Background
Recent advances in very high frame rate use of ultrasonography have enabled the application of two-dimensional speckle-tracking echocardiography (STE) to small animal cardiac functional assessments. In this study, two-dimensional STE was applied to a rat model of hypertensive heart failure with preserved ejection fraction to clarify consequences of left ventricular (LV) wall deformation in the progression of heart failure with preserved ejection fraction.
Methods
STE was performed every 2 weeks in Dahl salt-sensitive rats fed a 0.3% (control group) or 8% (hypertension [HT] group) sodium chloride diet from 6 to 14 weeks of age. Longitudinal, radial, and circumferential global strain and strain rate were measured, and the time courses of these parameters were observed.
Results
Deterioration of longitudinal strain occurred in the early phase of the progression of LV hypertrophy and continued to worsen until congestive heart failure developed (longitudinal strain in the HT group: 25 ± 3% at 10 weeks, 21 ± 4% at 12 weeks, and 18 ± 2% at 14 weeks; longitudinal strain in controls was preserved during the experimental period). At 12 weeks, radial strain (HT group, 35 ± 7%; controls, 41 ± 10%) had deteriorated at the late stage of manifest diastolic dysfunction. Throughout the experiments, circumferential strain was preserved (HT group, 35 ± 6%; control group, 35 ± 5%), and no significant increase in short-axis function was observed.
Conclusions
STE is applicable to the small animal heart and detected LV wall long-axis dysfunction preceding short-axis dysfunction or overt congestive heart failure in the progression of hypertensive LV hypertrophy in a rat model.
Echocardiography is a widely available noninvasive tool for imaging cardiac structures and assessing performance. Because of its noninvasive nature, echocardiography is the best diagnostic tool with which to follow temporal changes from disease progression or regression. Two-dimensional (2D) speckle-tracking echocardiography (STE) is a newly developed technique that quantifies myocardial deformation by tracking the ultrasonographic motion of speckles throughout the cardiac cycle. Recent advances in speckle-tracking echocardiographic technology have enabled its application to the small animal cardiovascular disease model and allowed the identification of both ischemic left ventricular (LV) dysfunction induced by myocardial scarring and of other forms of LV dysfunction with elevated afterload.
The prognosis of heart failure may not be stratified simply by the LV ejection fraction (LVEF). Recently, deterioration of longitudinal strain (LS) obtained on STE has been reported in heart failure with preserved ejection fraction (HFpEF). In addition, the utility of long-axis dysfunction has been the focus in the prediction of the heart failure outcome. However, the time course of long-axis dysfunction in patients with HFpEF has not been fully studied. We hypothesized that a newly developed echocardiographic system could identify myocardial deformation of the rat heart serially and clarify how the various myocardial wall strains relate to hemodynamic findings with regard to the stage of diastolic dysfunction. A rat model of diastolic heart failure on the basis of hypertensive heart disease has been developed in the Dahl salt-sensitive rat.
Accordingly, the purpose of the present study was to apply high-resolution STE to a rat model with hypertension (HT) and to investigate the sequential longitudinal and short-axis functional changes that occur in the progression of hypertensive heart disease.
Methods
Experimental Animals
Animal experiments were carried out in a humane manner after we received approval for this study from the Institutional Animal Experiments Committee of the University of Tsukuba and were in accordance with the regulations for animal experiments of our university. In this study, 43 male Dahl salt-sensitive rats (DIS/Eis; Eisai, Tokyo, Japan) were studied. They were divided into two groups: the control group ( n = 21) was fed low-salt laboratory chow containing 0.3% sodium chloride, and the HT group ( n = 22) was fed high-salt laboratory chow containing 8% sodium chloride from 6 weeks of age. The rats were killed after catheterization at 12 or 14 weeks of age. The sample size calculation was performed on the basis of the following assumptions: at week 14, the difference in LS was 15% between the two groups, the standard deviation within the group was 10%, power was 0.80, and the significance level was .05. As a result, we calculated a required sample size of 10 rats in each group for the invasive hemodynamic test.
All rats were housed two to three rats per cage in an air-conditioned room at 25°C with an automatic 12-hour light-dark cycle and free access to chow in the Laboratory Animal Research Center at the University of Tsukuba. Blood pressure measurement using a tail cuff system (BP-98A; Softron, Tokyo, Japan) was performed every 2 weeks from 6 weeks of age.
Echocardiographic Studies
Echocardiographic studies were performed every 2 weeks from 6 weeks of age. For in vivo echocardiography, rats were first anesthetized using isoflurane at a concentration of 3% to 4% in a knockdown box. Once anesthetized, the rats were positioned on the platform ventral side up, and the chest was shaved. The concentration of isoflurane was then changed to 1.5% to 2% and adjusted to a dose adequate to maintain the heart rate at 300 to 350 beats/min during the imaging session. Cardiac image sequences were acquired using a Vevo2100 high-resolution ultrasound system (VisualSonics Inc., Toronto, ON, Canada) using a 13-MHz to 24-MHz linear transducer (MS-250). For speckle-tracking analysis, parasternal long-axis views, which contained the LV anterior and inferior wall from base to apex, and a short-axis view at the papillary muscle level were acquired, with attention to setting the minimal ultrasonic scanning range to obtain the 2D image with a high frame rate. M-mode images were recorded at the same level to obtain the anterior wall diastolic thickness and inferior wall diastolic thickness and LV end-diastolic diameter (LVDd) and LV end-systolic diameter. We defined the average of anterior wall and inferior wall diastolic thicknesses as the wall thickness. Conventional measurements of the left ventricle, which included endocardial fractional shortening, were calculated by measuring M-mode images as follows:
Fractional shortening ( % ) = [ ( LVDd − LV end-systolic diameter ) / LVDd ] × 100.
Relative wall thickness was calculated as follows:
Relative wall thickness = ( anterior wall diastolic thickness + inferior wall diastolic thickness ) / LVDd .
Fractional area change was calculated automatically by tracing end-diastolic epicardial and endocardial areas of short-axis B-mode loops:
Fractional area change ( % ) = [ ( end-diastolic areas − end-systolic areas ) / end-diastolic areas ] × 100.
Similarly, LV end-diastolic volume (LVEDV), LV end-systolic volume, LVEF, and LV mass were calculated automatically by tracing end-diastolic epicardial and endocardial areas of long-axis B-mode loops according to the following formulas:
LVEDV = { [ 7.0 / ( 2.4 + end-diastolic short-axis diameter ) ] × ( end-diastolic short-axis diameter ) 3 } ,
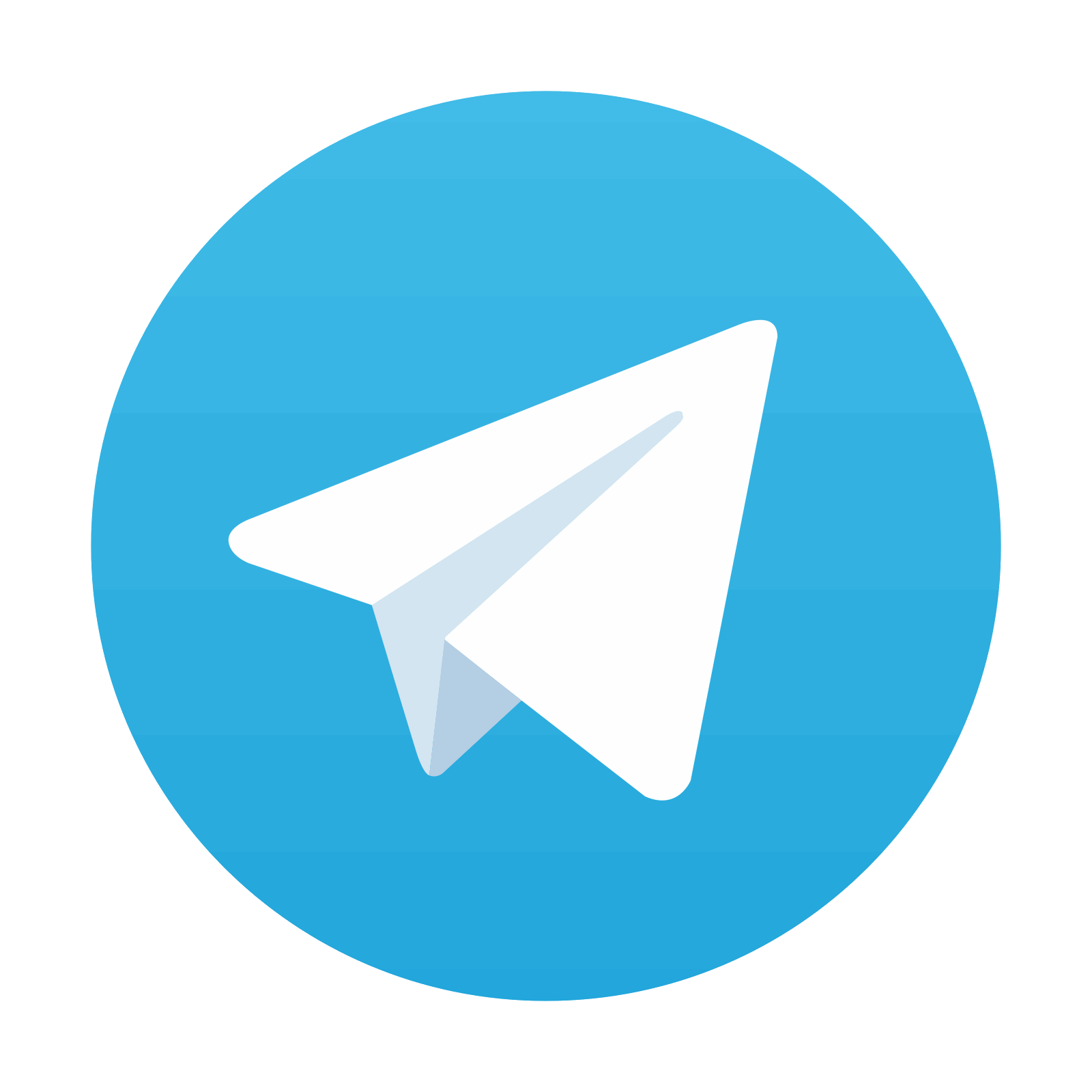
Stay updated, free articles. Join our Telegram channel

Full access? Get Clinical Tree
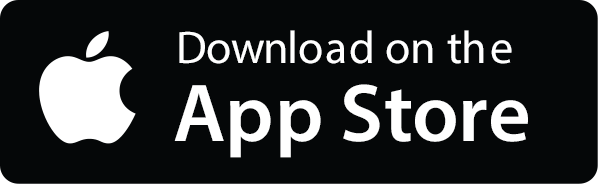
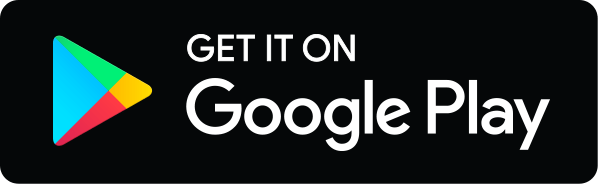