Lipid Disorders
Bibin Varghese
Renato Quispe
Seth S. Martin
INTRODUCTION
Lipid disorders play a critical role in the development of atherosclerotic cardiovascular disease (ASCVD), including coronary heart disease (CHD), stroke, and peripheral vascular disease (PVD).1 A wealth of evidence has confirmed the proportional, causal relationship between low-density lipoprotein cholesterol (LDL-C) and development of atherosclerosis and ASCVD.2,3,4,5 One of the cornerstone therapies in preventive cardiology recommended by the current guidelines worldwide is pharmacologic LDL-C lowering with statin agents6 and non-statin LDL-C lowering agents, in particular ezetimibe7 and proprotein convertase subtilisin/kexin type 9 (PCSK9) inhibitors.8,9 Despite the progress made in primary and secondary prevention with lipid-lowering therapies, further work in this field remains because ASCVD continues to be a leading cause of morbidity and mortality worldwide.3,4
This chapter provides an overview of the epidemiology, risk factors, pathogenesis, clinical presentation, diagnosis, and management of lipid disorders because they pertain to clinical practice.
Epidemiology
More than 12% of the U.S. population (29 million) have total cholesterol (TC) levels greater than 240 mg/dL10 and 7% of U.S. adolescents (ages 6-19) have high cholesterol. In addition, only 55% (43 million) of the patients eligible for lipid-lowering therapy are currently taking it. Although there has been a substantial reduction in average TC levels in the United States over the past decades with lipid-lowering therapies, TC levels still remain the highest in North America and Europe.11,12
Risk Factors
Nonmodifiable risk factors such as age, sex, and ethnicity (ie, South Asian ancestry) are associated with dyslipidemia. Modifiable risk factors linked to dyslipidemia include obesity, blood glucose control, diet, and sedentary lifestyle.5 Finally, secondary causes of lipid disorders including diabetes mellitus, hypothyroidism, medications, chronic kidney disease, nephrotic syndrome, cirrhosis, inflammatory diseases (ie, rheumatoid arthritis, psoriasis, human immunodeficiency virus [HIV] infection), premature menopause, and preeclampsia should be considered in the right clinical context as contributors to dyslipidemia.3,4
PATHOGENESIS
Lipids are hydrophobic molecules that play essential roles in the formation of lipid bilayers, energy storage, bile formation, and production of steroid hormones that are essential for the normal functioning of the human body.2,13 Owing to their hydrophobic nature, lipids need to be transported in serum from sites of origin to target tissues via lipoproteins. Lipoproteins are complex macromolecules with the exterior, hydrophilic portions (free cholesterol, phospholipids, apolipoproteins) enveloping interior, hydrophobic components (such as triglycerides [TGs] and cholesterol esters). Lipoproteins are classified by size, density, lipid composition, and surface apolipoproteins. Examples of lipoproteins include chylomicrons, chylomicron remnants, very low-density lipoprotein (VLDL), intermediate-density lipoprotein (IDL), LDL, lipoprotein(a) (Lp(a)), and high-density lipoprotein (HDL) (Figure 99.1 and Table 99.1).
The exogenous pathway for lipid transport begins in intestinal epithelial cells where fatty acids, the breakdown product of TG, and cholesterol are absorbed.2,13 The fatty acids and cholesterol that are absorbed into intestinal epithelial cells are repackaged into apoB-48-containing TG-rich chylomicrons. These chylomicrons enter the circulation and are broken down by lipoprotein lipase (LPL) found on endothelial cells. The free fatty acids released by breakdown of TG within chylomicrons are either rapidly taken up by nearby tissues (such as muscle or adipose tissue) for energy or transported via lipid binding proteins to the liver for repackaging into VLDL.
The remnant chylomicron particles formed after interaction with LPL undergo reuptake in the liver via apolipoprotein E found on the surface of the remnant particles. As part of the endogenous pathway, the liver produces VLDL, a TG-rich lipoprotein that is smaller than a chylomicron, to transport TG to peripheral tissues to provide energy independent of dietary intake or food availability (Figure 99.2). As opposed to apoB-48 seen on the surface of chylomicrons, each VLDL has one apoB-100 protein on its surface that is recognized by the LDL receptor. Once VLDL particles are secreted by the liver, they also acquire apoC and apoE, at least in part, from HDL. VLDL particles are then broken down in the periphery by LPL. Formed after TG breakdown, VLDL remnants (and the previously mentioned chylomicron remnants) also undergo lipid and protein exchanges with HDL. The remnant particles shed apoC while keeping apoE, and exchange TG for cholesterol
ester with HDL via cholesterol ester transfer protein (CETP). This exchange of apolipoproteins, TG, and cholesterol ester serves to set the metabolic fate of the remnant particles and also helps transport cholesterol from HDL to VLDL remnants so that it can be processed by the liver as part of the reverse cholesterol transport pathway. The VLDL remnant particle, now relatively richer in cholesterol, is called IDL. IDL particles either undergo liver uptake via complex apoE-receptor interactions or undergo further breakdown via hepatic lipase to form LDL.
ester with HDL via cholesterol ester transfer protein (CETP). This exchange of apolipoproteins, TG, and cholesterol ester serves to set the metabolic fate of the remnant particles and also helps transport cholesterol from HDL to VLDL remnants so that it can be processed by the liver as part of the reverse cholesterol transport pathway. The VLDL remnant particle, now relatively richer in cholesterol, is called IDL. IDL particles either undergo liver uptake via complex apoE-receptor interactions or undergo further breakdown via hepatic lipase to form LDL.
![]() FIGURE 99.1 The lipoprotein classes are based on size, density, lipid composition, and surface apolipoproteins. Each of the lipoproteins plays an essential role in lipid metabolism and homeostasis. HDL, high-density lipoproteins; IDL, intermediate-density lipoprotein; Lp(a), lipoprotein(a); LDL, low-density lipoprotein; VLDL, very low-density lipoprotein. |
TABLE 99.1 Characteristics of Lipoproteins | ||||||||||||||||||||||||||||||||||||||||||||||||
---|---|---|---|---|---|---|---|---|---|---|---|---|---|---|---|---|---|---|---|---|---|---|---|---|---|---|---|---|---|---|---|---|---|---|---|---|---|---|---|---|---|---|---|---|---|---|---|---|
|
Because LDL is formed after TG breakdown, these particles are depleted in TG and relatively rich in cholesterol esters. LDL can be considered the “garbage product” of lipid metabolism. Although LDL has been classically considered as responsible for transporting cholesterol ester throughout the body, modern clinical evidence is reframing our understanding of the importance of this function. Specifically, patients treated with statins, ezetimibe, and PCSK9 inhibitors to maintain LDL cholesterol concentrations near-zero do not suffer adverse nonvascular effects.6,14 After transport of the cholesterol ester to peripheral tissues, the LDL particles are uptaken by the LDL receptor on the hepatocyte via the single apoB on the surface of LDL. Once bound to the LDL receptor, clathrin-mediated endocytosis occurs, and the complex is degraded in the lysosome, releasing free cholesterol within the cell. The LDL receptor can then either be recycled back to the surface of the hepatocyte for further reuptake of LDL or it can be bound by the chaperone PCSK9, which pushes the LDL receptor toward a degradation pathway, thereby preventing recycling of the LDL receptor. The balance between the intrinsic rate of synthesis of hepatic LDL and the rate of uptake of LDL by its receptor is what determines the overall LDL particle number and LDL concentration within plasma.
LDL-C is a large component of the guideline recommendations for risk assessment and management of ASCVD.3,4 It has the longest half-life relative to other lipoproteins and typically constitutes greater than 90% of circulating atherogenic lipoproteins.15 In the presence of endothelial injury and
increased oxidative stress, the LDL particles in circulation enter the endothelial lumen, and the single apoB particle on the surface of LDL becomes modified because of oxidative stress that leads to the cascade of events, causing foam cell formation and development of atherosclerosis.16
increased oxidative stress, the LDL particles in circulation enter the endothelial lumen, and the single apoB particle on the surface of LDL becomes modified because of oxidative stress that leads to the cascade of events, causing foam cell formation and development of atherosclerosis.16
![]() FIGURE 99.2 Lipid metabolism begins with exogenous dietary intake of triglycerides (TGs) and cholesterol-rich foods that are metabolized, absorbed, and repackaged into chylomicrons in intestinal epithelial cells. These particles are secreted into the lymphatic system and enter the circulation. The liver also produces endogenous triacylglycerol (TAG-rich) very low-density lipoprotein (VLDL) particles that transport TG and cholesterol to target tissues. Lipoprotein lipase (LPL) found on endothelial cells breaks down TG from chylomicrons and VLDL. Once the TGs are processed, lipid and apolipoprotein exchanges occur with high-density lipoprotein (HDL), which results in the formation of remnant particles. Remnant chylomicron particles are taken up by the liver. After TG breakdown and HDL exchanges, VLDL remnant particles are called intermediate density lipoprotein (IDL). The IDL particles are either taken up by the liver or undergo reaction with hepatic lipase and further lipid exchanges with HDL to form low-density lipoprotein (LDL). (A, Adapted with permission from Rubin E, Farber JL. Pathology. 3rd ed. Philadelphia, PA: Lippincott Williams & Wilkins; 1999. Figure 10.18.; B, Adapted with permission from Harvey RA, Ferrier DR. Lippincott Illustrated Reviews: Biochemistry. 5th ed. Philadelphia, PA: Wolters Kluwer Health/Lippincott Williams & Wilkins; 2010. Figure 18.17.) |
To date, most guidelines emphasize the significance of LDL-C concentration in preventive cardiology.3,4 The reason for its prominence in guidelines is the wealth of clinical trial evidence for the benefit of pharmacotherapies that specifically target LDL-C. However, other broader measures of atherogenic cholesterol and lipoprotein burden may provide complementary information, such as non-HDL-C (estimated as TC minus HDL-C). Although both LDL-C and non-HDL-C are fundamentally cholesterol-based measures, it is postulated that the circulating concentration of atherogenic particles that come into contact with the lumen of the endothelium can be independently associated with risk in the setting of discordance between cholesterol and particle measures.15 Because each of the atherogenic lipoproteins (LDL, VLDL, and IDL) have one apoB particle, levels of apoB are more closely correlated to particle numbers in circulation than to cholesterol-based measures.17 Nevertheless, often the measures are highly correlated. However, in the case of discordance, apoB levels are more closely associated with ASCVD risk compared to LDL-C or non-HDL-C in cross-sectional and prospective observational studies and Bayesian analysis of statin trials.15
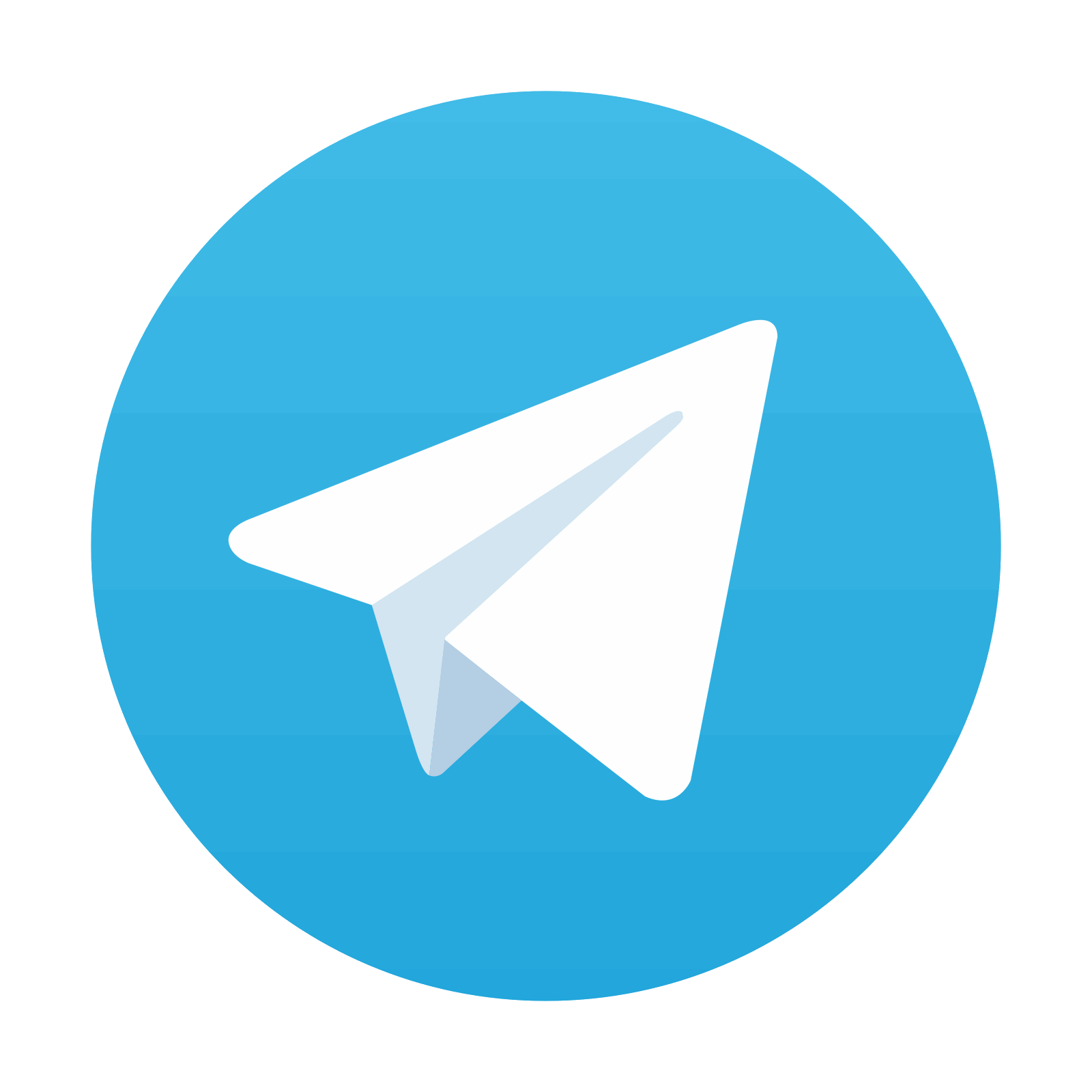
Stay updated, free articles. Join our Telegram channel

Full access? Get Clinical Tree
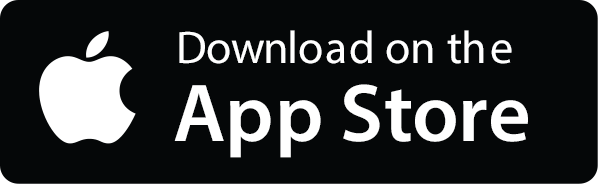
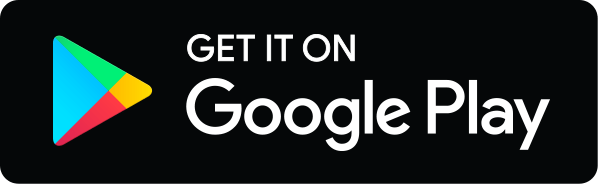