Chapter 2
Lung physiology
Mustafa Zakkar, Ian Hunt
1 | What is pulmonary ventilation? |
• | Pulmonary ventilation refers to the movement of air to and from the lungs during breathing. |
2 | Describe the airway structures involved in ventilation |
• | Air enters the nose and passes via the nasopharynx to the larynx. After passing the epiglottis, it then enters the trachea (Figure 1). |
The trachea divides into left and right main bronchi (primary bronchi), which in turn divide into segmental bronchi (secondary bronchi), subsegmental bronchi (tertiary bronchi) and, finally, multiple bronchioles. | |
• | Bronchioles differ from bronchi as they lack cartilage, thereby making them collapsible. |
• | The airways terminate in alveoli, which are the site of gas exchange. |
• | Both the bronchi and bronchioles have smooth muscle cells in their walls and are lined with mucus-secreting ciliated epithelium. |
There are 23 branching generations of airways from the trachea to the alveoli: | |
a) | conducting zone (generations 1-16) – where no gas exchange occurs; |
b) | respiratory zone (generations 17-23) – where gas exchange occurs and comprises the respiratory bronchioles, alveolar ducts and alveolar sacs. |
• | As the generation of branching increases, the: |
a) | radius of each airway reduces; |
b) | total cross-sectional area of the airways increases; |
c) | velocity of air flowing through the airway reduces. |
Figure 1. Central airway structures.
• | A pulmonary acinus represents a respiratory bronchiole and all of its branches. |
• | In both lungs, there are approximately 300 million alveoli, each of which have a diameter of 100-300µm. |
• | Each alveolus is surrounded by approximately 1000 capillaries and each capillary is in contact with several alveoli, so the capillaries can present a sheet of blood to the alveolar air for gas exchange (Figure 2). |
Figure 2. Peripheral airway structures.
3 | Describe the physiology of the pleural space (Figure 3) |
• | The pleura is a double-layered serous membrane that consists of: |
a) | parietal pleura (outer layer) – which lines the thoracic wall; |
b) | visceral pleura (inner layer) – which covers the lungs. |
• | The two layers are separated by the intrapleural space, which contains a thin film of pleural fluid that ensures the two pleural layers are tightly adherent to each other due to surface tension. |
• | This creates a negative pressure during inspiration and prevents the lungs from collapsing. |
Figure 3. Pleural membranes.
4 | What is the function of the airways? |
• | Gas exchange – by acting as a conduit for the passage of gases. |
• | Warm and humidify air – by saturating the air with water vapour before it reaches the alveoli. |
• | Filter – protecting the lungs from particulate matter by: |
a) | nasal hairs; |
b) | mucociliary escalator – particles, including bacteria, are trapped by the mucus lining of the airway and transported by the continuous movement of the ciliated epithelium back towards the larynx, where they then can be removed by expectoration or ingestion. |
What factors influence airflow during breathing? | |
• | The flow of air depends on the pressure gradient and the resistance of the airways, and is governed by the formula: |
• | Airway resistance is usually very low, so small pressure gradients lead to air movement during ventilation. |
• | Smooth muscle cells contained in the walls of the airways can contribute largely to airway resistance and are regulated by the autonomic nervous system: |
a) | adrenergic sympathetic fibres producing bronchodilation; |
b) | cholinergic parasympathetic fibres producing bronchoconstriction. |
6 | What is transpulmonary pressure? |
• | Transpulmonary pressure (Ptp) represents the difference between the alveolar pressure (Palv), which is equal to atmospheric pressure, and intrapleural pressure (Pip) during ventilation |
Ptp = Palv – Pip | |
• | The net difference between the two pressures determines whether the lungs have a tendency to inflate or deflate. |
• | Since atmospheric pressure is relatively constant, only variations of intrapleural pressure allow air to flow between the atmosphere and the alveoli. |
• | In situations where the transpulmonary pressure becomes zero, the lungs collapse as a result of their inherent elastic recoil and therefore there is no movement of air, such as when: |
a) | air enters the intrapleural space (pneumothorax); |
b) | the lungs are removed from the chest cavity (lung transplantation). |
• | During normal inspiration, the outward movement of the chest wall and diaphragm generate a relatively large negative intrapleural pressure (approximately -4mmHg) so that the transpulmonary pressure is 4mmHg. As a result, air moves towards the alveoli. |
During normal expiration, the intrapleural pressure increases slightly as a result of inward movement of the chest wall and diaphragm, and elastic recoil of the parenchyma, thus the transpulmonary gradient reduces whilst the alveolar pressure moves from slightly negative to slightly positive. As a result, air moves away from the alveoli. | |
• | Under physiological conditions, the transpulmonary pressure is always positive and equal or greater than the force of the elastic recoil of the lung parenchyma, thereby acting as distending pressure to the airways and alveoli, to keep the lungs fully inflated. |
7 | What is lung compliance? |
• | Lung compliance is defined as the volume change (∆V) per unit of pressure change (∆P) across the lung and represents the ease with which the lungs can be inflated (Figure 4). |
• | Normal compliance is 100mL/cm H2O. |
• | Reduced pulmonary compliance, such as in patients with fibrotic lung disease, represents a stiff lung, where extra work is required to expand the lungs during inspiration and move the same volume of air (Figure 5). |
• | Increased pulmonary compliance, such as in patients with emphysema, represents a lung where the elastic tissue has been damaged, usually due to overstretching by chronic over-inflation. As recoil function is decreased, extra work is required to move the same volumes of air during expiration. |
Figure 4. Pulmonary compliance.
Figure 5. Pulmonary compliance in normal and pathological states.
8 | What is surfactant? |
• | Surfactant is a surface-active lipoprotein complex (phospholipoprotein) formed by Type II alveolar cells (Figure 6) with hydrophilic head and hydrophobic tail regions. |
Figure 6. Alveolus with Type I and II alveolar cells.
9 | What are the functions of surfactant? |
• | Prevents alveolar instability – surfactant is present in all alveoli and acts to reduce the surface tension. As the concentration of surfactant is higher in smaller alveoli, it ensures that smaller alveoli do not collapse, as the distending pressure of the alveoli is directly proportional to the tension in the wall of the alveoli and inversely proportional to its radius (Laplace’s law, Figure 7). |
Figure 7. The effect of Laplace’s law on the alveoli.
• | Immune function – surfactant binds to sugars on the surface of pathogens and thereby opsonizes them for uptake by phagocytes. Surfactant also interacts with the adaptive immune response and regulates inflammatory responses. |
• | Regulating pulmonary compliance – the internal surface of the alveolus is covered with a thin coat of fluid. The water in this fluid has a high surface tension and provides a force that could collapse the alveolus. The presence of surfactant in this fluid breaks up the surface tension of water, allowing low transpulmonary pressure gradients to distend the alveoli during inspiration. |
10 | What is the definition of minute volume? |
• | Minute volume (MV) is the volume of gas inhaled (inhaled minute volume) or exhaled (exhaled minute volume) every minute. |
• | This can be calculated as a product of the respiratory rate (RR) and tidal volume (TV): |
MV = RR x TV |
11 | What is the mechanism of respiration (Figure 8)? |
• | Inspiration is an active process during which the thoracic volume is augmented by: |
a) | downward movement of the diaphragm, which increases the vertical height of the thoracic cavity; |
b) | contraction of the external intercostal muscles, which raises the ribs to a more horizontal position, thereby increasing the width of the thoracic cavity. |
• | This process leads to a fall of intrapleural pressure from -5cmH2O at the end of expiration to -9cmH2O at the end of inspiration. The consequent rise in the distending pressure across the wall of the alveoli (transpulmonary pressure) causes the lung to expand. |
• | Expiration is a passive process and depends on the elastic recoil properties of the stretched lungs as the inspiratory muscles relax. Elastic recoil generates a positive intra-alveolar pressure, which drives air out of the lungs. |
Figure 8. Active process of inspiration and passive process of expiration.
12 | What is the neurological control of respiration? |
• | Ventilation is normally regulated by involuntary neural mechanisms. |
• | Inspiration is activated by neurons in the medulla, which demonstrate spontaneous rhythmic activity separated by periods of inactivity. |
• | Motor neurons transmit from the spinal cord to the diaphragm and the external intercostal muscles leading to contraction during inspiration. |
• | Passive expiration occurs during the inspiratory neuronal refractory period when the diaphragm and intercostal muscles relax. |
• | During episodes of increased ventilation involving forced or active expiration, the expiratory neurons, also located in the medulla, are activated resulting in contraction of internal intercostal and abdominal muscles. |
• | Respiratory cells in the pons can modify the pattern of breathing by stimulating the pneumotaxic centre to inhibit the inspiratory neurons and shorten inspiration. |
What is dead space? | |
• | Dead space is defined as the part of the tidal volume that does not take part in gas exchange because it remains in the conducting airways or in alveoli that are poorly perfused. It is composed of: |
a) | anatomical dead space (approximately 150mL) – which is the total volume of the conducting airways from the nose or mouth down to the level of the terminal bronchioles. The anatomical dead space fills with inspired air at the end of each inspiration but this air is exhaled unchanged; |
b) | alveolar (physiological) dead space – which represents the alveoli that are well ventilated but poorly perfused and therefore less efficient at exchanging gas with the blood. |
• | In healthy individuals, the anatomical and physiologic dead spaces are roughly equivalent, since all areas of the lung are well perfused. In disease states, however, where portions of the lung are poorly perfused, the physiologic dead space may be considerably larger than the anatomic dead space. |
• | Physiological dead space is clinically more useful than anatomical dead space. |
14 | What is the ventilation-perfusion ratio? |
• | The alveoli should be adequately ventilated and perfused for proper gas exchange. |
• | The relationship between ventilation (V) and perfusion (Q) can be quantified by the V/Q ratio. |
15 | What is an intrapulmonary shunt? |
• | An intrapulmonary shunt refers to an area in the lung where perfusion exceeds ventilation, causing part of the cardiac output to return to the left heart without being exposed to ventilated alveoli, resulting in lower arterial oxygen levels (PaO2) and higher arterial carbon dioxide levels (PaCO2). |
• | The O2 content of mixed arterial blood is determined by the proportion of the blood that reaches ventilated alveoli compared to blood that bypasses ventilated alveoli. |
Physiological shunting (2-4% of blood) occurs when blood from the bronchial veins drains directly into the left ventricle without undergoing gas exchange via the lung, resulting in an arterial PaO2 slightly lower than the alveolar PAO2. | |
• | Pathological shunting occurs in patients with: |
a) | reduced alveolar ventilation, secondary to pulmonary oedema, severe pneumonia (with mucus retention) or atelectasis; |
b) | congenital pulmonary arteriovenous malformations (AVMs) – where blood bypasses the pulmonary capillary circulation and therefore is diverted away from the alveoli. |
16 | What is ventilation-perfusion (V/Q) mismatch (Figure 9)? |
• | V/Q mismatch refers to any deviation from the normal V/Q ratio, such as: |
a) | reduced V/Q (increased intrapulmonary shunt) – where an area of the lung is well perfused but not ventilated, such as in patients with pneumonia or pulmonary oedema, which will lead to a reduced PaO2 and increased PaCO2; |
b) | increased V/Q (increased physiological dead space) – when the alveoli are adequately ventilated but not perfused, such as in patients with a pulmonary embolus, which will lead to an increased alveolar PAO2 and decreased arterial PaO2. |
Figure 9. V/Q ratio in: A) reduced ventilation; B) normal conditions; and C) reduced perfusion.
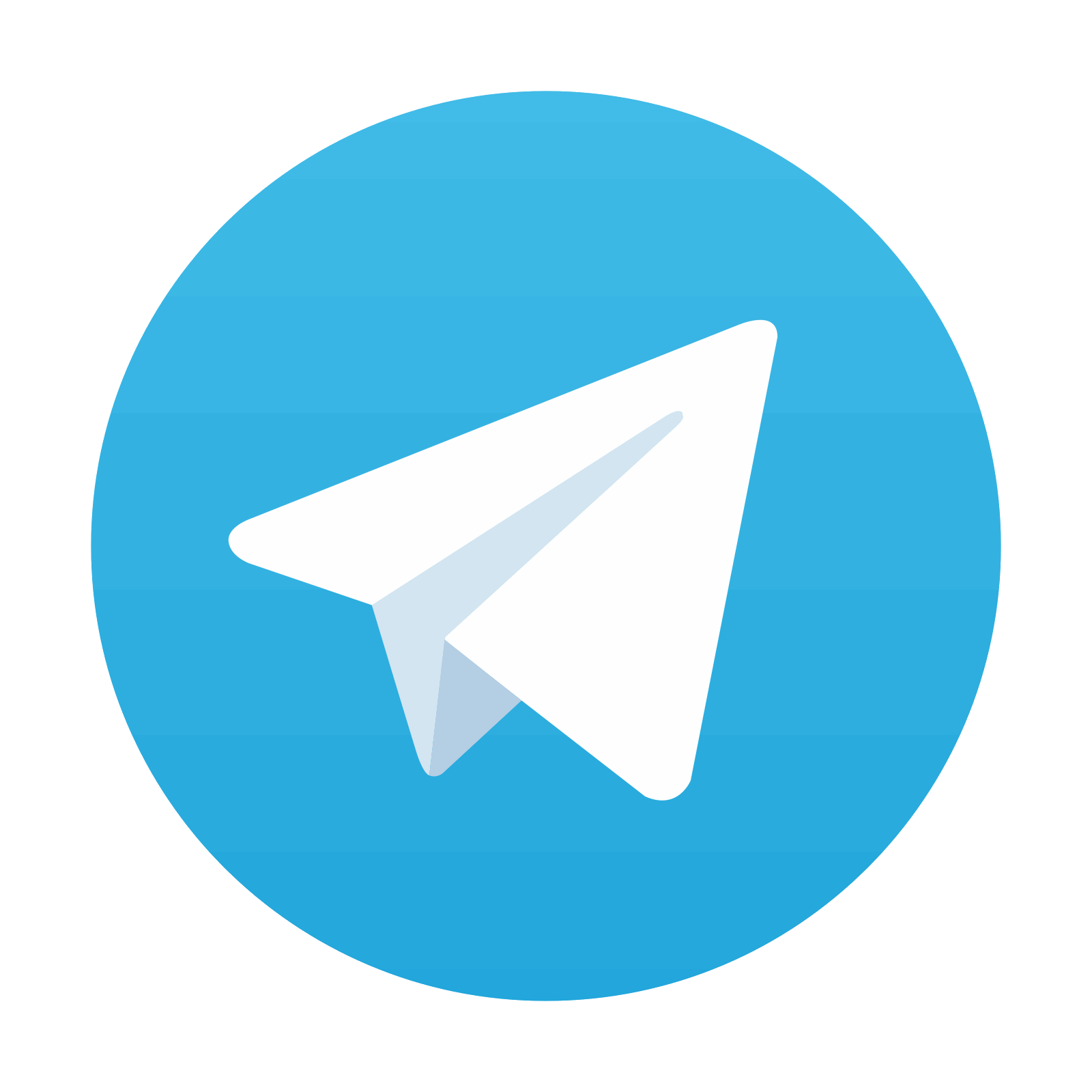
Stay updated, free articles. Join our Telegram channel

Full access? Get Clinical Tree
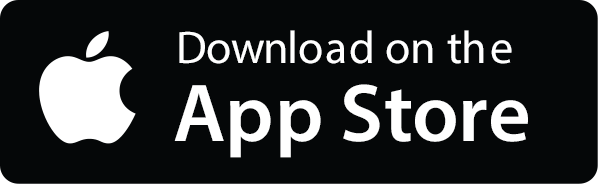
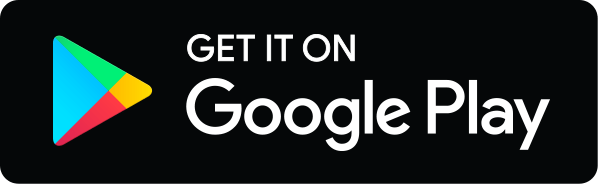