Background
Normative data for fetal myocardial deformation values have not been comprehensively described in a longitudinal cohort. The effect of gestational age on these values and on interobserver variability require further investigation.
Methods
Sixty gravid women were prospectively enrolled before 20 weeks’ gestation. The following measures were obtained by two blinded observers at five time points across gestation and also at 4 to 8 weeks’ postnatal age: global circumferential strain and strain rate, global longitudinal left ventricular strain and strain rate, global longitudinal right ventricular strain and strain rate, and left and right ventricular myocardial performance indices. Optimal myocardial visualization and frame rate (≥100 frames/sec) were ensured.
Results
For gestational age groups ≥24 weeks, intraclass correlation coefficients between observers were >0.70 for all measures and >0.85 for the majority of measures of myocardial deformation. At 20 to 21 weeks’ gestation, intraclass correlation coefficients were 0.35 to 0.52 for longitudinal measures and 0.74 to 0.82 for circumferential measures. Myocardial performance index intraclass correlation coefficients were <0.80 at all time points and <0.70 for most time points. Global longitudinal left ventricular strain and global circumferential strain values remained stable across gestational age groups. Global longitudinal right ventricular strain values remained stable across gestation and increased after birth. Global circumferential strain rate, global longitudinal left ventricular strain rate, and global longitudinal right ventricular strain rate decreased from 20 to 21 weeks’ gestation to the remainder of gestation and then remained stable until delivery. Upon delivery, global circumferential strain rate and global longitudinal left ventricular strain rate decreased, and global longitudinal right ventricular strain rate increased.
Conclusions
Interobserver variability of fetal strain and strain rate measured at ≥24 weeks’ gestation was lower in comparison with values obtained at 20 to 21 weeks’ gestation and lower in comparison with left ventricular and right ventricular myocardial performance indices using the described protocol. Gestational changes in fetal myocardial deformation values likely reflect changes in preload and/or afterload on the fetal heart.
Echocardiography has emerged as the mainstay of fetal cardiovascular assessment. The assessment of cardiac function is essential to the evaluation and/or treatment of several pregnancy-related complications, including twin-twin transfusion syndrome, high-output cardiac failure, gestational diabetes, growth restriction, nonimmune hydrops, anemia, cardiomyopathy, and structural heart diseases. However, objective methods to assess the severity of intrinsic fetal cardiac dysfunction have not been well validated. Traditional measures of fetal cardiac function include the myocardial performance index (MPI). The MPI has some utility in identifying cardiac dysfunction, but its use has been hampered by concerns about reliability of the measurement and poor correlation with clinical outcomes.
Myocardial deformation analysis (measurement of strain and strain rate) is a direct assessment of conformational change of the myocardium and has been used in adults and in children to prognosticate subclinical disease or diseases of questionable severity. Measures of myocardial deformation have been applied to the fetus, with promising results. However, similar to the MPI, these measures have also carried concerns about reproducibility. The lack of a robust longitudinally acquired normative data set and a paucity of data on circumferential strain and strain rate also limit clinical applicability. A novel method to measure myocardial deformation using cardiac events in lieu of an electrocardiographic signal has been described for the measurement of fetal strain and strain rate and used at a number of centers.
In the present investigation, we applied a standardized protocol to measure longitudinal and circumferential ventricular strain and strain rate in a prospectively enrolled, longitudinal cohort of normal fetuses across pregnancy and after delivery. We sought to compare interobserver variability of these measures at multiple gestational age time points, determine the effect of advancing gestational age on these measures, and report normative data for these measures across gestation and upon delivery.
Methods
Mothers who were undergoing routine obstetric ultrasonography at Texas Children’s Hospital Pavilion for Women were invited to enroll in this institutional review board–approved study at the time of the routine second-trimester ultrasound study. Informed consent was obtained, and participants were compensated for parking and time spent participating in the study. Criteria for inclusion in the study included acceptable visualization of the fetal heart, absence of cardiac abnormalities during the screening examination, and body mass index < 30 kg/m 2 . Only mothers in whom the fetal heart could be adequately visualized for clinical care on the routine second-trimester ultrasound study were offered participation in the study. The determination of whether the fetal heart was adequately visualized was made at the discretion of the staff obstetrician, as per clinical routine. Exclusion criteria included preexisting or history of gestational diabetes, maternal hypertension, growth restriction, thyroid disease, and other significant chronic disease or obstetric complication.
On the basis of previous research and our own preliminary data, the SD of global longitudinal left ventricular (LV) strain was expected to be <0.04 units (4%). Assuming SD = 0.04, a total sample size of 53 patients was chosen to obtain a 99% CI (α = 0.01). The type I error rate was assumed to be 0.01 for sample size considerations to allow multiple hypothesis testing and control the overall probably of a type I error. Additional patients were enrolled because of a presumed attrition rate of about 10%.
Image Acquisition and Analysis
Image acquisition for measuring fetal strain and strain rate was performed by one of two American Registry for Diagnostic Medical Sonography–certified fetal sonographers with ≥7 years’ experience performing fetal echocardiography. Image acquisition for measuring neonatal strain and strain rate was performed by one of two American Registry for Diagnostic Medical Sonography–certified pediatric cardiac sonographers. All fetal and postnatal images were obtained using a GE Vivid E9 ultrasound system (GE Healthcare, Little Chalfont, United Kingdom) using a 5- or 6-MHz phased-array probe and stored as raw data. Although it was possible to use the curvilinear probe, we found that the phased-array probes provided the best combination of acoustic signal and temporal resolution. Echocardiograms were obtained at the following time points for each fetus: 20 to 21 weeks’ gestation, 24 to 25 weeks’ gestation, 28 to 29 weeks’ gestation, 32 to 33 weeks’ gestation, 36 to 37 weeks’ gestation, and 4 to 8 weeks’ postnatal age, with particular attention paid to optimal spatial resolution and signal and with a minimum frame rate of 100 frames/sec. The 4- to 8-week postnatal age time frame was chosen to ensure transition to postnatal circulation. A larger window (4 weeks) was chosen to ensure compliance. Raw, uncompressed data were then sent to an independent workstation for analysis, which involved speckle-tracking for measurement of strain and strain rate (EchoPAC version 110.0.2; GE Healthcare). For each measure, in all study subjects, offline image analysis was performed by two examiners, each blinded to the results of the other: a pediatric cardiologist with expertise in fetal imaging (S.A.M.) and a sonographer with substantial experience in the measurement of myocardial deformation, who serves as the research sonographer for our group. To ensure adequate blinding, data from each observer were saved in a separate folder on a research server. Observers analyzed data obtained on the same acquisition and were free to choose which cardiac cycle in the particular acquisition to analyze.
In lieu of an available electrocardiogram, mechanical events were used to time the cardiac cycle. The time cursor typically used to mark the beginning of the QRS complex was placed at mitral valve closure ( Figure 1 A). Aortic valve (AV) opening and closure were manually identified on spectral Doppler interrogation of the AV ( Figure 1 B). The following measures of myocardial deformation were obtained: global longitudinal LV strain (GLSLV) and global longitudinal LV strain rate (GLSrLV), global longitudinal right ventricular (RV) strain (GLSRV) and global longitudinal RV strain rate (GLSrRV), and global circumferential LV strain (GCS) and global circumferential LV strain rate (GCSr). For longitudinal strain, a U-shaped region of interest was traced in the four-chamber views. For analysis of GLSLV and GLSrLV, an acquisition in the four-chamber plane focused on optimal LV visualization was used. For analysis GLSRV and GLSrRV, the acquisition focused specifically on the right ventricle ( Figures 1C and 1D ). For circumferential strain, a circle-shaped region of interest was traced in the short-axis view at the level of the mitral valve. For each measurement, the ventricular endocardium was roughly marked at end-diastole, and acoustic markers were automatically tracked throughout the cardiac cycle.

MPI measurements of the left and right ventricles were calculated at each time point. The standard method using Doppler flow pattern returning to baseline, not the valve-click method, was used. Pulsed-wave Doppler patterns of mitral valve inflow, AV outflow, tricuspid valve inflow, and pulmonary valve outflow were sampled. The time interval from AV valve closure to AV valve opening and the ejection time of the ventricle were measured. These values were then used to calculate MPI for the right and left ventricles according to the following formula: (AV valve closure to opening interval − ejection time)/ejection time. To mimic the clinical practice in our laboratory, each observer measured three successive cardiac cycles and averaged the values for each time interval.
Statistical Analysis
Interobserver variability was measured by two means: calculating intraclass correlation coefficients (ICCs) and Bland-Altman analysis. To compare values across gestational age, generalized linear mixed random-effect models were used to obtain least square means at each time point. Multiple-comparison adjustment was performed using the Bonferroni method. P values < .05 were considered to indicate statistical significance. Mixed multilevel modeling incorporating a random-effects model was used to construct mean and percentile values. All dependent variables were log e transformed to normalize their distributions. A linearizing function of gestational age was obtained from the best-fitting fractional polynomial. The model consisted of fixed components (intercept and slope[s]) and random components (random effect for intercept alone). For all parameters, a residual diagnostic test was performed; 2 log likelihoods and the likelihood ratio test were used to assess model fit. Mathematical equations for the gestational age-specific expected values and SDs were generated for each parameter as follows: assuming Y ij equals the dependent variable of interest at gestational age j (GA j ), the expected value, E (), and variance, Var(), of the log-transformed Y i ( Z i ) at GA j are
E ( Z i ) j = μ i = β 0 i + β 1 i GA j
Var ( Z i ) j = σ 2 i = σ 2 int i + σ 2 e i ,
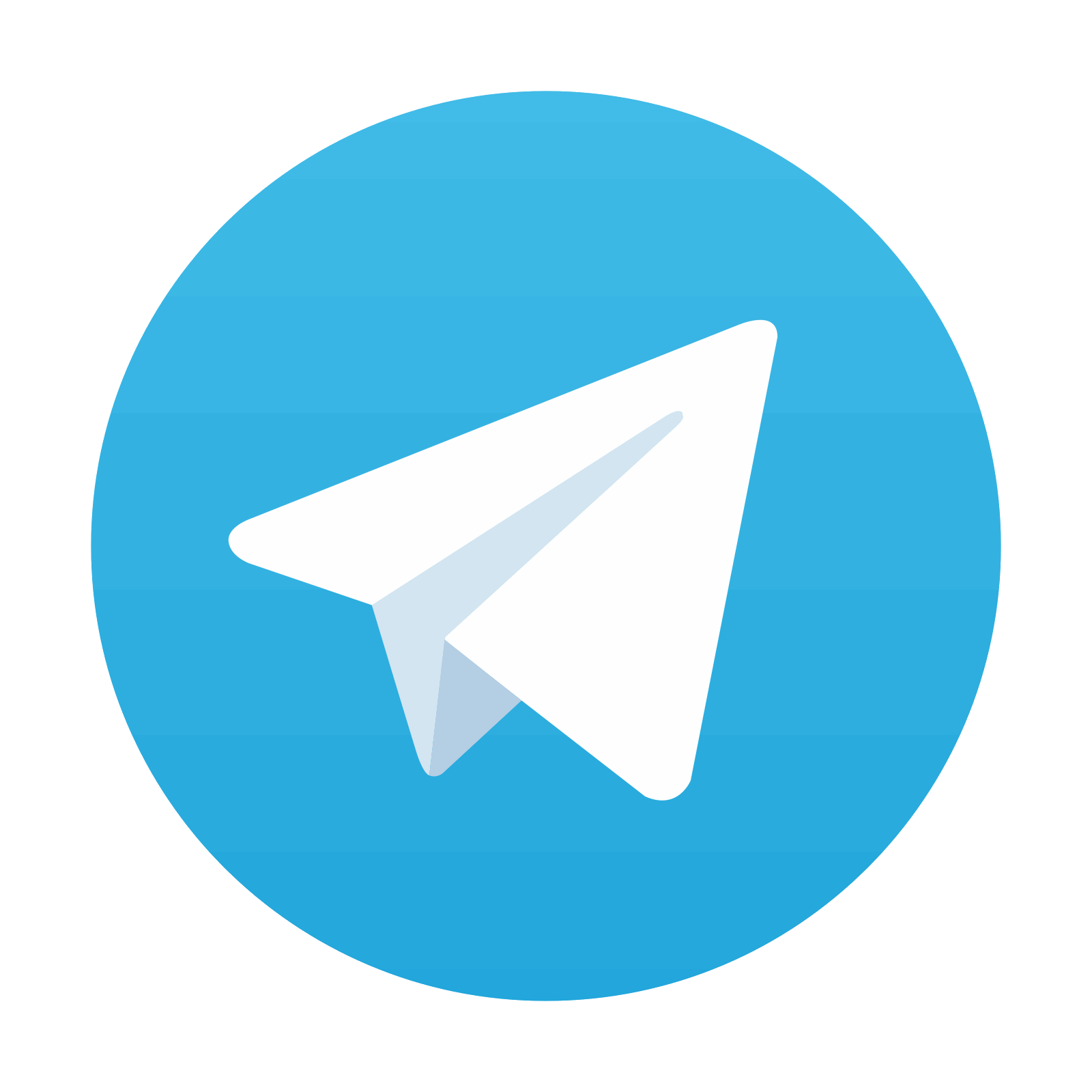
Stay updated, free articles. Join our Telegram channel

Full access? Get Clinical Tree
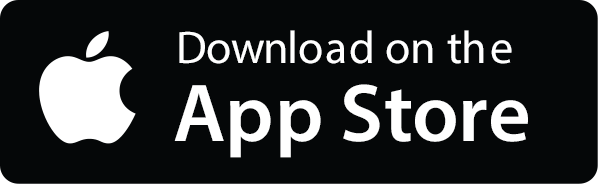
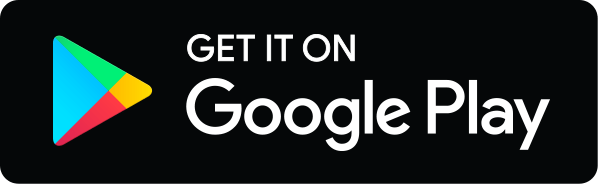