Liver and Portal Venous System
15.2Anatomy and Common Variants
15.3Examination Technique Including Contrast Administration
15.4Normal Findings, Variants, and Hemodynamics
15.6Diagnosis in Surgical and Interventional Percutaneous Procedures
15.7Comparison of Color Duplex Sonography and CEUS with Other Modalities
15 Liver and Portal Venous System
15.1 General Remarks
“We believe that imaging of the liver is complicated.”8
Color Doppler imaging (CDI) has been used in the routine clinical imaging of abdominal diseases for nearly 40 years. Rapid technical advances have taken place during that time. Greatly improved sensitivity in the detection of slow and low-volume blood flow, the advent of power Doppler, B-flow, superb microvascular imaging (SMI), and the use of ultrasound contrast agents have dramatically improved the diagnostic utility of ultrasound. Advances in B-mode image quality from techniques such as tissue harmonic imaging (THI), automatic image correction modes, methods of artifact reduction such as image averaging techniques, and advances in transducer technology have contributed to the ongoing evolution of diagnostic ultrasound. B-flow for the B-mode imaging of flowing blood was introduced in 1999 for use with higher-frequency linear transducers, and in 2013 the technique also became available for lower-frequency curved arrays.
Meanwhile, the range of therapeutic options has been expanding and has created new challenges:
•Ultrasound-guided ablative therapies for focal liver malignancies, including follow-ups
•New developments in tumor surgery, embolization, and ablative procedures such as the radiofrequency ablation (RFA), microwave ablation (MWA), and irreversible electroporation (IRE) of tumors
•Selective internal radiotherapy (SIRT)
•Immune therapy for acute and chronic liver diseases as well as in malignant liver lesions135 , 136
•Interventional radiologic procedures such as the transjugular intrahepatic portosystemic shunt (TIPS)
This chapter will explore the capabilities and limitations of color duplex sonography and contrast-enhanced ultrasound (CEUS) in the detection and characterization of primary and secondary diseases of the liver and its vessels.
15.2 Anatomy and Common Variants
15.2.1 Oxygen and Nutrient Supply of the Liver
The liver receives approximately 70% of its blood supply from the portal vein and approximately 25% to 30% from the hepatic artery or arteries. The arterial and portal venous blood flow have a reciprocal effect on each other through sinusoidal anastomoses and the presence of presinusoidal arterial and portal venous sphincters.
15.2.2 Arterial Blood Supply
The liver derives its arterial blood supply from the proper hepatic artery, a branch of the common hepatic artery that generally arises from the celiac trunk. The vascular supply is subject to considerable variations in its origins and branches. A knowledge of normal variants can have major importance, especially in the planning of liver surgery and in liver transplantation: One-third of all adults exhibit variants in the origin or course of the celiac trunk and superior mesenteric artery. Variants may involve the presence of accessory or aberrant vessels.80 The most common variants are illustrated in Fig. 15.1 and Fig. 15.2.
•Both the aberrant (11%, Michels type III) and accessory right hepatic artery (7%, Michels type IV) arise from the superior mesenteric artery and run posterior to the portal vein in the portocaval space (Fig. 15.2a).
•An aberrant common hepatic artery arises from the superior mesenteric artery in 2.5% to 4.5% of the population (Michels type IX).
•The aberrant left hepatic artery arises from the left gastric artery (10%, Michels type II) and runs to the hepatic left lobe in the ligamentum venosum (Fig. 15.2b).
•The celiacomesenteric trunk is a rare variant in which the two major vessels form a common trunk.
Fig. 15.1 Most common variants in the origin of the hepatic arteries from the aorta. CHA, common hepatic artery; LGA, left gastric artery; LHA, left branch of hepatic artery; RHA, right branch of hepatic artery; SMA, superior mesenteric artery. (Adapted with permission from Abu-Yousef.1)
Fig. 15.2 Common variants in the arterial blood supply of the liver. (a) Right hepatic artery arising from the superior mesenteric artery. (b) Isolated origin of the right hepatic artery from the celiac trunk in B-mode and color duplex. (c) Arrow points at red color dot, an accessory in the ligamentum venosum (epigastric longitudinal scan). It arises from the left gastric artery.
15.2.3 Portal Vein
The portal vein is formed behind the pancreatic isthmus by the confluence of the superior mesenteric vein and splenic vein (see Fig. 12.5). Its extrahepatic portion is 8 to 10 cm long and runs laterally and obliquely to the porta hepatis, passing between the common bile duct and hepatic artery in the hepatoduodenal ligament. Its elliptical diameter in a healthy, fasted patient is 8 to 13 mm. On entering the liver, the portal vein generally divides into two main branches, accompanied by the branches of the hepatic artery and bile ducts.
•The right branch runs for 1 to 3 cm before dividing into an anterior and posterior branch, which supply the right lobe of the liver. Each of these branches in turn divides into a superior and inferior branch.
•The left branch enters the left lobe of the liver, its transverse part turning anteroinferiorly to form the umbilical part of the left portal vein branch. The round ligament of the liver appears as an echogenic band in continuity with the left branch in the falciform ligament. The round ligament is the remnant of the obliterated umbilical vein. In portal hypertension, a paraumbilical vein may form that displays hepatofugal blood flow directed along the hepatic round ligament to the umbilicus (Cruveilhier–von Baumgarten syndrome).
The caudate lobe is supplied by branches arising from the left portal vein branch. Veins drain directly from the caudate lobe into the inferior vena cava. The cystic veins generally drain into the right main branch of the portal vein but rarely may open directly into the main trunk. They unite with the veins of the common bile duct and may form a collateral network in response to occlusion of the portal vein.
15.2.4 Intrahepatic Vascular Distribution and the Hepatic Segments
The intrahepatic portions of the hepatic arteries and portal veins run parallel to the bile ducts in the periportal fields or within the portal triad. Past the division into preterminal and terminal branches, blood drains from the portal veins into the sinusoids along with blood from the accompanying arterial branches. The hepatic lobules with the interspersed arteries, portal veins, and bile ducts form the acini, or functional units of the liver. The arteries and portal veins run between the hepatic trabeculae and connect with the sinusoids. Blood from the sinusoids then drains through the central veins of the lobules into the segmental veins, which usually unite to form three main hepatic veins. At the confluence of the hepatic veins, blood empties into the inferior vena cava just before entering the right atrium of the heart.
For reasons relating to operative strategy, an awareness of normal variants is particularly important for resecting metastatic tumors at the confluence of the hepatic veins. In the most common situation (approximately 46% of cases), the middle and left hepatic veins unite before emptying into the inferior vena cava. In 28% of cases, all three hepatic veins open separately into the inferior vena cava. About 16% of cases show an isolated termination of the right hepatic vein and a left hepatic trunk (formed by the middle hepatic vein, left hepatic vein, and an accessory left hepatic vein). Around 4% of cases show termination of an accessory right hepatic vein along with the left and middle hepatic veins. More than three main hepatic veins are present in 30% of cases.64
Besides the variants of the principal veins, a variable number of accessory veins may be found. They are most commonly encountered in the area where the liver attaches to the diaphragm. Among them are veins named veins of Sappey located close to falciform ligament, with a small-sized lumen. They drain the venous blood from the anterior part of the abdominal wall directly into the liver (see Fig. 15.24). The principal accessory veins are the right inferior phrenic veins, dorsal hepatic veins, veins of the caudate lobe, and collaterals to the right suprarenal vein. The veins of the caudate lobe are responsible for survival of patient with Budd-Chiari syndrome.58
Segmental divisions of the liver have been defined based on the course of the portal vein branches and other venous branches. Each segment is centered on a portal vein branch, while the hepatic veins course at the periphery of the segments and separate them from one another (Fig. 15.3). Despite numerous variants, this functional scheme of hepatic segmentation has proven helpful in the surgical management of liver tumors.30 , 64 , 93
Fig. 15.3 Segmental anatomy of the liver as described by Couinaud (1957).
The left lobe of the liver consists of segments II, III, and IV. The left liver lobe is bounded medially by the falciform ligament and on the right side by the middle hepatic vein. The portal vein branch divides segment IV into subsegments IVa and IVb. When the liver is viewed from the front, the numbering of the segments proceeds in a clockwise direction. The right lobe consists of segments V to VIII. Lateral to the middle hepatic vein, segment V is inferior while segment VIII is superior. Lateral to the right hepatic vein are segments VI (inferior) and VII (superior).
15.3 Examination Technique Including Contrast Administration
15.3.1 Examination Protocol and Doppler Measurements
Patient Preparation
The patient should be fasted for quantitative flow measurements in the hepatic or mesenteric veins because flow velocities and flow volumes may be greatly affected by the time since the last meal. Consequently the patient should be fasted for at least 4 hours to ensure that the blood flow measurements can be followed and compared over time. The patient should be positioned supine for ultrasound scanning of the hepatic and portal veins.
Procedure
After the large hepatic veins have been scrutinized in the B-mode image, the vascular segment of interest is evaluated by CDI and, if necessary, by pulsed-wave (PW) flow analysis. B-mode evaluation includes an assessment of vessel calibers and wall characteristics, while flow direction and velocity range are analyzed with color flow imaging.
▶ Arteries. The common and proper hepatic arteries are identified in relation to the celiac trunk and are traced into the hepatoduodenal ligament (Fig. 15.4). Their intrahepatic branches are seen running parallel to the portal vein branches. The most suitable site for duplex interrogation will depend on the quantitative parameters of interest. For determining flow velocity and blood volume, it is best to choose a relatively straight vascular segment that will allow for optimum angle correction. The angle of insonation should not exceed 60 degrees. The proper hepatic artery often runs a tortuous course in the porta hepatis, and this usually prevents reliable angle correction. Because the resistive index (RI) is largely independent of insonation angle, it is more suitable than velocity data for characterizing blood flow. Spectral waveform analysis should include attention to a rapid upslope (acceleration) and early systolic peak.
Fig. 15.4 Examination of the hepatic arteries starting from the celiac trunk. (a) B-flow image displays the origin and branches of the celiac trunk. (b) Doppler shift signals from the celiac trunk. (c) Doppler shift signals from the common hepatic artery. (d) Doppler shift signals from the splenic artery.
•The divisions of the umbilical part of the left portal vein branch can be demonstrated from the epigastrium (segmental branches to II, III, and IV).
•The divisions of the right portal vein branch (segmental branches V, VI, VII, and VIII) can be defined in a subcostal transverse scan at the level of the midclavicular line (MCL) on the right side.
The caudate lobe is supplied by small veins arising from the left portal vein branch. Veins drain directly from the caudate lobe into the vena cava, and this drainage is displayed particularly well in patients with right heart failure due to the enlarged vein diameters.
Although velocity measurements in the portal vein are quite reproducible when the Doppler angle is sufficiently small, the determination of portal vein volume flow has proven difficult due to methodologic constraints (patients fasted and not on vasoactive medications, selection of measurement site, elliptical to round vascular cross section). This accounts for the large range of normal values reported in the literature.
▶ Hepatic veins. The hepatic veins are best demonstrated in a subcostal oblique scan and in longitudinal scans through the left and right lobes of the liver. The hepatic veins in a healthy liver run in smooth arch from the periphery to the vena cava forming branch angles of less than 45 degrees. They display smooth, sharp borders with no intraluminal echoes. The principal veins can be traced to the second- and third-order levels with CDI. Their flow pattern shows cardiac modulation with brief retrograde flow during atrial systole. In duplex examination of the hepatic veins, the timing of the last meal does not appear to have a significant impact on Doppler measurements.115
15.3.2 Equipment Settings and Flow Detection Techniques
▶ Transducer selection. Selection of the transducer and of the B-mode and CDI frequency depends on the location of the vessel of interest and on the sound conductivity of the liver. Children and thin patients can be scanned with higher-frequency transducers, while muscular and obese patients will require lower frequencies, especially in the Doppler modes. The use of a phased-array transducer may be helpful under difficult insonation conditions, such as postoperative patients with a small acoustic window and small liver.
▶ B-mode. The frequency, gain, time gain compensation (TGC), and focal depth for B-mode ultrasound should be adjusted so that the liver tissue appears homogeneous throughout the image, including the far field. Imaging a very fatty liver requires a lower frequency (also for CDI), and THI may have to be omitted. Some equipment manufacturers offer a preset for obese patients that even includes a modified pulse length. When interpreting the B-mode image, the sonographer should look for a straight vessel course with hepatic veins branching at acute angles. Attention is also given to vessel caliber, wall contours, and any normal variants that may be present.
Often the B-mode image can detect blood flow by the presence of spontaneous contrast in the hepatic veins and inferior vena cava—a finding that is without pathologic significance. It most likely relates to the stacking of red blood cells (rouleaux) to form larger, more echogenic reflectors.39 When the THI mode is used along with higher-frequency broad-band transducers, spontaneous contrast is often seen predominantly in the hepatic veins and vena cava (Fig. 15.5). The slowing of blood flow, especially in the peripheral veins, promotes the formation of “erythrocyte sludge” and is consistently observed in congested veins.
Fig. 15.5 When tissue harmonic imaging (THI) is performed with a high-frequency broadband transducer, spontaneous contrast is often visible in the hepatic veins and vena cava. (a) Spontaneous contrast in the hepatic veins. (b) Spontaneous contrast in the inferior vena cava.
▶ Color duplex sonography. CDI displays a mean or modal blood flow velocity within a selected color box. It can detect local velocity changes based on changing color intensities or color inversion, even without the use of pulsed Doppler. This requires matching the PRF and if necessary the color gain to the velocity in the vessel of interest. The brief atrial reverse-flow component in hepatic venous flow may be missed if the wall filter or PRF is set too high or if the frame rate is too low in color mode.
▶ Power Doppler. The main advantage of power Doppler is its ability to detect very slow volume flows in small-caliber vessels at an unfavorable Doppler angle.124 Only bidirectional power Doppler can indicate flow direction. However, almost all equipment manufacturers can add a Doppler signal to the amplitude signal, creating an imaging mode that can detect flow direction.
▶ SMI. Similar techniques applying an improved, highly sensitive Doppler technique to image blood flow within a color box without displaying flow direction. It also provides a very high spatial resolution.
▶ B-flow. Techniques such as B-flow (see Chapter 3.7.12) and especially CEUS are increasingly used in the evaluation of liver diseases and have become established tools for diagnostic management. It does not require a “color box,” the whole image is taken for flow imaging.
15.3.3 Contrast Examination
Ultrasound contrast agents (see Chapter 4.1) are based on the frequency response of microbubbles undergoing resonant oscillation in flowing blood and of stationary microbubbles in the sinusoids of the liver and spleen. The degree of signal enhancement depends largely on the following factors:
•Acoustic properties of the liver
•CEUS setting and the quality of the ultrasound machine (pulse-inversion technique, high resolution contrast imaging or amplitude modulation at different transmit frequencies, slice thickness and possible blooming will interfere with the spatial resolution)
•Dose of contrast agent depends on the contrast agent, ultrasound device, and contrast setting
•Applied acoustic energy (measured as the mechanical index (MI) value or acoustic output as a percentage of the emitted sound energy)
•Mode of contrast administration (bolus injection or infusion)
When the contrast agent is administered by peripheral intravenous (i.v.) bolus injection (usually through the cubital vein), it first reaches the hepatic artery. It enters the portal vein approximately 3 s later, passes through the terminal vascular branches in the liver, and is cleared through the hepatic veins into the vena cava (Fig. 15.6).
Fig. 15.6 Distribution of contrast medium after intravenous (i.v.) administration. (a) Overview of contrast bolus transit through the capillary beds. (b) Blood flow (contrast distribution in the hepatic lobule).
When the contrast agent is administered through a central venous line or port, the arrival times of the microbubbles are shortened. Because the contrast agent is retained longer in the sinusoids, there is almost complete washout of the agent from the hepatic veins by the late phase, while signal enhancement persists in the liver parenchyma.
Analogous to computed tomography (CT), three consecutive phases of contrast enhancement are distinguished in the liver (Table 15.1)2 , 82:
Table 15.1 Definition of enhancement phases in the liver (Based on Albrecht et al3)
Phase | Visual microbubble detection time after bolus injection (seconds) | |
Start | End | |
Arterial phase | 10–20 | 25–35 |
Portal venous phase | 30–45 | 120 |
Late phase | > 120 | Approximately 240–260 (microbubble washout) |
•Arterial phase (< 30 s), which is dominated by arterial flow in the liver parenchyma
•Portal venous phase (31–120 s), in which portal venous flow is predominant
•Late phase (> 120 s)
In young patients, sensitive imaging techniques can usually detect the first microbubbles only 8 to 10 s after injection. A diagnostic enhancement may persist for more than 5 to 6 minutes. The times stated for contrast inflow apply only to normal pressure dynamics in the right heart and only if there are no unphysiologic arteriovenous (AV) shunt volumes in the lung, bowel, or liver. After passing through the lung, the contrast agent passes from the left heart into the arterial circulation 8 to 12 s after i.v. bolus injection. It passes through multiple capillary regions (lung, bowel, spleen, liver) before reentering the right ventricle. A large, physiologic “shunt volume” is delivered directly to the liver through the hepatic artery. The physiologic arrival and transit times are shown in Fig. 15.7.
Fig. 15.7 Contrast arrival and hepatic transit times. Light gray bar shows difference in arrival time between the three hepatic vessels. HA, hepatic artery; HV, hepatic vein; PV, portal vein. (Adapted with permission from Weskott.125)
For the characterization of known lesions, the site is imaged with the best possible sound quality and is scanned in a plane that includes the feeding portal vein and arteries (see also Fig. 15.43 and Fig. 15.47). The most useful period is the initial seconds of the wash-in phase. At this time the examiner can assess the degree of vascularity, vascular architecture, shunts, direction of contrast spread, and differences in local contrast and bubble (vascular) concentration. Moreover, the relationship of a lesion to adjacent vessels and possible tumor invasion are appreciated most clearly in the wash-in phase. The washout of hepatic metastases begins in the late arterial phase or at least by the early portal venous phase.
Enhancement characteristics also require documentation. The whole arterial phase should be documented with a cine clip, as the degree of tumor vascularity is best appreciated during the initial seconds of arterial contrast arrival. Imaging documentation of the portal venous phase and late phase can usually be limited to a few seconds.
For cases where liver abnormalities are found, several examples will be presented to illustrate the enhancement characteristics of the most common hepatic lesions (see Chapter 15.5.9). With few exceptions, CDI does not contribute to lesion detection and is of minor value for lesion characterization. If tumor vascularity is demonstrable by CDI, the contrast dose for CEUS should be reduced to avoid a blooming artifact in the arterial phase.
15.4 Normal Findings, Variants, and Hemodynamics
▶ Hepatic artery. The hepatic artery can be identified and traced as a large vessel arising from the celiac trunk (Fig. 15.4). Color Doppler shows that it is perfused by continuous, hepatopetal flow. When the PRF is optimized for the portal vein, aliasing may occur. Delineation of the portal vein may be difficult, so B-flow or SMI can be supplemented. Taking advantage of the differences in bubble arrival time on CEUS, the course of hepatic arteries can be imaged by an early sweep over the right or left liver lobe (Fig. 15.8a,b).
Fig. 15.8 A low MI tissue harmonic imaging (HIREC) taken at the early arterial phase. (a) A cross section accumulation sweep through the right liver lobe shows during the first seconds only the arterial tree (b) Same scan technique taken a few seconds later shows the arterial tree plus the accompanying portal veins, with the arteries being brighter than the veins.
Besides the variants shown in Fig. 15.1, the left gastric artery may arise from the left hepatic artery in rare cases, just as an accessory left hepatic artery may arise from the left gastric artery (Fig. 15.2). With a left gastric artery arising from the left hepatic artery, the arterial vessel in the ligamentum venosum will be perfused by hepatofugal flow. Administering cytostatic drugs through a port into the common hepatic artery in such cases may lead to gastric ulceration, severe gastric hemorrhage, and perforations.
▶ Portal vein. The portal vein should be completely color filled on CDI. Flow is directed toward the liver, and respiratory and cardiac modulation are usually detectable only by spectral Doppler. The normal range for velocity measurements in the portal vein is 10 to 25 cm/s; the peak velocity may rise to more than 40 cm/s after a meal. The main portal vein and its major branches can be traced over its full length in approximately 80% of cases when scanned from the anterior side, and in virtually all cases when scanned through a right intercostal approach.
Variants of the portal vein are rare. Besides a preduodenal location, there have been reports of duplication, aplasia, and anomalous terminations. These variants are easily recognized by attempting to trace the portal vein from its tributaries across the confluence to the porta hepatis (Fig. 12.3).
15.4.1 Hemodynamics
The principal Doppler data for the hepatic vessels are summarized in Table 15.2, Table 15.3, Table 15.4, Table 15.5.
Table 15.3 Measured values and indices (DPI, LVI, PCI) reported for the hepatic artery
Parameters | Measured values | Authors |
TAV (cm/s) | 18.4 ± 8.1 | Walsh et al120 |
19.6 ± 11.3 | Leen et al66 | |
20.4 ± 13.5 | Leen et al67 | |
AC (ms) | 125 ± 42 | Paulson et al89 |
RI porta hepatis | 0.69 ± 0.08 | Paulson et al89 |
0.80 ± 0.04 | Leen et al66 | |
0.79 ± 0.04 | Leen et al66 | |
RI intrahepatic | 0.64 ± 0.08 | Leen et al67 |
0.64 ± 0.02 (average of intrahepatic and proper hepatic artery) | Colli et al27 | |
PI | 1.27 ± 0.27 porta hepatis | Colli et al27 |
0.95 ± 0.17 R hepatic artery | Iwao et al55 | |
0.92 ± 0.1 porta hepatis | Schneider et al102 | |
Volume flow (mL/min)* | 195 ± 103.5 | Walsh et al120 |
216 ± 136 | Leen et al66 | |
194 ± 125 | Leen et al66 | |
228 ± 161 | Leen et al67 | |
DPI | 0.17 ± 0.06 | Walsh et al120 |
0.15 ± 0.07 | Leen et al66 | |
0.14 ± 0.06 | Leen et al66 | |
0.13 ± 0.07 | Leen et al67 | |
LVI | 17.2 ± 4.3 | Iwao et al55 |
PCI | 0.106 ± 0.05 | Dodd et al33 |
Abbreviations: AC, acceleration; DPI, Doppler perfusion index; LVI, liver vascular index; PCI, portal congestive index; PI, pulsatility index; RI, resistive index; TAV, time-averaged velocity. Note: * Measured in the common hepatic artery. |
Table 15.4 Measured values and indices reported for the portal vein (at the porta hepatis)
Parameters | Measured values | Authors |
TAV | 15.9 ± 2.8 | Iwao et al55 |
13.7 ± 5.2 | Walsh et al120 | |
16.6 ± 1.7 | Chawla et al24 | |
15.9 ± 6.2 | Leen et al66 | |
17.9 ± 6.6 | Leen et al67 | |
LVI | 17.2 ± 4.3 | Iwao et al55 |
Volume flow | 1040.1 ± 497.4 | Walsh et al120 |
835 ± 187.4 | Chawla et al24 | |
1301 ± 596 | Leen et al66 | |
1200 ± 572 | Leen et al66 | |
1542 ± 586 | Leen et al67 | |
PI | 0.81 | Hosoki et al52 |
Abbreviations: LVI, liver vascular index; PI, pulsatility index; TAV, time-averaged velocity. |
Table 15.5 Velocities of the a-, x-, v-, and y-waves in cm/s measured in the middle or right hepatic vein
Measured values | Authors | |
a-wave | + 10 ± 0.1* | Teichgräber et al115 |
+ 10.2 ± 4.8** | Weskott et al126 | |
+ 10.0 | Abu-Yousef1 | |
x-wave | −29.9 ± 0.2 | Teichgräber et al115 |
−29 | Abu-Yousef1 | |
v-wave | −1.3 | Abu-Yousef1 |
y-wave | −18 ± 0.16 | Teichgräber et al115 |
−23 | Abu-Yousef1 | |
a: x | −0.4 ± 0.1** | Weskott et al126 |
Notes: * Middle hepatic vein at functional residual capacity. ** Middle and right hepatic veins at end-expiration. |
The hepatic arteries display the monophasic, unidirectional pulsatile Doppler waveform that is typical of visceral arteries. As an angle correction is not always achievable, a quick velocity upstoke (time to peak < 0.07 s) and an RI number between 0.55 and 0.70 condition are regarded as normal with the latter changing due to the fasting/nonfasting situation. The RI and pulsatility index (PI) in the hepatic arteries decline toward the periphery. In patients with nonalcoholic fatty liver disease (NAFLD), the RI number declines with the severity of fatty content while it rises with increasing fibrotic changes.137 After orthotopic liver transplantation (LTX) a persistent hepatic arterial diastolic reversal waveform with an RI number of about 1.0 will be seen in splenic steal syndrome,138 and a low RI in case of a proximal stenosis at the anastomosis. A tardus parvus waveform provides a high specificity of a proximal hepatic artery stenosis in LTX. A low peak systolic velocity (below 48 cm/s) of the hepatic artery with the tardus parvus pattern considerably improves the specificity to over 90%, but decreases the sensitivity to 69%.139 The RI of the hepatic artery decreases during the last trimenster in pregnancy.140 , 141 Table 15.3 lists quantitative parameters for the hepatic artery along with the Doppler perfusion index (DPI), liver vascular index (LVI), and portal congestive index (PCI).
Portal Vein
The Doppler spectrum of the portal vein is age-dependent. While it shows cardiac modulation in younger patients (Fig. 15.9), a predominantly flat waveform is seen in older individuals. As in other vessels, vortex flow may also occur in the portal vein, with spectral Doppler showing reverse flow components. Color duplex scanners with high spatial velocity resolution will occasionally show one or two “brighter” eccentric flow jets near the center of the portal vein. A cross-sectional view of the portal vein may demonstrate blue and red components without aliasing. These CDI and PW Doppler findings reflect the spiral flow pattern in the portal vein. They lead to spectral broadening on Doppler spectral analysis and occasionally create the impression of pseudo-reverse flow, depending on the scan angle.
Fig. 15.9 Normal Doppler spectra sampled from the hepatic and portal veins. (a) Lateral flank scan with color Doppler and spectral views of the portal vein. Flow in the vein is normally directed toward the liver, and cardiac modulation is present in young patients. (b) Color Doppler and spectral views of the right hepatic vein. The green reference line was placed at the start of the P-wave in the electrocardiogram (ECG). It is preceded by an h-wave with hepatopetal flow and is followed by the a-wave, from which a very brief c-wave quickly develops. The x- and y-waves show hepatofugal flow. Between them is the v-wave, which may also have a small hepatofugal flow component.
Quantitative parameters for the portal vein are listed in Table 15.4. The volume flow, and thus the peak flow velocity, vary with food intake: The velocity and volume flow increase approximately 30 minutes after a meal due to increased blood flow through the mesenteric veins. According to Fisher et al, however, the postprandial increase in volume flow measurable by Doppler techniques is still within the range of interobserver variability.40
Hepatic Veins
The flow velocities in the hepatic veins depend on right atrial pressure dynamics and reflect the temporal pressure variations during one cardiac cycle (Fig. 15.9). Their spectral waveforms are influenced by respiratory intrathoracic pressure changes, rising intra-abdominal pressure on elevation of the diaphragm, and patient positioning. As the intra-abdominal pressure rises with deep inspiration, venous return decreases. Thus, Doppler ultrasound measurements in the hepatic veins should be performed at end-expiration with no bearing-down effort by the patient. Due to the brief, physiologic apnea that occurs at end-expiration, one advantage of imaging at that point is the ability to examine poorly cooperative patients during several cardiac cycles.
It reflects the venous pressure changes that occur during atrial systole, ventricular systole, and diastole. The peak flow velocity falls off slightly toward the periphery of the liver. Fig. 15.9b illustrates a normal triphasic waveform sampled from the right hepatic vein.
The designations for maximum and minimum velocities are drawn from terminology used in cardiology (h-, a-, c-, x-, v-, and y-waves):
•The a-wave is generated by atrial systole. It represents the first velocity peak directed toward the vena cava after the P-wave.
•A c-wave, generated by closure of the tricuspid valve, is occasionally demonstrated by hepatic vein Doppler. It has the same direction as the a-wave and is synchronous with tricuspid valve closure. The c-wave (occurring after the P-wave in the ECG) is caused by a bulging of the tricuspid valve into the right atrium. The h-wave (occurring before the P-wave) may be seen in subjects with a prolonged diastole. The “y” wave is often followed by a small, brief, positive wave, the “h” wave, prior to the a-wave. The assignment of velocity peaks can be best done with a synchronous ECG trace. The h-wave and c-wave become less frequent with ageing.
•During the atrial filling phase, the tricuspid valve is closed and atrial pressure falls. Blood drains from the hepatic veins into the vena cava until the peak velocity is indicated by the x-wave.
•As atrial pressure rises, hepatofugal flow from the hepatic veins decreases again until the tricuspid valve opens and the v-wave marks the lowest recorded velocity.
•As blood drains from the atrium into the right ventricle, outflow into the vena cava increases again, forming the peak of the y-wave. During the subsequent filling phase, atrial pressure rises again with a resultant drop in the hepatofugal velocity.18
Thus, the Doppler waveform reflects the changing atrial pressures. The a-wave and c-wave are only occasionally separated in the Doppler spectrum, especially in young subjects, with higher a-waves due to a softer liver tissue: The maximum velocities of the x- and y-waves may differ, and usually the peak diastolic velocity is slightly lower. A brief flow reversal with low velocities is noted at end-diastole in approximately one-half of examinations.90 The phasicity of blood flow may decrease slightly during inspiration. The velocity peak produced by atrial contraction may flatten off during inspiration and lose its retrograde flow direction. From expiration to inspiration, the ratio of x:y increases from 1.4 to 1.8.1 Velocities of the a-, x-, v-, and y-waves measured in hepatic veins are listed in Table 15.5.
▶ Pregnancy-related changes. The phasicity of the Doppler waveform changes during pregnancy. As gestation progresses, the triphasic velocity pattern changes to a flattened, monophasic Doppler profile (80% in the third trimester97).
▶ Effects of heart disease. The initial change seen in patients with right heart overload (Fig. 15.10) is an increase in the a-wave to more than 10 to 15 cm/s (normal: 10.2 ± 4.2 cm/s). The ratio of the a-wave and x-wave (normal: −0.4 ± 0.1) increases and may assume a positive value.126 Increasing right heart failure may be associated with systolic reverse flow into the liver, and the a-wave becomes smaller. Depending on the degree of valvular incompetence, the systolic waveform initially flattens and then changes direction to match the atrial flow direction and may blend with it. Thus the cardiac cycle imparts an M-shaped waveform to the hepatic veins. In patients with severe right heart failure, the atrial wave seems to be absent, and a sinusoidal pattern of alternating flow develops in the hepatic veins. If absolute arrhythmia is present, the atrial portion of the waveform may be absent. If the atrial flutter volume is still hemodynamically significant, multiple a-waves may appear (Fig. 15.11). A blending of the a-wave and hepatofugal x-wave leads to a sawtooth waveform in the hepatic veins. In patients with severe right heart failure, the portal vein branches may also exhibit significant pulsatile flow with a hepatopetal and hepatofugal component.95 Alternating flow in older patients (> 60 years) is generally a sign of severe right heart failure, whereas it is a relatively common finding in healthy young patients (< 30 years). Doppler waveform analysis cannot differentiate between underlying pulmonary or cardiac disease (Fig. 15.12). Interpretation is difficult in patients with hepatic cirrhosis and heart failure, as in cases with coexisting alcohol-induced dilated cardiomyopathy. The flat, monophasic hepatic venous flow may regain its phasicity due to the heart failure, and the waveform may be interpreted as normal. Both organic diseases modulate the height of the a-wave and the a/x ratio in opposite ways.
Fig. 15.10 Spectral waveforms from the right hepatic vein and portal vein in patients with ischemic and dilated cardiomyopathy. (a) Increasing change in the velocity peak of the a-wave with preservation of hepatofugal flow; systolic flow reversal at lower left. The velocity ratio (a- to x-wave) is suggestive of right heart failure (upper left −0.85/a-wave: 18 cm/s; center left −1.5/a-wave: 18 cm/s; lower left + 0.53/a-wave: 6.3 cm/s). (b) Waveforms from the portal veins of the same patients show a increasing ratio between the maximum and minimum velocities.
Fig. 15.11 (a) A 28-year-old woman with severe pulmonary hypertension. Spectrum from the right hepatic vein shows a high-amplitude a-wave and an increased a:x ratio (a:x = −0.93). (b) Right atrial pressure curve in this patient shows as well high a-waves and typical mechanical artifacts appearing as double peaks in the a- and x-waves.
Fig. 15.12 A 45-year-old woman with dilated cardiomyopathy (33 cm/s; a:x = −0.67), proving atrial flutter, resulting from the right heart failure with multiple a-waves indicating atrial contraction is still hemodynamically inefficient.
15.5 Pathologic Findings
15.5.1 Aneurysms
Hepatic Artery
Aneurysms of the hepatic artery are rare. They are an incidental imaging finding in asymptomatic patients. They are believed to be associated with a much higher rupture risk (up to 80%) than in other visceral arteries.79 , 85 Besides degenerative wall changes and trauma, aneurysms may result from inflammatory vascular diseases (mycotic aneurysm) or various forms of vasculitis.31 , 100
▶ Findings. A hepatic artery aneurysm appears on B-mode as a hypoechoic or echo-free mass. Color Doppler usually demonstrates turbulent flow. In aneurysms that have been embolized, the coils can be visualized and possible residual perfusion or reperfusion can be excluded. Over time the thrombus material retracts and the aneurysm becomes smaller. By 2 years ultrasound shows only a shrunken aneurysm sac like that described previously for visceral artery aneurysms (see Chapter 11.5.3).
Portal Vein
Although traditionally believed to be rare, aneurysms of the portal venous system have been detected with greater frequency owing to the use of CDI. They can occur at any age and may be located either in the main trunk just above the confluence or in the more distal portal vein branches.
Their etiology is still controversial. They may be congenital or iatrogenic,10 but there is also evidence of a link to portal hypertension. Another potential cause is poststenotic dilatation due, for example, to anastomotic stenosis after liver transplantation. The aneurysm may cause biliary tract obstruction due to extrinsic compression.
15.5.2 Malformations of the Portal Venous System
Variant positions and malformations of the portal vein have been described in rare cases. Most are extrahepatic and result from disturbances of embryonic venous development or malrotation of the bowel. A preduodenal position of the portal vein or congenital obstructing valves may impede blood flow into the liver.
Intrahepatic Portosystemic Shunt
An intrahepatic portosystemic shunt is a direct connection between the portal vein and hepatic veins or inferior vena cava within the liver (Fig. 15.13). Half of the cases reported in the literature occur in a setting of hepatic cirrhosis and portal hypertension. Some may be congenital, however. Four types are distinguished according to Park et al88:
•Shunt between the right portal vein branch and vena cava
•Shunt between the portal vein and hepatic vein in the periphery of one hepatic segment
•Peripheral shunt between the portal vein and hepatic vein caused by an aneurysm
•Numerous connections between the portal vein and hepatic vein in both lobes of the liver
Fig. 15.13 Peripheral intrahepatic shunt between the portal vein and hepatic vein. (a) B-mode image shows circumscribed dilatation of a portal vein branch. (b) Three-dimensional color Doppler gives an angiogram-like view of the feeding portal vein branch (coded in red), the site of the shunt itself, and the outflow vein (coded in blue).
Extrahepatic Portocaval Shunt
Congenital portosystemic venous malformations are rare abnormalities in which the portal blood drains into a systemic vein (first described by John Abernethy in 1793). Only isolated reports have been published on congenital extrahepatic portocaval shunts. They are characterized by an absence of portal venous inflow. Blood from the spleen and mesentery is not carried to the liver but is channeled into the renal vein or directly into the inferior vena cava. In patients with complete diversion of portal venous inflow, the liver is found to contain multiple adenomas and sites of FNH.62 , 65
▶ Findings Fig. 12.17. Ultrasound typically demonstrates a connection between the splenic vein, superior mesenteric vein, and inferior vena cava. Another finding is nonvisualization of intrahepatic portal vein branches with compensatory dilatation of intrahepatic arteries, which can be traced into the periphery (Fig. 15.7). Patients with this anomaly should be routinely checked for development of liver tumors.
Arterioportal Fistula
Arterioportal fistulas most commonly result from trauma or surgery. They lead to pressure elevation in the portal venous system. With a large fistula volume, the portal vein lumen may show aneurysmal dilatation that can obstruct outflow from the mesenteric venous system.
▶ Findings. CDI shows fusiform dilatation of the portal vein with turbulent flow. The color mosaic artifact typically seen at the periphery of AV fistulas is not always present with an arterioportal fistula. If the pressure elevation is substantial, decreased or retrograde flow will be found in the portal vein trunk distal to the fistula and in the superior mesenteric vein.
15.5.3 Hereditary Hemorrhagic Telangiectasia (Osler-Weber-Rendu Disease)
Osler disease is an autosomal dominant disease that produces characteristic visible lesions in the skin and mucous membranes and may additionally involve almost any parenchymal organ. The prevalence is 1 to 2 cases per 100,000 population, and the liver is reportedly involved in 8% to 31% of patients. Typical findings include arterioportal and portovenous shunts, aneurysms, and a corkscrew-like intraparenchymal course of the hepatic arteries.21
▶ Findings. The arterioportal and portovenous shunts may be detectable by CDI, depending on the shunt volume. The hepatic arteries are usually dilated and elongated with a corkscrew appearance (Fig. 15.14). Naganuma et al found that the flow velocities in the frequently dilated hepatic arteries were significantly higher than in healthy vessels (153 ± 65.2 cm/s versus 64.9 ± 11.4 cm/s).83 Given the large variance in disease severity, the hemodynamics are also variable. Flow volumes in the celiac trunk and common hepatic artery may increase, cardiac output may be increased due to a high shunt volume, and portal hypertension may occur. As a consequence from the high shunt volume a right heart failure may develop. Patients occasionally develop sites of regenerative nodular hyperplasia, similar to FNH.
Fig. 15.14 High resolution contrast image (HIREC) accumulation image during the first few seconds after contrast arrival in a patient with Osler disease involving the liver. Dilated central arteries, early contrast enhancement due to arterializations of the liver and shunts that can be detected during the early arterial phase (here: 12 s). The green arrows point to the shunting area. Hepatic veins start to enhance during the arterial phase (red arrows) after 12 s.
Fig. 15.15 Patient with multiple intrahepatic shunts (a) torturous peripheral arteries at 12s, followed by parenchymal hyperenhancement at (b) 17s and (c) 22 s at the liver periphery.
15.5.4 Obstruction of the Hepatic Veins
Postsinusoidal portal hypertension develops when the hepatic veins become obstructed. Two main types of obstruction are distinguished by their location:
•A truncal form with primary involvement of the large hepatic veins (Budd-Chiari syndrome)
•A fascicular type that starts at the level of the central and sublobular veins (veno-occlusive disease, VOD)
Most obstructions are caused by thrombosis or thrombophlebitis, tumor thrombosis, extrinsic compression, and in rare cases by congenital anomalies such as obstructing valves. Budd-Chiari syndrome and VOD run different courses but patients have the same clinical presentation of a congested liver and portal hypertension, depending on the acuteness of the obstruction.
Budd-Chiari Syndrome
This syndrome, named for Budd (1845) and Chiari (1899), involves a partial or complete occlusion of the large hepatic veins and presents clinically with hepatomegaly, ascites, and abdominal pain. The etiology in almost two-thirds of cases is uncertain. Potential causes include coagulopathies, malignant tumor invasion, trauma, and, very rarely, a congenital membranous septum.
▶ Findings. Besides showing an enlarged hepatic left lobe, ultrasound demonstrates a very large caudate lobe. High-level intraluminal echoes in the B-mode image are strongly suggestive of a thrombotic occlusion (Fig. 15.16), which is confirmed by duplex scanning and especially by color Doppler and CEUS (Fig. 15.17). The development of complex intrahepatic collaterals and absent or reverse flow in the affected hepatic veins are virtually pathognomonic for the syndrome.15 It is important to evaluate flow in the retrohepatic segment of the inferior vena cava, which is often difficult to assess by B-mode imaging due to the size of the caudate lobe and left lobe.
Fig. 15.16 A 25-year-old male with metastatic colorectal carcinoma developed a sinusoidal obstruction syndrome (SOS) (obliterative phlebitis of the terminal hepatic venules) under high-dose chemotherapy, including Cisplatin. He quickly developed jaundice, ascites, fatigue, right upper-quadrant pain, tender hepatomegaly and 20-fold increased levels of transaminases. (a) Liver showed an increased echogenicity. (b–d) Contrast-enhanced ultrasound (bolus injection via central venous port) showed a late arrival with a patchy contrast pattern during the late arterial phase. (e) A slow rise in contrast concentration over time demonstrated in a time-intensity curve (TIC).
Fig. 15.17 Occlusion of the middle hepatic vein in Budd-Chiari syndrome. (a) B-mode image. (b) High resolution contrast image (HIREC) examination pulse inversion imaging (PII) demonstrates occlusion of the middle and left hepatic veins and the inferior vena cava. (c) An accessory right hepatic vein drains into the still-patent portion of the inferior vena cava (HIREC). (d) Contrast-enhanced B-flow image. Additional collaterals carry blood to the azygos vein via paravertebral arteries.
Veno-occlusive Disease
Veno-occlusive disease (VOD) is a collective term for postsinusoidal hepatic venous changes occurring at various sites due to various causes. Initial changes occur in the epithelium of the central and sublobular veins and lead to complete fibrous obliteration of the affected venous branches. Potential causes include spontaneous thrombosis secondary to coagulation disorders, fibrin webs, primary membranous obstructions (irradiation), or tumor-related obstruction.
VOD has a high association with polycythemia vera and especially with bone marrow transplantation due to the combined use of radiation and chemotherapy.57 , 78 This therapy-induced endothelial damage, often due to chemotherapy, affects the small terminal hepatic venous branches and is known as sinusoidal obstruction syndrome (SOS). The clinical diagnosis correlates well with the typical manifestations of VOD: weight gain, hepatomegaly and/or right upper-quadrant tenderness, and jaundice or elevated bilirubin during the first 20 days after bone marrow transplantation. The clinical course may be mild or fulminating with hepatic parenchymal necrosis, ascites, and finally death from multisystem organ failure, often caused by aggressive chemotherapeutic regimes.51
▶ Findings. Sonographic findings are hepatosplenomegaly, ascites, thickening of the gallbladder wall, compression of hepatic veins, and heterogeneous increased echogenicity of the liver parenchyma. The RI number of the hepatic artery exceeds 0.75. There may also be an alteration of portal vein flow, including possible flow reversal. Only 50% of patients show ultrasound abnormalities shortly after transplantation despite clinical manifestations of VOD. However, similar changes may also be seen in patients without VOD.
Thus, ultrasound contributes little to the management of patients with VOD/SOS following bone marrow transplantation or chemotherapy.51 Patients who have been selected for transplantation or aggressive chemotherapy should have a preliminary baseline ultrasound for early detection, then scheduled for subsequent follow-ups under standardized conditions.20 , 50 However, these results are controversial. While Brown et al20 reported a steady decline in Doppler ultrasound–measured mean velocities in the portal vein in 1 patient with VOD and detected flow reversal in 3 patients, Herbetko et al50 were unable to confirm these findings in a larger series of 65 patients. On the other hand, 19 or 20 episodes of VOD were associated with an increased RI in the hepatic artery: 0.8 compared with 0.7 in controls. This could result from counterregulation at the central or sinusoidal level, and studies with large case numbers are still needed to make a definitive analysis. CEUS may add value in clinically suspected SOS (Fig. 15.16).
15.5.5 Circumscribed Hepatic Vein Stenosis
Diffuse especially inflammatory or tumor diseases may lead to circumscribed stenoses ranging from less than 1 mm to several centimeters in length.72 The causes of circumscribed hepatic vein stenosis include extrinsic compression and occasional local tumor invasion (see Fig. 15.18 ).121 Besides liver tumors, the differential diagnosis would include vena cava thrombosis and Budd-Chiari syndrome (see Chapter 15.5.4.1).129
CEUS should be performed to determine the number of occluded hepatic veins and collaterals (Fig. 15.17). Regenerative nodules suspicious for FNH are more commonly found in chronic Budd-Chiari syndrome and are particularly well demonstrated by CEUS.22 , 84 , 117 As the degree of venous stenosis increases, the waveform distal to the stenosis increasingly flattens until it finally becomes a monophasic band (Fig. 15.18). If local stenosis after angioplasty is treated by stenting, a biphasic waveform may be restored.86
Fig. 15.18 Local hepatic vein stenosis with a threefold flow acceleration in the stenotic segment. (a) Color Doppler imaging (CDI) of the right hepatic vein. (b) Arrows indicate the sample volume sites.
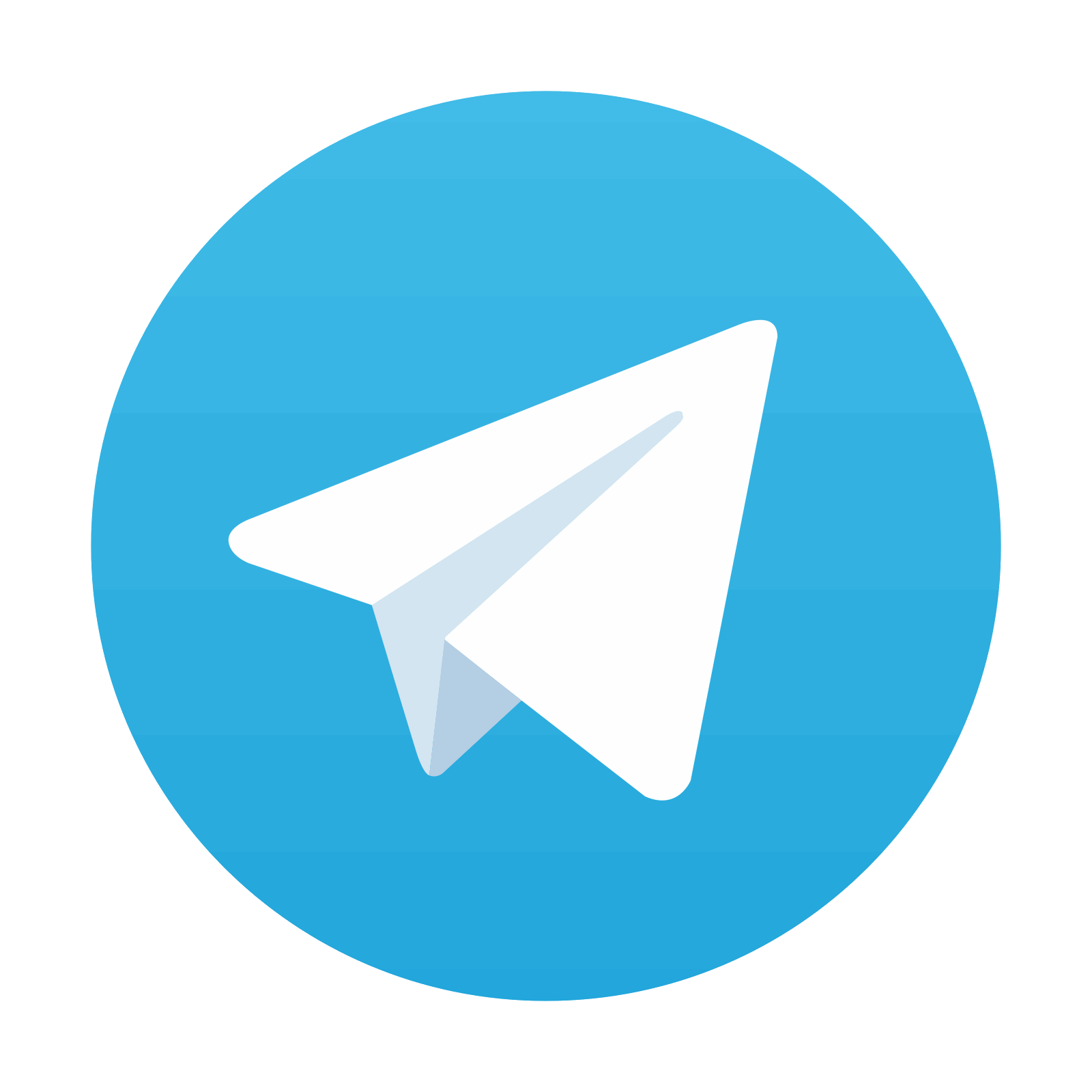
Stay updated, free articles. Join our Telegram channel

Full access? Get Clinical Tree
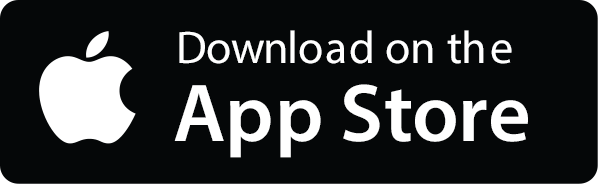
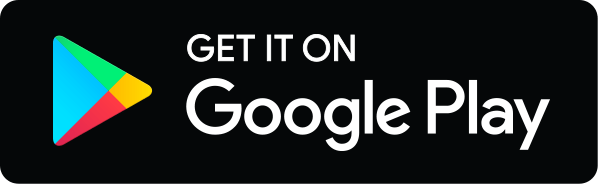