(1)
University of Padova Medical School, Department of Cardiothoracic and Vascular Sciences, Pathological Anatomy Section, Padova, Italy
Lung transplantation-related pathology includes a large box of disorders such as (a) explant pathology, (b) perioperative allograft pathology, (d) rejection (acute and chronic), (e) infections, and (f) tumors.
In all these settings, the pathologist plays a key role for final diagnostic characterization.
16.1 Explant Pathology
Lung transplantation has become an accepted modality of treatment for several end-stage pulmonary diseases that can be categorized in three main groups. These are obstructive, restrictive, and vascular diseases, the latter including both primary and secondary causes. The study of native lungs represents a unique opportunity to carefully study whole lungs without the superimposed agonal complications seen in autopsy specimens. The availability of fresh lung samples allowed a lung biobank to be set up, a valuable source for research into a range of pulmonary diseases [1]. Diagnostic characterization of lung diseases prior to transplantation has often been made on clinical and radiological data. Examination of the explanted lungs often permits a more accurate diagnosis and represents an audit of thoracic medical practice. A review of explanted lungs over a long period (10 years and 12 years) performed by two European lung transplant centers (Papworth, UK, and Padova, IT) reported important findings [2, 3]. Both centers reported significant discrepancies between referral and pathologic diagnoses, some of them with significant impact on recipient management. In the Padova experience, the rate of major discrepancies was mainly detected in the group of interstitial lung diseases (ILDs), mainly in those cases without a pretransplant biopsy diagnosis. Incorrect histological pretransplant diagnoses could be due to a truly difficult morphologic characterization of ILDs, performed late in the course of the disease often obtaining insufficient samples with frequently fading histopathologic markers. Another important reason is that some patients with ILDs were not investigated in the transplant unit but in hospitals without specific experience in this field. A multidisciplinary specialist approach and experience in the diagnosis of ILDs are considered mandatory for accurate diagnosis [4]. An important step to improve the accuracy of referral diagnosis is that every lung biopsy should be reviewed by skilled pathologists of transplant centers at the time of assessment. If necessary an additional biopsy should be planned if permitted by the patient’s clinical conditions [2, 3].
16.1.1 Obstructive Diseases
The principal end-stage lung diseases of this group include cystic fibrosis, bronchiectasis, and emphysema.
Cystic fibrosis: Life expectancy in cystic fibrosis (CF) has improved substantially over the last 75 years, with a median predicted survival now approaching 40 years; however CF and non-CF bronchiectasis are the most important chronic suppurative diseases requiring lung transplantation, usually bilateral lung transplantation. The availability of stored explanted CF lungs has significantly supported research into pathogenesis, providing also detailed histopathological reports. CF is caused by mutations in the gene encoding the CF transmembrane conductance regulator (CFTR) protein [5]; however, the pathophysiology of CF lung disease remains incompletely understood. In particular the contribution of inflammation, airway remodeling, and other factors remain undefined. A better knowledge of the disease in particular the true onset (usually it begins before symptoms) is extremely important for suggesting new preventions and treatments. The lack of an animal model that mirrors CF in humans has delayed progress in discovering the origins of the lung disease [6]. Lung disease such as that in humans does not develop in mice with cftr mutations, even if new animal models have recently been successfully generated [7]. Interesting findings coming from the study of explanted lungs are now changing the pathogenetic view. Fibro-obliterative processes either isolated to the airways, i.e., obliterative bronchiolitis, or as a part of organizing pneumonia of a bronchiolitis obliterans-organizing pneumonia pattern are frequently detected. This has renewed the pathogenesis of the disease with more interest in the small airways instead of large airways, which has been the main area of clinical and pathologic interest. Other important data frequently detected from careful morphological studies is the presence of emphysema, until recently considered minimal [8, 9]. Mets et al. assessing by morphometry explanted lungs of 20 CF patients and found that emphysema is common and age related [10]. Emphysema might become an increasingly important disease component in the aging CF population. This finding appears of particular importance due to the fact that life expectancy of CF patients has dramatically increased in the last decades.
Other associated pathologic lesions that can be detected in end-stage CF and non-CF bronchiectasis lungs are Aspergillus or mycobacterial infections and neuroendocrine lesions (i.e., carcinoids and tumorlet); both lesions are important and should be described in the diagnostic report for more careful monitoring of recipients.
16.1.1.1 Emphysema
Emphysema/chronic bronchitis with or without alpha-1-antitrypsin (AAT) deficiency is a common indication for lung transplantation. Unexpected findings have been found in explanted lungs such as asthma lesions and infective complications, the latter with important implications in the remaining native lung when a single lung procedure is used [2, 3]. New discoveries have recently changed the pathogenesis of the disease: the importance of inflammatory response has been highlighted, inviting a broader look at the possible involvement of the immune system in the pathogenetic events of chronic obstructive pulmonary disease [11]. Emerging evidence has emphasized the role of adaptive immune responses, possibly with an autoimmune component. The study of emphysematous native lungs detected many stigmata of autoimmune disease such as the consistent presence of B-cell infiltration sometimes aggregate in follicular patterns and the presence of BAFF pathway and cytokines such as interleukin 32 that play a key role in various autoimmune diseases [12–14]. Inflammation is also evident in AAT deficiency-related emphysematous lung. The disease appears far from “burnt out,” and the interstitial space, both in irreversibly damaged and less affected areas, shows extensive inflammatory infiltration [12, 15]. The presence of complex cell infiltration even in end-stage disease promotes the development of new treatment strategies for a more effective control of inflammation.
16.1.2 Restrictive Diseases
Among restrictive forms idiopathic pulmonary fibrosis (IPF) represents the most frequent referral end-stage lung disease. IPF, the most common form of idiopathic interstitial pneumonias, is a chronic, progressive, irreversible, and usually lethal lung disease often occurring in middle-aged and elderly adults (median age at diagnosis 66 years, range 55–75 years) [16]. The annual incidence of IPF is rising and is estimated to be between 4 · 6 and 16 · 3 per 100,000 people, and the prevalence is 13–20 cases per 100,000 [17–19]. The main histopathological features of IPF, named usual interstitial pneumonia, is characterized by a heterogeneous appearance with areas of subpleural/paraseptal fibrosis and honeycombing alternating with areas of less affected or normal parenchyma (spatial heterogeneity). Small areas of active fibrosis called fibroblast foci are present in the background of collagen deposition, and they reflect the temporal heterogeneity of the process. Inflammation is usually mild and consists of a patchy lymphoplasmacytic interstitial infiltrate. All these pathologic changes can be also detected in end-stage disease of explant lungs. More inflammation and moreover a temporally uniform distribution of the lesions are present in other interstitial lung diseases (desquamative interstitial pneumonia, nonspecific interstitial pneumonia, acute interstitial pneumonia, hypersensitivity pneumonia, collagen vascular diseases) which should be distinguished from UIP/IPF due to a better responsiveness to steroid treatment, better prognosis, and rarely indication for lung transplantation [19]. Multidisciplinary teams with high expertise and experience have been recommended by several authors to obtain a more correct final diagnosis [5, 18]. In our internal audit, both minor and major discrepancies were found in explanted lungs with a clinical diagnosis of IPF [3]. One of the most important lesions detected in our IPF explanted lungs was the presence of lung cancer; all our recipients with unsuspected neoplasia had a poor posttransplant follow-up (death or disease recurrence).
Today the increased diagnostic sensitivity of noninvasive techniques (such as high-resolution computed tomography scan) particularly if performed in referral centers have significantly reduced the use of lung biopsy [19]. Thus explanted lungs now represent an important tissue source available for any type of research. Many recent views about the IPF/UIP pathogenesis have just come from the study of native lungs. The recent hypothesis of IPF pathogenesis indicates that injured and hyperplastic alveolar epithelial cells are able to release cytokines causing proliferation of fibroblasts/myofibroblasts and deposition of extracellular matrix. Among profibrogenetic cytokines, transforming growth factor β (TGF β) represents the key growth factor which leads to mesenchymal differentiation, myofibroblast infiltration, and proliferation. Interestingly, the molecular pathways aberrantly activated in IPF seem to be the same involved in epithelial-mesenchymal communications occurring in lung development and differentiation [20].
Our experience, studying a large number of IPF native lungs, demonstrated that there is an abnormal epithelial renewal with diffuse signs of morphological changes through variegated cellular alterations, such as hyperplasia, atypia, squamous metaplasia, and various degrees of dysplasia [21, 22]. In particular, hyperplasic lesions and epithelial atypia have often been detected in highly remodeled lung areas as honeycomb.
This area represents a site of high instability at risk of neoplastic transformation, which are more frequently of squamous type [23–30]. Serpin B3/B4, a clade B serpin involved in several pathways including apoptosis/proliferation imbalance, has been found overexpressed in patients with squamous cell carcinoma type of different organs [31–38] and in metaplastic/dysplastic area of IPF lungs. Different works, in vitro and in vivo (both human and animal), have demonstrated a strict influence between serpin B3 and TGF-ß [39, 40]. Apoptosis/proliferation imbalance with a significant gain of epithelial proliferation has recently been demonstrated in bleomycin-induced lung damage using serpin B3 transgenic mice [40]. The overexpression of serpin B3/B4, particularly the isoform B4, in IPF patients may be an important factor in the resistance of injured epithelial cells to apoptosis, thus leading to progressive lesions at high risk of malignant transformation. Interestingly our group found a direct relationship between serpin B3/B4 and TGF-β expression [22, 39]. The ov-serpins have been shown to render cells more resistant to apoptotic cell death, and proapoptotic effect of TGF-β might represent a counterbalancing mechanism leading to accumulation of fibrosis as a secondary effect. Epithelial mesenchymal transition, typically ascribed to TGF-ß [41], has also been related to serpin B3 [42]. The study has provided evidence that the antiprotease activity of serpin could be implicated in the TGF-ß induction.
Another important aspect extensively explored in lung tissue sampled from IPF native lungs concerns the investigation of viral infection as a contributing etiological factor [43]. Several studies have implicated viral infection as a cause of epithelial injury and therefore an important factor in its pathogenesis [44–61]. Among viral agents herpes viruses, in particular Epstein-Barr virus (EBV), have been suggested as principal cofactors (as initiating or exacerbating agents) of fibrotic lung disease, and treatment with ganciclovir has been shown to attenuate disease progression in a subgroup of patients [62]. Our experience demonstrated a different phenotype of virus-positive IPF patients. In particular virus-positive IPF cases showed more pronounced vessel remodeling and a higher mean pulmonary arterial pressure (mPAP) and significantly higher primary graft dysfunction after transplantation. While there is large mechanistic evidence of epithelial herpesvirus-associated alveolar injury, the effect of these viruses on the pulmonary vasculature in IPF merits investigation. A deeper knowledge of viral-induced pathways in endothelial cells could give new insights for a targeted therapeutic approach to pulmonary hypertension, one of the most important IPF complications.
16.1.3 Vascular Disease (Pulmonary Hypertension)
Pulmonary hypertension, either primary or secondary origin, is considered an important indication for lung transplantation (with and without the heart). Patients with pulmonary hypertension secondary congenital heart disease and patients with end-stage thromboembolic pulmonary hypertension are usually diagnosed on native lungs at transplantation. However chronic thromboembolic pulmonary hypertension is now rarely considered an indication for transplantation being treated surgically by pulmonary thrombectomy. There is an increasing focus on the cellular and molecular pathogenesis of idiopathic pulmonary hypertension. Animal models do not reproduce the morphological features of human pulmonary hypertension, thus it is mandatory that the human tissue available through transplantation is adequately studied. Many studies have given significant improvement in the understanding of pathogenesis and evolution of plexiform lesions leading to better clinical staging and more effective treatment strategies [63]. Explanted lungs were also successfully used for several studies focusing on key biomarkers such as the expression of genes for bone morphogenetic protein receptor type II (BMPR2) and TGF-β type I and II receptors [64]. The group of Papworth studying explanted lungs with primary pulmonary hypertension demonstrated that TGF-β R1 and RII co-localized to vascular endothelium in the normal pulmonary circulation, with reduced expression of TGF-β RII subtype in plexiform and intimal lesions of primary pulmonary hypertension [64]. Evidence is emerging that germline and/or somatic mutations in key cell growth regulatory genes play a role in the development of idiopathic sporadic and familial pulmonary hypertension [65]. Our group studied lungs with pulmonary hypertension (primary and secondary forms) and demonstrated increased intrapulmonary endothelial progenitor cells (EPC), suggesting that these cells may contribute to vascular remodeling [66]. The localization of specific cell markers and the identification of EPC are very likely to have an impact on therapy for both pre- and posttransplant conditions.
16.2 Perioperative Complications
These include surgical complications (anastomotic airway and vascular complications) and ischemia reperfusion injury. The most recent International Society of Heart-Lung Transplantation (ISHLT) registy, reports early graft failure in 2,3 % of recipients with a significant high 30-day rate mortality mainly due to perioperative complication [67]. Anastomotic airway complications may be characterized by formation of granulation tissue which sometimes may appear as exuberant polypoid granulation tissue. Superimposed infection, including fungi and bacteria, can often complicate the healing. Ischemia/reperfusion injury occurs in 10–20 % of patients. Transbronchial biopsies of the recently implanted lung may show a number of minor morphological changes such as peribronchiolar and perivascular edema, lymphatic dilatation, and neutrophil infiltration. In cases with important ischemia/reperfusion injury, the biopsy shows diffuse alveolar damage and organizing pneumonia whose features should be distinct by hyperacute lung rejection. The absence of perivascular and airway mononuclear cell infiltrates distinguishes it from acute rejection.
16.3 Lung Allograft Rejection
In the ISHLT 2012 report, lung transplantation-related pathology, specifically acute rejection, was reported to affect 34 % of lung transplant recipients within the first postoperative year. In the revised working formulation for the standardization of nomenclature in the diagnosis of rejection, the key role of biopsy for final diagnosis of rejection was again emphasized, while the most sensitive tool for infection is bronchoalveolar lavage usually collected at the same time of biopsy [68]. Adequacy of specimens (at least five slices of well-expanded alveolated lung parenchyma) and histological processing (three-level serial section of paraffin block and additional special stains for microorganism and collagen deposition) have been reported in the revised international grading scheme as general recommendations to reach a more sensitive diagnosis.
Lung allograft rejection is classified as hyperacute, acute, and chronic and affects the vasculature and the airways. The rejection process may involve humoral/antibody-mediated and cell-mediated immune mechanisms.
16.3.1 Hyperacute Lung Rejection
Hyperacute rejection is an early complication of transplantation, caused by the presence in the recipient of preformed donor-directed antibodies. It occurs within 24–48 h of graft implantation and should be suspected when the lungs become markedly congested and edematous with the production of copious frothy bloodstained sputum from the bronchial orifice of the graft. The histologic features of hyperacute rejection include diffuse alveolar damage, alveolar hemorrhage, interstitial pneumonia, and vasculitis. As only a few well-documented cases of hyperacute rejection of the lung have recently been reported in the literature [69, 70], it was not included in the working formulation. Ancillary techniques are useful for the final diagnosis: immunofluorescence may show IgG and complement (C3d and C4d) deposition in the alveolar septa. Immunohistochemistry for C4d should be interpreted as positive only when strongly stained deposition are detected.
16.3.2 Acute Rejection (Grade A, B)
The diagnosis is based on the presence of perivascular or airway inflammatory infiltrates that are composed mainly of activated lymphocytes, few eosinophils, neutrophils, and plasma cells (grade A). Lymphocytic bronchiolitis can also be observed and is characterized by bronchiolar inflammatory cell infiltrates with epithelial cell ulceration in severe forms (grade B). The histologic grade is based on the intensity and distribution of the infiltrate (Table 16.1; Fig. 16.1a–d).

Rejection | Grading | Histological features |
---|---|---|
Acute rejection | Grade AX Ungradable | No alveolated lung tissue or no arteriole/venule |
Grade A0 No acute rejection | Parenchyma without perivascular inflammation | |
Grade A1 Minimal acute rejection | Perivascular infiltrates forming an incomplete or a 2-or 3-cell-layer-thick cuff. Rare eosinophils; endothelialitis usually absent | |
Grade A2 Mild acute rejection | More frequent perivascular infiltrates consisting of lymphocytes, macrophages, eosinophils, and endothelialitis | |
Grade A3 Moderate acute rejection | Dense perivascular mononuclear cell infiltrates with frequent foci of endothelialitis and extension into alveolar walls; eosinophils and neutrophils often present | |
Grade A4 Severe acute rejection | Diffuse perivascular, interstitial and alveolar infiltrates; pneumocyte damage, endothelialitis, intra-alveolar necrotic epithelial cells, hyaline membrane hemorrhage, and neutrophils | |
Lymphocytic bronchiolitis | Grade BX Ungradable | Insufficient sample, artifact, infection |
Grade B0 No lymphocytic bronchiolitis | No evidence of airway inflammation | |
Grade B1R Low-grade small airway inflammation | Few lymphocytes and plasma cells within submucosa, rare eosinophils | |
Grade B2R High-grade small airway inflammation | More inflammation with greater numbers of eosinophils and plasmacytoid cells. Epithelial damage and marked intraepithelial lymphocytes | |
Chronic airway rejection | Grade C0 No airway rejection | No evidence of obliterative bronchiolitis |
Grade C1 Airway rejection | Asymmetric or concentric/partial or complete airway obstruction | |
Chronic vascular rejection | Grade D Vascular rejection | Presence of fibrointimal thickening with/without inflammation |
Grade D0 No vascular rejection | No evidence of vascular thickening (atherosclerosis) |

Fig. 16.1
(a) Grade A1 minimal acute rejection: a high power view reveals a minimum perivascular mononuclear cell infiltrate with no evidence of endotheliitis, usually occurring in a background of entirely normal lung parenchyma. (b) Grade A2 mild acute rejection: a dense perivascular infiltrate with expansion of the adventitial space is seen, but no involvement of alveolar walls. (c) Grade A3 moderate acute rejection: a dense perivascular infiltrate with endothelial involvement and extension into adjacent airspaces is well visible. The inflammatory infiltrate consists of small and large lymphocytes, macrophages, and polymorphs. (d) Grade A4 severe acute rejection: a section from an autopsy case demonstrating perivascular infiltrate associated with hemorrhage and edema with alveolar capillary congestion and hyaline membrane formation. Perivascular distribution is not a feature of diffuse alveolar damage due to reimplantation/perfusion injury, infection, hypovolemic shock, or other causes of posttransplantation diffuse alveolar damage.
16.3.3 Chronic Rejection (Grade C and D)
Chronic rejection is primarily manifested as bronchiolitis obliterans (grade C). It is the most significant complication limiting long-term survival, affecting up to 50 % of patients 3 years after transplantation. Previous episodes of acute rejections are the most well-recognized risk factor. Since bronchiolitis obliterans (BO) is a patchy process and diagnostic yield of transbronchial biopsy is low, pulmonary functional tests are considered as more sensitive tools: when the decline is greater than 10 % of baseline, a clinical diagnosis of BO syndrome is made (without pathologic confirmation) and graded from 1 to 3 based on the degree of loss of lung function [71] (Table 16.1; Fig. 16.2a).


Fig. 16.2
(a) Grade C1 obliterative bronchiolitis: little cellular infiltrate and marked concentric fibrotic narrowing of the bronchiolar lumen is seen. The smooth muscle in the wall is interrupted by the bronchiolar fibrosis. (b) Grade D chronic vascular rejection: a section from an autopsy case of chronic lung allograft dysfunction showing a thickened vessel with massive fibrointimal fibrosis
16.3.4 Emerging Immunological Lesions
16.3.4.1 Antibody-Mediated (Humoral) Rejection
Emerging data suggest that humoral immunity plays an important and previously underestimated role in lung rejection and chronic allograft dysfunction. Antibody-mediated rejection (AMR) is believed to be triggered by the binding of donor-specific antibodies to the lung microvasculature. Currently AMR is a widely accepted and well-defined form of rejection in the fields of kidney and heart transplantation. A summary statement on the pathology of AMR following lung transplantation has recently been proposed [72]. However, the morphological aspect, including histology and immunohistochemistry in the lung, defies accurate characterization. The histopathology criteria of lung AMR were not addressed in the 1990 and 1996 ISHLT classifications of lung allograft rejection and were not clearly defined in the revised 2007 ISHLT classification [68]. The 2012 statement from the Pathology Council reported “Non-specific patterns of injury that can also be seen in disorders such as severe acute cellular rejection, infection (especially bacterial and viral), graft preservation injury and drug reaction.” Reported AMR histological findings such as neutrophilic capillaritis, arteritis, and diffuse alveolar damage are so broad that it is difficult to diagnose AMR. The significance of complement 4d (C4d) deposition in the diagnosis of AMR in lung allografts is controversial. A potential cause may be the problematic reproducibility of immunostaining. A multidisciplinary approach seems to be the best way to diagnose AMR in lung allograft biopsies (Fig. 16.3a, b).


Fig. 16.3
(a, b) Antibody-mediated rejection: histological section showed widening of interstitial spaces with mixed inflammatory cell infiltration (a), immunohistochemistry shows a crisp lining of C4d deposition along the endothelial cells of capillaries and small vessels (b). The patient had high level of donor-specific antibody
2) Restrictive allograft syndrome (RAS): RAS is a novel form of chronic lung allograft dysfunction after lung transplantation. Although BO syndrome, characterized clinically by irreversible obstructive deficits in pulmonary function tests, remains the major cause of late mortality, recently a distinct form of chronic lung allograft dysfunction demonstrating restrictive pulmonary function decline has been reported with an estimated frequency of 25–35 % of chronic lung allograft dysfunction [73, 74]. Pleuroparenchymal fibroelastosis is a major histopathologic correlate of restrictive allograft syndrome. Idiopathic pleuroparenchymal fibroelastosis as a distinct entity was first proposed by Frankel et al. in 2004 [75]. It is characterized radiologically by features suggestive of a chronic interstitial pneumonia with upper lobe predominance. From histological aspects it is characterized by pleural fibrosis and parenchymal fibroelastosis in a predominantly subpleural distribution, with a sharp demarcation between fibroelastotic and unaffected lung parenchyma, and with the presence of fibroblastic foci at this interface (Fig. 16.4). Pleuroparenchymal fibroelastosis in restrictive allograft syndrome patients is often present concurrently with features of diffuse alveolar damage as well as BO [76]. The etiologic factors underlying restrictive allograft syndrome and pleuroparenchymal fibroelastosis remain uncertain. Immunological mechanism (autoantibodies) and/or infections have been suggested to play a contributing role in the development and progression of the lesion [77, 78].


Fig. 16.4
(a) Cytomegalovirus infection: bronchoalveolar lavage demonstrates viral inclusion of CMV in the center of field. (b) Bacterial infection: the lavage shows necrotic debris and polymorphs in the background. Gram staining reveals numerous gram-positive bacteria. (c) Aspergillus infection: Grocott silver stain shows the pathognomonic features characterized by dichotomous branching and septate, broad, and regular hyphae. (d) Pneumocystis carinii infection: bronchoalveolar lavage shows cluster of microorganism cysts
16.4 Infections
Lung transplant recipients are at risk of developing infections that can be bacterial, viral, or fungal. In the context of infection, the pathologist should take into consideration several aspects, the most important include (1) differentiation of acute rejection from viral infection; (2) differentiation between colonization, subclinical infection, and significant clinical disease; and (3) identification of infections with histological patterns that may differ from those observed in the non-transplanted, immunocompetent patient. Bronchoalveolar lavage is the best tool for more sensitive diagnosis of various forms of infections (Fig. 16.4a–d).
16.4.1 Viral Infection
Cytomegalovirus (CMV) infection is the most important cause of pneumonia. Primary infection, through blood transfusion or transmitted in the graft, may cause very severe disease in the allograft with systemic spread. Reactivation in a seropositive recipient may cause clinical disease that is less severe than in seronegative recipients. A prophylactic regimen has significantly reduced the severity and the frequency of CMV pneumonia [79–81]. CMV pneumonia is diagnosed in transbronchial biopsies by finding the classical single intranuclear and multiple small cytoplasmic inclusions in an enlarged cell. Treated patients may show more frequently smudged eosinophilic inclusions which may be difficult to identify as CMV. In these cases the use of in situ hybridization or immunohistochemistry against immediate-early antigen could be extremely useful [82, 83]. Routine histology with immunohistochemistry and/or in situ hybridization shows however low sensitivity in CMV detection often leading to underdiagnosis and/or delayed diagnosis of CMV pneumonitis. Increasing interest has focused on more sensitive molecular detection techniques both applied to transbronchial biopsy and overall to bronchoalveolar lavage. Although the critical threshold of viral load (4.6 × 104 and 5.0 × 105) is not yet completely validated, several works have proposed CMV quantification in bronchoalveolar lavage to have a higher sensitivity in the differentiation of patients with active CMV disease from latently infected patients, and they suggested that molecular quantitative assay on bronchoalveolar lavage should replace traditional culture and also CMV DNA assay in the blood [84–86]. The use of these ancillary techniques is overall useful to differentiate histological changes from acute rejection particularly when both diseases coexist and inclusions are sparse. Indeed CMV is known to be associated with an increased incidence of allograft dysfunction, possibly through upregulation of MHC class I and class II antigens in alveolar cells [87, 88]. In severe infection, diffuse alveolar damage is the prevalent histological finding. Adenovirus infection is more common in children, and scattered adults and pediatric patients develop serious pneumonias due to other respiratory viruses (e.g., respiratory syncytial virus, rhinovirus, parainfluenza, influenza).
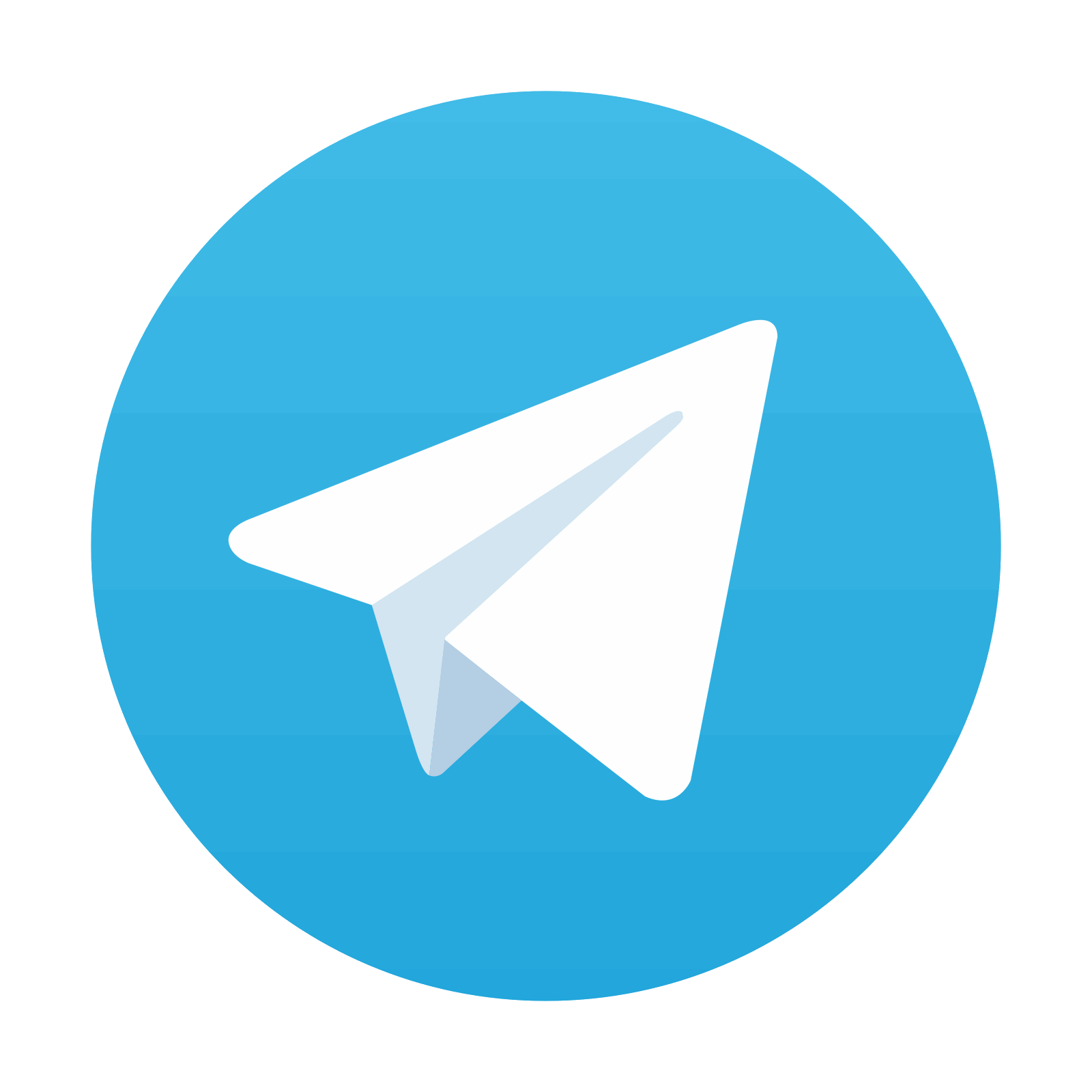
Stay updated, free articles. Join our Telegram channel

Full access? Get Clinical Tree
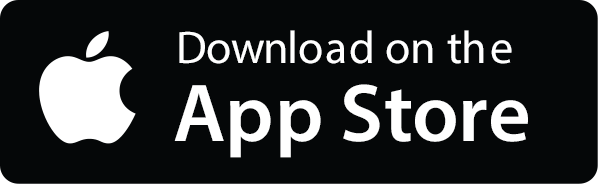
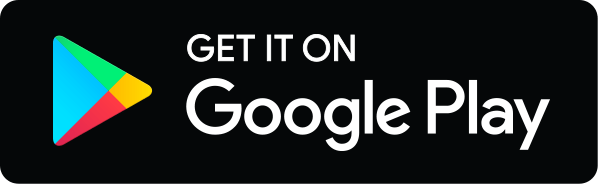