such phenomena (referred to as “variable fusion”) should be distinguished from “constant fusion” and “progressive fusion” characteristic of entrainment, and sometimes this requires pacing for long intervals to demonstrate variable degrees of fusion. Focal ATs (automatic, triggered activity, or microreentrant) cannot manifest stable fusion during overdrive pacing (i.e., overdrive pacing during focal AT results in a fully paced P morphology and intracardiac activation sequence). Moreover, overdrive pacing frequently results in suppression (automatic) or acceleration (triggered activity) of focal ATs, rather than resumption of the original tachycardia with an unchanged TCL.
Entrainment with manifest fusion.
Entrainment of MRATs commonly produces manifest fusion that is stable (fixed) during pacing at a given PCL. Repeated entrainment at PCLs progressively shorter than the TCL results in different degrees of fusion between pacing episodes (while showing fixed fusion within any given episode of pacing), with the resultant P wave configuration looking more like a fully paced configuration as the paced rate increases.
Demonstration of the presence of manifest fusion during entrainment requires knowledge of the tachycardia surface P wave morphology and of pure pacing (at the same site and rate) in the absence of tachycardia. However, in the setting of MRAT, P wave morphology during tachycardia or pure pacing may not be easily visualized in the presence of overlapping QRS complexes and ST-T waves; hence, the intracardiac atrial activation sequence can be utilized as a surrogate to ECG morphology to demonstrate fusion and is preferable due to the ability to have many discrete recordings for comparison of paced versus tachycardia sequences. Manifest fusion is said to be present when the ECG morphology (or intracardiac activation sequence) is a hybrid of the complex morphology of the tachycardia and that observed during pure pacing.
However, fusion can sometimes be difficult to recognize on the surface ECG because the morphology of the purely paced ECG complex may not be readily available. In this setting, the presence of manifest fusion can be inferred by of one of the following observations:
- 1.
The surface P wave during entrainment is different from pure tachycardia morphology and the onset of the P wave precedes the pacing stimulus artifact of each paced beat by a fixed interval (see Fig. 13.7 ; see Fig. 12.12 ). This observation provides evidence that the tachycardia wavefront has exited from the circuit, and that the initial portion of the P wave (inscribed before the pacing stimulus artifact) is activated orthodromically by the tachycardia wavefront while the latter portion is activated by the paced wavefront.
- 2.
The surface P wave morphology (or intracardiac activation sequence) is different from the pure tachycardia morphology but is inconsistent with the expected morphology during pure pacing at a particular site (for example, AT entrainment from the distal CS producing a proximal-to-distal CS activation sequence).
- 3.
The demonstration of shortening of conduction time (and change in electrogram morphology) at an intracardiac electrode recording site in response to increasing pacing rates during entrainment. Because conduction velocity with an increasing rate is expected to stay the same or decrease, but not increase, a decrease in conduction time (same paced site, same recorded site) in relation to a faster pacing rate demonstrates that there are two routes of activation and that the faster one can only conduct to the recording site at faster pacing rates. This represents the “fourth” entrainment criterion and is the intracardiac equivalent of the second entrainment criterion (progressive fusion). The amount of tissue antidromically captured is critically dependent on the pacing rate. If the recording site is located in an area activated orthodromically at a slower rate and antidromically at a faster rate, the conduction time will dramatically shorten at the faster pacing rate.
- 4.
The demonstration of pacing from electrodes on a multipolar catheter that have late activation times, results in acceleration of electrograms recorded from other electrodes on the same catheter that had earlier activation times during tachycardia ( Fig. 13.8 ). This downstream pacing affecting upstream recordings has been shown to correlate with macroreentry with high specificity.
Fig. 13.8
Entrainment of Scar-Related Macroreentrant Right Atrial Tachycardia.
Overdrive pacing is performed from the lateral right atrium ( Halo 7–8), a site that is “downstream” from other electrodes with earlier activation during tachycardia, but nonetheless controls electrograms that are “upstream” from it on the next cycle. Electrograms shaded in blue are activated at a paced cycle length, indicating they are controlled by the last pacing stimulus, whereas electrograms shaded in red are activated at the tachycardia cycle length. This phenomenon is an indicator of macroreentry.
Entrainment with inapparent fusion.
Entrainment with inapparent fusion (also referred to as “local” or “intracardiac” fusion) is said to be present when a fully paced P morphology (with no ECG fusion) results, even when the tachycardia impulse exits the reentrant circuit (orthodromic activation of the presystolic electrogram is present). In this setting, fusion is limited to a small area and does not produce surface ECG fusion, and only intracardiac (local) fusion can be recognized (and even then, only with recording electrodes in the correct location).
Entrainment with concealed fusion.
Entrainment with concealed fusion (sometimes also referred to as “concealed entrainment” or “exact entrainment”) is defined as entrainment with orthodromic capture and a surface ECG complex identical to that of the tachycardia. Entrainment with concealed fusion suggests that the pacing site is within a protected isthmus, either inside or outside of, but attached to, the circuit’s diastolic corridor.
Pacing in a protected isthmus, either inside or outside or attached to the reentrant circuit isthmus, forces the paced wavefront to travel in one (orthodromic) direction through the same reentrant pathway as the tachycardia wavefront. Propagation of the paced wavefront in the opposite (antidromic) direction is prevented by either a dead end (when one end of the protected isthmus is attached to the circuit and the other end is a dead end) or by colliding with the previous reentrant wavefront propagating orthodromically through the reentrant circuit (when both ends of the protected isthmus are attached to the circuit, whether the isthmus is critical to the circuit or just a bystander). In either situation, the paced wavefront is forced to use the same reentry circuit exit to activate the rest of the atrial myocardium and is prevented from activating the myocardium by propagating in any other direction. Hence, the entrained P wave morphology is identical to that of the tachycardia.
Termination.
Rapid atrial burst pacing can usually terminate MRAT. However, termination is less likely when the pacing drive is short or the pacing site is distant from the reentrant circuit. It is important to avoid unintended termination of MRAT because it can be very difficult to reinitiate the clinically relevant AT, particularly in a patient with complex scar and multiple potential circuits.
Overdrive suppression.
Overdrive suppression analogous to that seen with automatic AT is not expected in MRAT. As noted, the PPI remains relatively stable when entrainment of MRAT is performed at the same site, regardless of the length of the pacing drive. This is in contrast to overdrive suppression seen in automatic ATs, which would be associated with progressive delay of the first tachycardia beat return cycle with progressively longer pacing drives.
Transformation.
Rapid atrial burst pacing can potentially convert MRAT to AF, to typical AFL, or to another type of MRAT. The risk of tachycardia transformation is significant, especially given the frequent prevalence of complex combinations of anatomic and functional obstacles that can support multiple reentrant circuits. Therefore pacing maneuvers during AT should be used sparingly, and only when necessary to confirm the role of critical areas of the circuit (during entrainment mapping, as discussed later). Detection of tachycardia transformation requires careful attention to the atrial activation sequence and the ECG pattern after cessation of pacing. Also, recording multiple simultaneous electrograms on multipolar catheters (e.g., Halo and CS catheters), as continuous endocardial references, facilitates detection of changes in atrial activation sequence.
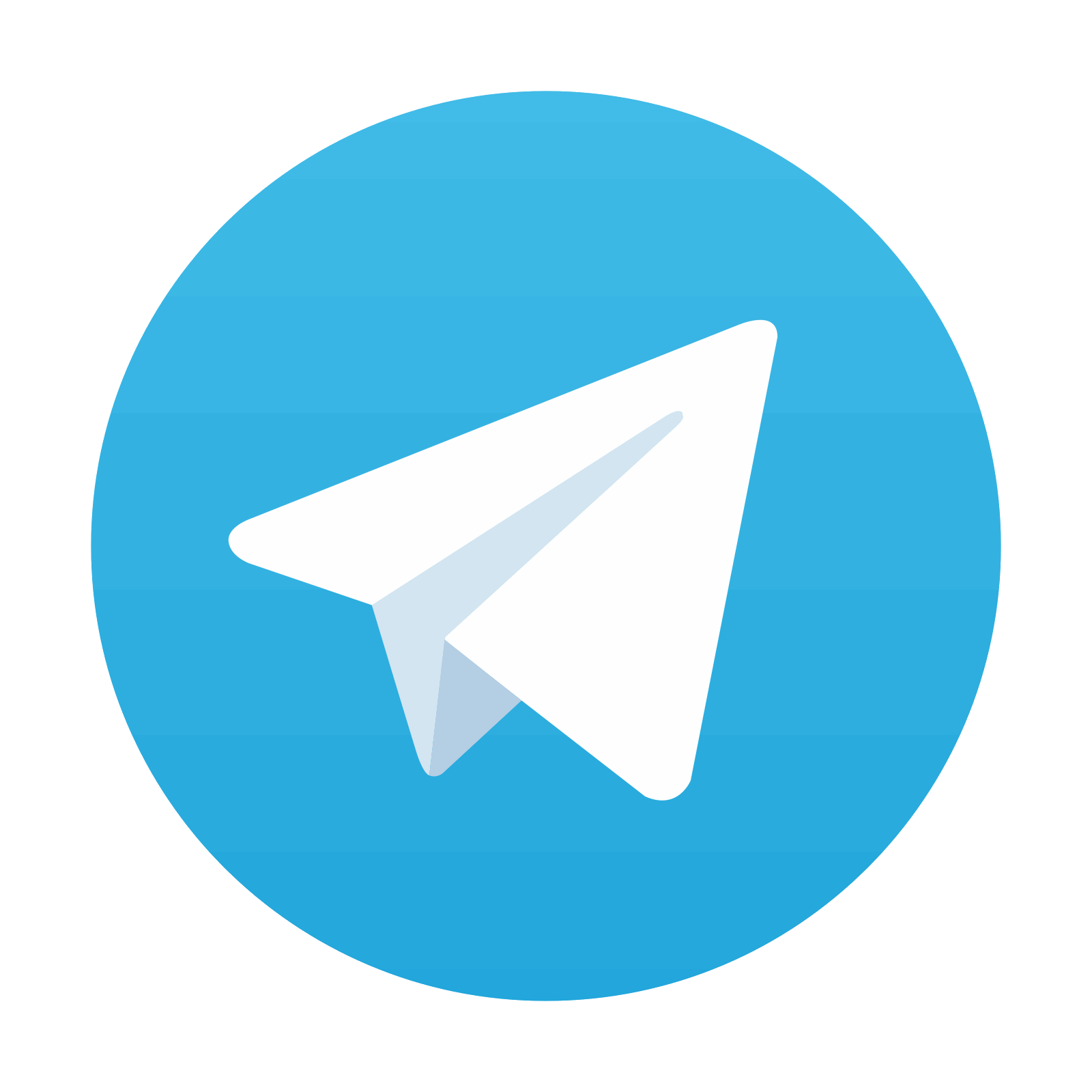
Stay updated, free articles. Join our Telegram channel

Full access? Get Clinical Tree
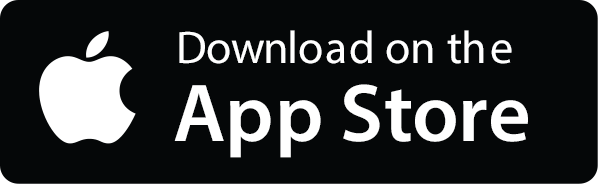
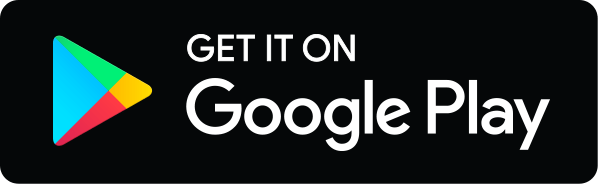