© Springer International Publishing Switzerland 2017
Massimo Fioranelli (ed.)Integrative Cardiology10.1007/978-3-319-40010-5_33. Low-Dose Therapy for the Treatment of Low-Grade Chronic Inflammation
Massimo Fioranelli1 , Marco Del Prete2, Jahaira Carolina Aracena3, Maria Grazia Roccia4, Carlo Dal Lin5 and Claudio Tomella3
(1)
Department of Nuclear Physics, Sub-nuclear and Radiation, Guglielmo Marconi University, Rome, Italy
(2)
Comitato Scientifico Amiot, Guglielmo Marconi University, Rome, Italy
(3)
Guglielmo Marconi University, Rome, Italy
(4)
University B.I.S. Group of Institutions, Punjab Technical University, Punjab, India
(5)
Department of Cardiological, Thoracic and Vascular Sciences, Padua University Medical School, Padova, Italy
3.1 Introduction
From a macroscopic point of view, the inflammatory process is considered to be an event for which it is possible to identify a beginning and an end, typically represented by the appearance and subsequent disappearance of the classical signs and symptoms (rubor, tumor, calor, dolor, and functio laesa) respectively [1, 2].
However, there is a subacute form of inflammation not characterized by the classic symptoms; it may be secondary to an acute inflammatory event or exist per se as a result of a slow and progressive alteration of physiological homeostasis.
It should never be forgotten that inflammation is a defense mechanism of the physiological organism, and is activated whenever it proves necessary, to eliminate a pathogen, for example, or in a psycho-neuro-endocrine-immunology (PNEI) vision, the elimination of homotoxins not expelled through the excretory organs.
Dr HH Reckeweg, in the 1940s, pictured the inflammatory phenomena as “the sacred fire by which the body burns toxins”; the impossibility of the resolution of the physiological inflammatory process inevitably leads the body to search for a new homeostatic (but pathological) balance characterized by the activation of a low-intensity chronic inflammatory response: low-grade chronic inflammation (LGCI).
From the point of view of inflammatory and immune homeostasis, LGCI can be described as the gradual and slow move toward a state of equilibrium in a pro-inflammatory one, marked by an increase in plasma levels of Th1/Th17-derived cytokines such as interleukins (ILs)-6; -1β; -17 (autoimmunity), and tumor necrosis factor-alpha (TNF-α). The increasing concentration of these factors leads to the progressive reduction of organ function, to the reduction of the efficiency of the clearing systems (difficulty in maintaining PNEI homeostasis), and to a higher incidence of inflammation-related pathological conditions [3, 4].
One physiological process is closely reminiscent of LGCI: the aging phenomenon [5, 6]. The parameters cited are also typical markers of aging; in particular, the time course of the IL-6 plasma level is a key factor for following the performance of both the physiological aging is of LGCI, as further proof of the close connection between the two biological events.
Under conditions of healthy aging, IL-6 increases, following a nonlinear fashion, starting a slow climb near 50 years of age and reaching, in an exponential fashion, high values in centenarians; the maintenance of this physiological progression curve depicts “physiological aging” [7].
The main effect of LGCI is to anticipate the exponential phase of IL-6 growth. This results in premature aging, which accelerates the progressive impairment of organ function.
3.2 Gastrointestinal Homeostasis, Microbiota Composition and Low-Grade Chronic Inflammation
Looking at the impressive list of stressors responsible for LGCI [8–15] onset, it is clear that any organ, system, or apparatus is “immune” from the effects of chronic inflammation; however, the gastrointestinal tract is the most “sensitive” to alterations of homeostasis induced by proinflammatory triggers.
Diet, lifestyle, stress, and drugs have significant effects on the gastrointestinal tract.
At the level of the intestinal mucosa, eukaryotic and prokaryotic cells (microbiota) live closely and interact. Today, it is no longer possible to think of the human body without neglecting the relationship between it and the microbiota; thus, it is a superorganism [16, 17]. This microcosm, a real eco system, is able to maintain a homeostatic physiological condition through cellular and molecular interactions, finely regulated by the nervous and endocrine systems and in the maintenance of an adequate immune response, rapid, efficient, and intense so as to lead to the resolution of perturbing events without affecting the morphological and functional integrity of the structure in which it takes place. The gastrointestinal tract is characterized as a PNEI system and plays the role of homeostatic controller, not only of its own relevant compartment, but of the whole organism [18, 19].
The intestinal mucosa is able to secrete neuropeptides, neurohormones, hormones, and cytokines, carrying out nervous, endocrine, and immune functions and it is closely connected to other organs, systems, and apparatuses [20, 21]. As a result of these interactions, it is evident that the presence of a state of physiological inflammation is a normal phenomenon in the intestine, a real “function” inserted inside the mechanisms of PNEI homeostasis. The intestinal mucosa is constantly exposed to a massive antigen load represented by the commensal bacterial flora, also named microbiota. The tolerance of the microbiota is central to the maintenance of physiological inflammation in the intestine [20].
Quantitative and qualitative alterations of the microbiota may be a trigger for the onset of diseases characterized by local changes in permeability of the mucosa, such as inflammatory bowel disease (IBD), irritable bowel syndrome (IBS), gluten sensitivity, and leaky gut syndrome, but also extraintestinal diseases, such as autism spectrum disorders, the anxious–depressive syndromes, Alzheimer’s disease, type II diabetes, obesity, psoriasis, rheumatoid arthritis, chronic obstructive pulmonary diseases (COPDs), or recurrent respiratory infections (IRRs).
3.3 Cardiovascular Risk Factors and Low-Grade Chronic Inflammation
High levels of circulating lipids (specifically, high low-density lipoprotein (LDL) and low high-density lipoprotein levels) and triglycerides, obesity, hypertension, and hyperglycemia/diabetes are generally considered the best predictive parameters for the calculation of cardiovascular risk [32–34].
The combination of the above-mentioned risk factors with some anthropometric parameters (gender, age, etc.) is mathematically interpreted and creates a numerical score used to determine the position of the subject being analyzed on the risk scale. But what are the biological mechanisms underlying the events mechanically interpreted by mathematical functions? The mechanism of LGCI is the joining link between all the cardiovascular risk factors representing the biological response to the perturbation of continuous homeostasis triggered by multiple stressors.
There are many pro-inflammatory triggers involved in LGCI onset, affecting virtually every aspect of an individual’s life: Western diet; chronic stress; overweight and obesity; genetically modified organisms; environmental pollutants; drug abuse or misuse; and an inappropriate lifestyle [8–15].
The cardiovascular system, like every other tissue, organ, or system, is subject to alterations of its physiological functions due to the loss of the conditions needed for homeostasis.
Acute and especially chronic inflammatory (LGCI) conditions are potent triggers of homeostatic alterations and have an effect on cardiovascular function; every cell population, from myocardiocytes to the endothelial cells of the vessels, is therefore influenced by inflammatory mediators [35, 36].
Heart failure, heart attacks, atherosclerosis, and other cardiovascular diseases have an inflammatory component of considerable importance. In fact, among the etiological factors that cause the onset is inflammation of the myocardium.
Myocardial inflammation is secondary to a large number of triggers including infections (bacterial myocarditis), trauma mechanics, hemodynamic stress, hypertension, and not least, high levels of pro-inflammatory cytokines.
A deeper understanding of the inflammatory mechanism and the role of the specific markers C-reactive protein (CRP), IL-1, IL-6, and TNF-α [37–39] underlying the pathogenesis of chronic cardiac diseases is fundamental to the correct management of cardiovascular disease (CVD).
C-reactive protein is a key element of the inflammatory response; it is indispensable for the activation of the complement and as a phagocytosis-stimulating factor.
However, it is also one of the main events triggering inflammation that affects the blood vessel wall; CRP levels ≥2.4 mg/L are at high risk of CVD.
C-reactive protein is a typical acute phase reactant. It reaches a peak concentration at 48 h after inflammation onset (50,000 times higher than baseline). Its presence at a vascular level is indicative of great endothelial chronic inflammation, a fundamental factor for the triggering of atherogenic phenomena.
The importance of CRP as a marker of the individual risk of CVD has recently been highlighted by evidence that statins are able to reduce fatality rates in individuals who have a normal LDL cholesterol concentration, but increased CRP concentration, as statins directly decrease the CRP concentration.
Under IL-6 stimulation CRP is produced in the liver; macrophages are supposed to produce more IL-6 after different types of injury (infection, injury, etc.), but, interestingly, elevated levels of CRP are also detected in depressive individuals. CRP levels are completely independent of other factors linked to CRP concentration, leading to the hypothesis that a depressive mood is independent of an increase in inflammatory mediators, and, consequently, it is probably the key reason for the increased risk of CVD in depressive individuals. This interaction between a psychological disease and an increased inflammatory status clearly proves that both physiological and pathological inflammation constitute PNEI events, highlighting that alteration of the homeostatic balance (independently of the nature of the perturbing agent) has profound effects on the whole body.
Patients with chronic inflammatory diseases (e.g., rheumatoid arthritis or IBDs) are at a higher risk of CVD and related mortality. Cardiovascular risk factors are obviously a key trigger for CVD onset, but LGCI, which is also independently involved, accelerates atherosclerosis, myocardial infarction, cerebrovascular disease, and heart failure (HF). TNF-α and IL-1 could be major actors in this pathophysiology [40].
The inflammatory cytokines, IL-1α and IL-1β, are the master mediators of both local and systemic inflammatory responses underlying a broad spectrum of diseases that clearly includes CVD.
Atherosclerosis and the other CVDs have a significant inflammatory component in their etiology, and hyperlipidemia is also one of the main risk factors for hypertension and diabetes. Macrophages generate pro-inflammatory cytokines such as IL-1 and TNF-α and are deeply involved in atherosclerosis, in addition to mast cells, which generate several other cytokines (IL-6 and IFN-γ) and chemokines (MCP-1 and RANTES) [41] involved in monocyte recruitment and differentiation in the arterial endothelium.
3.4 The Prognostic Role of IL-6 in LGCI and Related Cardiovascular and Kidney Diseases
As mentioned above, IL-6 levels correlate with some of the traditional CVD risk factors [42]. Normally, IL-6 increases with age and its levels are directly associated with high blood pressure, smoking, and insulin resistance [43].
The synthesis of IL-6 increases in parallel with increasing levels of adiposity in healthy men and women. Indeed, about one-third of the total IL-6 circulating level originates from adipose tissue and this is a clear example of the direct correlation between obesity and LGCI.
Anticipating the onset of age-related diseases, IL-6 is a trigger for cardiovascular disease per se [44], but, consistent with its role in inflammatory diseases, it is also one of the best markers for risk assessment by virtue of its dependence on or independence from classic risk factors.
Analysis of variance between the normal increasing rate of IL-6 and an altered rate can be predictive of LGCI status, even in apparently healthy subjects, intensifying the screening for CVD risk and unlinking it from classical risk factors.
Inflammation also represents the link between CVDs and chronic kidney diseases (CKD). CVDs are common in patients affected by CKD and also end-stage renal disease (ESRD) [47]. Cardiovascular mortality and total mortality are 10- to 100-fold higher in ESRD patients than in age-matched controls [48, 49]. Indeed, the risk of death after a cardiovascular fatal event in a 30-year-old ESRD subject is comparable to that of a healthy 80-year-old subject. IL-6 is one of the best inflammation markers because of its high predictive value for outcome evaluation in ESRD [49].
3.5 LGCI, Gut–Liver Axis and PNEI Homeostasis Breakdown
The LGCI constitutes the point of contact between all the pathological manifestations of the loss of PNEI homeostasis. The gastrointestinal system participates in a decisive way in the establishment and the functioning of the PNEI network; it is the key in two axes to the maintenance of the gut–brain axis and the gut–liver axis [50, 51].
Any disturbance in the intestine (alterations of the structure, function, and microbiota) affects P.N.E.I. homeostasis with alterations of immune, endocrine, neuroendocrine, and hormone responses.
As already mentioned, the loss of intestinal functional balance has as the primary result the alteration of immunological tolerance against both the microbiota and the food antigens. This results in the shift from physiological to pathological inflammation. Continuous exposure to proinflammatory triggers reduces intestinal homeostasis recovery and the continuation of this condition leads to the appearance of LGCI.
There is ample evidence for the close correlation between the alteration of the microbiota and the intestinal function and chronic liver, kidney, and cardiovascular diseases [52, 53].
Actually, the metabolic syndrome (MS) is increasingly seen as a fundamental causative agent for liver function abnormalities [54]; in particular, there is a direct link between MS and that set of liver diseases (not related to viral infections or alcohol consumption) that are referred to as non-alcoholic fatty liver disease (NFLD) [55]. Faced with this evidence, numerous studies have shown that NFLD and CVD are closely linked by common pathophysiological aspects [56]: high oxidative stress, LGCI, and atherogenic dyslipidemia [57, 58]. A further etiogenetic component of NFLD is apparently not related to those cited: the alteration of the intestinal microbiota [59, 60]. In fact, we have already seen how quantitative changes in the intestinal bacterial population constitute a powerful pro-inflammatory trigger; the resulting LGCI returns this factor into the set of common factors in NFLD and CVD.
In summary, all phenomena of altered intestinal homeostasis are potential triggers able to change the whole endocrinological, immunological, and hormonal organism. The LGCI is the unsuccessful attempt by the body to recover a homeostatic balance, and its major mediators IL-1, TNF-α, IL-6, and CRP are involved, in various ways, in the development and maintenance of all the inflammation-based diseases.
3.6 The Cellular Target of LGCI: Impaired Mitochondrial Activity and Reflexes in Cardiovascular Dysfunction
The mitochondria are a natural source of reactive oxygen species (ROS) [61], which are physiologically generated during the processes of mitochondrial respiration. Low levels of ROS are therefore physiological and fundamental for the correct operation of the electron transport chain [62, 63]; redox homeostasis is achieved through protection mechanisms managed by suitable radical scavengers, such as superoxide dismutase (SOD) and coenzyme Q10 (ubiquinone), which operates both as a carrier of electrons and as an antioxidant by exploiting the possibility of passing through partially reduced and reduced forms by accepting electrons.
An excessive presence of free radical species is universally known to be a major cause of CVD. Induced changes in the environment at a cardiovascular level by free radicals have long been studied; in particular, it has become apparent that the cytotoxic properties and atherogenic LDL cholesterol, in both native and oxidized form, are mediated by events of oxidative stress [64–67].
Low-grade chronic inflammation is a considerable source of oxidative stress caused by the subclinical elevation of proinflammatory mediators and consequent generalized alteration of cellular metabolic homeostasis. As is well known, oxidative stress, in its most typical expression is represented by an excess of ROS, and attacks the membranous structures of the cell and its genetic material. Mitochondria appear to be most sensitive to ROS subcellular organelles and numerous studies have shown that mitochondria are also particularly sensitive to the reactive nitrogen species (RNS) [68].
Mitochondrial oxidative phosphorylation is impaired by an excess of free radicals that act as inhibitors of electron transport at a mitochondrial inner membrane level. In particular, NO radicals block the reduction of molecular oxygen (O2) to form H2O at complex IV level [69] (a part of the electron transport chain formed by a series of multisubunit protein complexes). Physiologically, 2–4 % of electrons escape the oxidative chain forming ROS; the presence of free radicals increases this percentage, inducing excessive oxidative stress and the biological result consists in increased levels of cellular damage, dysfunction, and consequently apoptosis. Hence, fluctuations of ROS/RNS levels heavily influence organelle functions, targeting membranes and mitochondrial DNA (mtDNA) [70]. Mitochondrial damage primarily impairs the ability of cells to produce energy and also alters many cel lular functions based on redox signaling pathways.
At the cardiovascular level, oxidative stress-induced mitochondrial damage is a crucial event in the onset of CVD [71, 72]. CVD patients show a high level of mtDNA degradation [73] (both deletions and sequence abnormalities) in both the heart and aorta compared with healthy subjects. It is clear that myocardiocytes and vascular endothelial cells are key targets for oxidative stress and the impaired mitochondrial functions in these cells take part in disease onset [66, 74].
Redox balance is fundamental to the maintenance of cardiovascular homeostasis. Impaired ROS scavenger synthesis at the cardiac level is linked to reduced resistance to ischemia/reperfusion damage; decreased expression of the specific superoxide dismutase 2 (SOD2) in vascular endothelium is directly linked to an increased risk of CVD [75, 76].
All main CVD risk factors share one feature: increased oxidative stress, which is the concomitant result and trigger for LGCI present in atherosclerosis, hypercholesterolemia, and diabetes; tobacco smoke-related cardiomyopathy is related to mitochondrial dysfunction and mtDNA damage at the aortic endothelium level.
The connection among oxidative stress, inflammation, and CVD risk is increasingly evident and the example of diabetes is typical. Hyperglycemia is a powerful trigger for ROS generation and, on the contrary, mitochondrial SOD2 is able to prevent hyperglycemia-associated ROS production and block NF-kB activation.
Loss of glucose homeostasis in diabetes results in increased ROS and reduced SOD2 production; loss of NF-kB translocation control results in a 2-/3-fold increase in its activation and consequently in the overexpression of pro-inflammatory mediators such as IL-1β, TNF-α, and IFN-γ. A vicious circle is established among altered homeostasis, inflammation, and oxidative stress; this results in LGCI and a progressively increasing risk of CVD.
At the mitochondrial level, a chronic inflammatory state and high oxidative stress reduce the production of energy. Coenzymatic systems are particularly susceptible to damage from ROS and inflammation. Mitochondrial coenzyme Q10 is essential for the management of the phases of aerobic ATP production [77, 78] (95% of the ATP product comes from the aerobic phase) as a basic component of the electron transport chain. The high transport capacity of electrons makes Q10 of one of the main scavengers of radical species, thus performing a fundamental antioxidant action capable of maintaining the redox equilibrium at the mitochondrial level [79].
The increased levels of oxidative stress by LGCI eventually lead to the exhaustion of buffering antioxidant systems [64]. It is significant to note that in the presence of metabolic syndrome levels of Q10, higher than the average values of a healthy subject are detected. This phenomenon describes the attempt by the body to increase its capacity to buffer the redox imbalance, but also highlights the failure of this protective mechanism: at a cardiovascular level, the loss of the ability of redox regulation results in a decline in mitochondrial function, which translates into progressive congestive heart failure (CHF).
Low-grade chronic inflammation also affects vascular endothelial functions [64, 80]. Physiological aging and pathological LGCI exert a detrimental action on endothelial cells, increasing mitochondrial oxidative stress and mitochondrial DNA damage [81]. LGCI generates an overproduction of ROS, which impairs the vasodilatory activity of nitric oxide (NO) and increases the formation of the peroxynitrite ONOO–, a dangerous free radical [64].
In addition, a reduced antioxidant response mediated by erythroid-2-related factor-2 (Nrf2) and downregulation of SOD2 contribute to the induction of a chronic pro-oxidative microenvironment in chronically inflamed vessels [64].
NF-kB is sensitive to redox imbalance and it is upregulated in endothelial cells from LGCI-affected subjects. NF-kB drives a proinflammatory shift that positively feedbacks oxidative stress and its chronic activation is due to upregulated angiotensin-II signaling and impaired sirtuin expression; these events reduce the cellular protective response against acute ROS generation. A major source of damage comes from the imbalanced production of ROS. Mitochondrial ROS (mtROS) prejudice both mitochondrial structures and biogenesis through redox alteration of macromolecules, such as mitochondrial DNA (mtDNA). Structural and functional deterioration of mitochondria plays a fundamental role in cardiovascular diseases, driving both cardiac failure and vascular atherosclerotic events.
MtROS homeostasis is fundamental for the maintenance of the physiological function of mitochondria and an optimal level of oxidative stress is required [82, 83]. ROS exert a biphasic action on mitochondria metabolism: excessive production of ROS (as previously stated) impairs mitochondrial activity, but extremely low levels of oxidative stress also have negative effects on mitochondrial homeostasis.
Pharmacological or nutritional (supplementation) intervention with massive doses of antioxidant agents may impair mitochondrial functionality by blocking the respiratory electron chain and, consequently, energy production.
In the presence of cardiovascular risk with reduced cardiac and vascular functions and impaired hemodynamic homeostasis, the sustenance and/or recovery of mitochondrial redox homeostasis is important within a correct anti-inflammatory strategy.
The redox imbalance could lead to increased lipid peroxidation (including oxLDL), boosting LGCI-induced atherosclerosis; the approach to reducing this imbalance is based on the administration of antioxidants such as vitamins C and E and beta-carotene and coenzyme Q; the latter is considered the more active against lipid peroxidation [84, 85].
It is important to note that high-dose treatment with statins, one of the most important classes of anti-hyperlipidemic drugs used for primary and secondary CVD prevention, decreases plasma coenzyme Q10 concentrations with deleterious effects on cardiac function owing to muscular damage induced by the impaired mitochondrial activity.
3.7 Low-Dose Therapy for the Treatment of LGCI
Actually, there are no protocols for the treatment of chronic inflammation (including LGCI) based on the use of specific synthetic drugs, but the chronic use of anti-inflammatory drugs designed for use in acute (e.g., NSAIDs) is frequent, with dramatic consequences in terms of side effects, especially on the cardiovascular [86, 87] (increased risk of nonfatal and fatal cardiovascular events, both in heart patients and in healthy individuals) and immune systems [88] (risk of immune deficiency and the consequent high susceptibility to bacterial/viral infections).
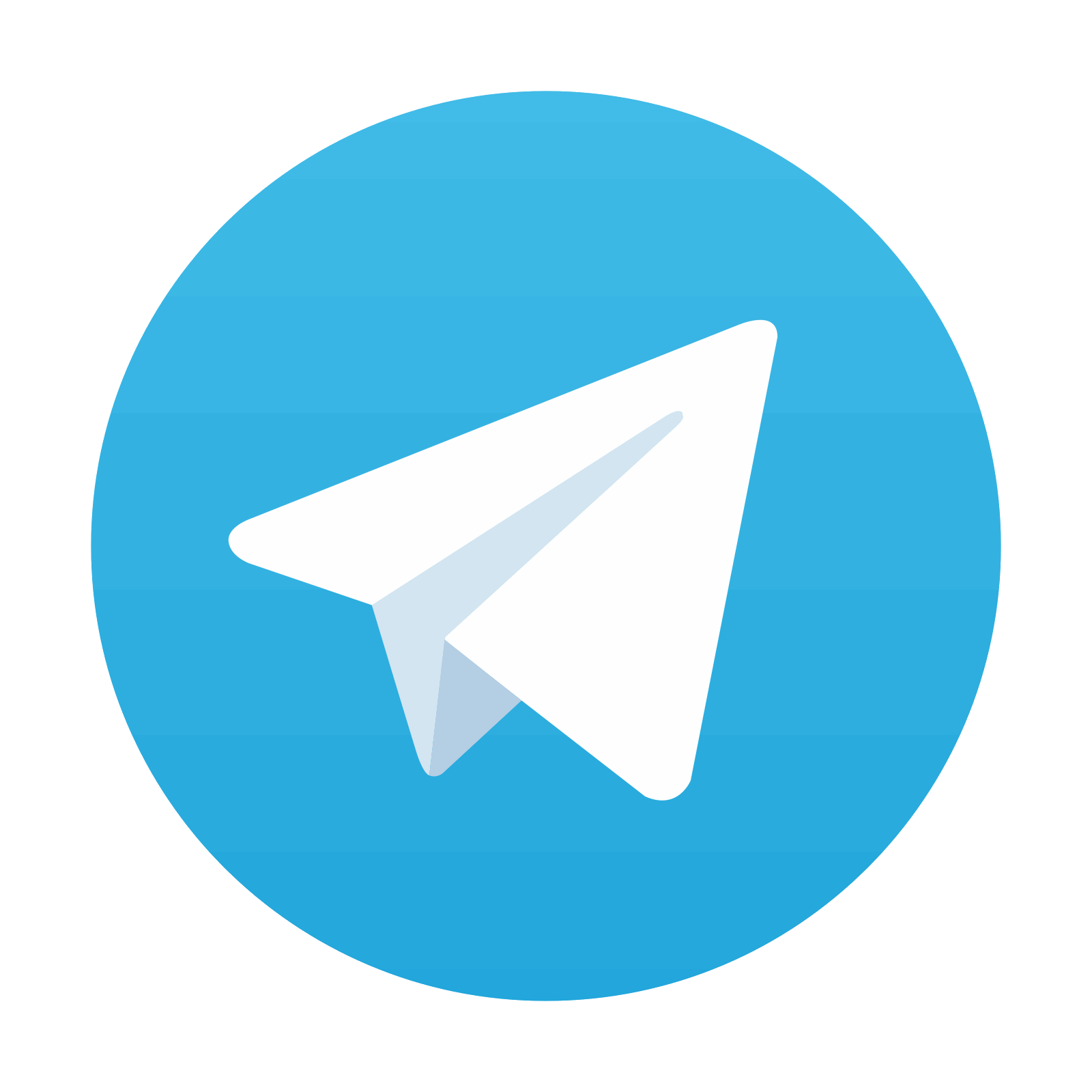
Stay updated, free articles. Join our Telegram channel

Full access? Get Clinical Tree
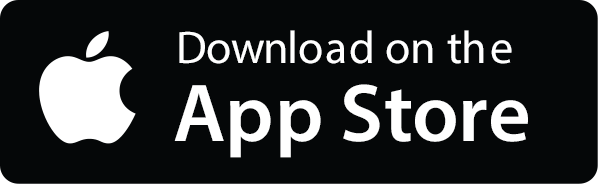
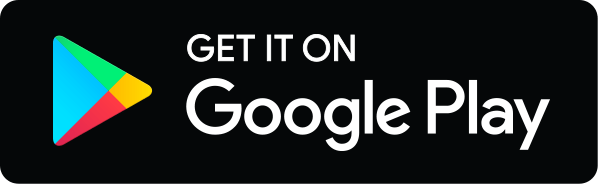