7.1 Upper Extremities
7.1.1 Arteries
General Remarks
Critical ischemia of the upper extremity is much rarer than in the pelvis and lower extremities and accounts for only about 5% of all peripheral arterial diseases.7 It is unclear why this is so. Although atherosclerosis is by far the leading cause of peripheral arterial diseases in the pelvis and lower extremities, stenosis and occlusion in upper extremity arteries are often due to nonatherosclerotic causes. These causes are listed below in descending order of frequency:
•Primary and secondary Raynaud’s syndromes
•Inflammatory vascular diseases (giant-cell arteritis, Takayasu’s arteritis, connective tissue diseases, rheumatic diseases, thromboangiitis obliterans)
•Embolism of upper extremity arteries
•Vascular compression syndromes
•Hypothenar hammer syndrome
•Arterial injuries (iatrogenic, humeral fracture in children)
In the case of acral ischemia, inflammatory vascular diseases cause a critical reduction of blood flow in just 40% of cases, followed by embolism in 20%.
Anatomy and Variants
The arterial anatomy of the arm is relatively simple, as there is only one vascular axis that runs distally, dividing mostly in the forearm into the ulnar artery and radial artery. The vessels show a more complex distribution in the hand and fingers. Thus, the examination technique is clearly prescribed and the arteries are easily visualized with a high-frequency transducer (7–12 MHz) owing to the relatively thin soft-tissue envelope of the arm.
Aortic Arch Branches
The following three large arteries arise from the aortic arch:
•Right brachiocephalic trunk
•Left common carotid artery (CCA)
•Left subclavian artery
The brachiocephalic trunk divides 3 to 5cm past its origin into the right CCA and right subclavian artery. The anatomy of the large supra-aortic branches is shown diagrammatically in Fig. 7.1.
Fig. 7.1 Brachiocephalic trunk, common carotid artery, and subclavian artery with the principal suprascapular side branches.
Anatomic variants are encountered in approximately 15% of the population (Fig. 7.2). The most common variant observed (8%–10%) is the presence of common trunk of brachiocephalic (right side) and left common carotid arteries arising from the aortic arch as a separate vessel (Fig. 7.2g). In 5% of cases, the left vertebral artery arises directly from the aortic arch. Much less common is a truncus bicaroticus with upper limb arteries arising by separate origins or all four vessels arising separately from the aorta.
Fig. 7.2 Diagrammatic representation of the aortic arch with the origins of the large supra-aortic branches and anatomic variants. (a) Aortic arch with the origins of the large branches. (b) Common origin of the brachiocephalic trunk and left subclavian artery. (c) “Bovine arch” with a common origin of the brachiocephalic trunk and left common carotid artery. (d) The left vertebral artery arising directly from the aortic arch. (e) Truncus bicaroticus. (f) Separate origins of all four aortic arch branches. (g) Common origin of the left carotid and subclavian arteries (“bilateral brachiocephalic trunk”). (h) Rare right-sided aortic arch.
Subclavian Artery and Axillary Artery
The left subclavian artery arches over the pleural dome and gives off five branches in its course: The first is the vertebral artery, which runs cranial. The internal thoracic artery (internal mammary artery) arises opposite the vertebral artery and descends on the inner surface of the chest wall. The next branches are the thyrocervical trunk and costocervical trunk, which run cephalad, and the scapular artery, which runs posterolaterally.
The subclavian artery passes through the interscalene triangle, bounded anteriorly by the anterior scalene muscle, posteriorly by the middle scalene muscle, and inferiorly by the first rib. From the inferior margin of the scapula to the distal border of the pectoralis major muscle, the vessel is called axillary artery. As a rare variant, the axillary artery may give rise to the deep brachial artery (profunda brachii) or an unusually high origin of the radial artery. Other axillary branches are the subscapular artery, lateral thoracic artery, and anterior and posterior humeral circumflex arteries (Fig. 7.3).
Fig. 7.3 Arterial anatomy of the shoulder and arm. (Reproduced with permission from: Schuenke M, Schulte E, Schumacher U. THIEME Atlas of Anatomy. General Anatomy and Musculoskeletal System. Illustrations by Voll M and Wesker K. © Thieme 2020.)
Brachial Artery, Radial Artery, and Ulnar Artery
On reaching the upper arm, the axillary artery becomes the brachial artery. Branching from its medial surface is the deep brachial artery, which runs close to the radial nerve. Approximately 10 to 20 mm past the elbow, the brachial artery divides into the radial artery, which runs superficially, and the ulnar artery, which runs beneath the pronator teres muscle.
The ulnar artery gives origin to the common interosseous artery, which divides into the anterior and posterior interosseous arteries. These very small caliber vessels run distally between the radial artery and ulnar arteries, passing anterior and posterior to the interosseous membrane of the forearm. In 0.7% to 9.4% of cases the ulnar artery runs superficially on the forearm flexors and may lead to complications if inadvertently pierced during an injection or venipuncture.4 , 13
In up to 10% of cases the radial artery arises from the proximal third of the brachial artery (high origin). It then runs down the radial, anterior side of the forearm, gives off the princeps pollicis artery at the level of the wrist, and continues distally to supply the deep palmar arch of the hand (Fig. 7.4).
The superficial palmar arch, which gives off the proper palmar digital arteries, is supplied mainly by the ulnar artery (Fig. 7.4). The blood supply to the superficial palmar arch has three main variants: it is supplied by the ulnar artery in 66% of cases, by the radial artery in 30%, and by the medial artery (a branch of the anterior interosseous artery) in 4% of cases. Each finger is supplied by two proper palmar digital arteries.9 Fig. 7.5 shows the most common anatomic variants of the arteries of the hand.5 Awareness about potential anatomic variants is an important prerequisite for treating critical ischemia of the hand.34
Fig. 7.4 Arteries of the hand. (a) Volar view of the hand arteries with the deep and superficial palmar arches. (b) Dorsal view of the hand with the principal collateral arteries. (Reproduced with permission from: Schuenke M, Schulte E, Schumacher U. THIEME Atlas of Anatomy. General Anatomy and Musculoskeletal System. Illustrations by Voll M and Wesker K. © Thieme 2020.)
Examination Technique
Preliminary Tests
Steno-occlusive disease of the upper extremity arteries should be suspected clinically in patients who complain of claudication when carrying objects, blanching of the hand or fingers during exercise, or necrotic changes in the fingers. Patients may also describe exercise-induced pain when doing unaccustomed work with the arm. Further evaluation is angiologic and includes the following tests and findings:
•Low blood pressure (BP) readings on the affected side
•Absent or diminished radial or brachial pulse
•Delayed reactive hyperemia of affected fingers in response to fist clenching
•Audible bruits over the supra- or infraclavicular fossa, less commonly over the axillary artery, possibly induced by exercise (e.g., fist clenching)
Allen’s Test
In this test the radial and ulnar arteries are simultaneously compressed during repetitive fist clenching. Then the pressure over the radial artery is released, and the hand should flush within 6 to 15 seconds. The same phenomenon occurs with the ulnar artery (reverse Allen’s test). If an artery is occluded or the arch is not fully patent, delayed reactive hyperemia will be seen in individual fingers or in the hand.
Under normal anatomic circumstances, linear array transducers operating at a B-mode frequency of 7 to 10 MHz are best for imaging the arteries of the arm. A lower transducer frequency (approximately 5 MHz) is recommended in obese patients. For imaging in the shoulder region, a 3- to 5-MHz sector transducer may be helpful in very muscular patients. A 3.5- to 5-MHz sector transducer is also used for scanning the origins of the supra-aortic branches. The aortic arch, brachiocephalic trunk, and subclavian arteries can be insonated from the jugular fossa when this type of transducer is used.
Modern multifrequency transducers permit the operator to vary the frequencies in each mode and thus use the highest possible transmission frequency for a given imaging depth. The frequencies used for B-mode image generation are always higher than those used for various Doppler techniques.19 High-frequency linear array transducers (5–17 MHz) with a small footprint are recommended for scanning superficial arteries of the hand or fingers.
Positions of Patient and Examiner
Large Arteries
With the patient’s head tilted back, the origins of the large supra-aortic branches (brachiocephalic trunk, left subclavian artery) are scanned from the jugular fossa with a sector transducer. Specially shaped mechanical transducers were formerly used for this purpose. Today it is best to use an electronic transducer with a small footprint. A small neck rest can be helpful in stabilizing the position of the patient’s head.
It is best to scan the subclavian artery with the patient in a supine or sitting position. Both the patient and the operator should be comfortably positioned, especially in a lengthy examination. Otherwise, difficulties in transducer handling could compromise the quality of the images. The operator should stand at the patient’s head when scanning the subclavian and axillary arteries and comparing the left and right sides. For scanning the arteries of the arm, the operator should sit at the side of the examination table with the patient’s arm positioned on the table in slight abduction.
A less experienced operator should work in a sitting position whenever possible in order to achieve a more stable hand and transducer position. An adjustable-height stool and an adjustable examination table will help the operator maintain an optimal position while scanning the patient. It should be possible for the operator to support the transducer hand or forearm on the examination table, or occasionally on the patient, to facilitate smooth and stable transducer handling.
Digital Arteries
Duplex sonography can display both the velocity curves of the digital arteries and the vessel lumen when high-resolution transducers (7–17 MHz) are used. The blood flow pattern in the digital arteries depends strongly on the room and skin temperature as well as level of excitation, especially in adolescent patients. Digital blood flow decreases in response to cold exposure. Thus, the evaluation of digital blood flow is greatly simplified by immersing the hand in a warm water bath (see Fig. 9.2). This not only provides a largely constant and reproducible examination temperature but can also reverse functional luminal narrowing and cold-induced vasospasms (Fig. 7.11). Additionally, this technique avoids compression artifacts due to excessive transducer pressure and saves ultrasound gel (Video 7.1).
Video 7.1 Short embolic occlusion of a digital artery in the right index finger, with compensatory collateral blood flow (fingertip on the right side of the screen).
Examination Protocol
Color Doppler flow imaging has greatly facilitated examination of the arteries in the upper arm, forearm, and especially the fingers. Arterial flow is coded in red, venous flow in blue. Attention should be given to zones of color brightening (increased flow velocity) or aliasing, and vascular cutoffs should be identified. The latter may also be marked by the diversion of flow into collateral vessels.
Flow disturbances are displayed continuously in the segments proximal to, within, and distal to the presumed site of luminal narrowing with angle-corrected pulsed Doppler. For rapid orientation, we recommend continuous color Doppler imaging of the large arteries and veins in longitudinal or transverse sections from the axilla to the wrist with the arm in slight external rotation. Attention is given to possible normal variants such as high vessel origins (e.g., radial or ulnar artery) and caliber variations (hypoplasia). Alternate scanning in longitudinal and transverse sections is essential for accurately determining occlusion length, location, diameter, and for the evaluation of neighboring structures.
The procedure and scope of the examination depend on the clinical question. If a vascular occlusion near the aorta is suspected, the operator should first look for indirect steno-occlusive criteria in dependent vascular segments. This requires examination of the CCA, vertebral artery, and infraclavicular subclavian artery on the right side or the vertebral artery and subclavian artery on the left side. A diagnosis can be made by supplementing abnormal indirect duplex criteria with direct criteria obtained by transjugular scanning.
Differentiation between a proximal subclavian artery stenosis and occlusion is not always easy but is aided by the auscultation of a bruit. Even if the subclavian artery cannot be interrogated directly beneath the clavicle because of the bone, a frequency spectrum acquired from the immediate infraclavicular segment will yield reliable information on the patency of the vessel at rest.10
A hypoechoic stenosis or occlusion of the axillary or brachial artery should be ascribed to inflammation until another equally plausible cause has been found, as described in Chapter 8. The principal functional stenoses that may occur in the setting of a thoracic outlet syndrome are also described under that heading.
When dealing with digital artery occlusions, it is important to exclude arterioarterial embolism by conducting a level-by-level ultrasound survey that covers the aortic arch (to exclude embolizing plaques by transesophageal echocardiography (TEE)), the subclavian artery (to exclude organic and functional stenosis), the axillary artery (e.g., inflammatory stenosis, compression syndromes), the brachial artery (compression syndromes at the elbow) (see Table 3.9 in Chapter 8), and finally the forearm arteries to the level of the hand (hypothenar hammer syndrome).25 , 27
In rare cases, duplex sonography can also be used to investigate a falsely elevated BP or “unexplained” interarm pressure difference. Medial sclerosis of the brachial artery should always be excluded in patients who have frequent BP readings of > 250 mmHg. This can cause a drastic BP difference when the condition is more pronounced in one arm than the other. Other potential causes of a BP difference are a high division of the brachial artery into the radial and ulnar arteries and an undetected rupture of the biceps brachii muscle (early compression of the artery during cuff inflation).
Normal Findings
The arteries of the upper extremity are easily identified sonographically as pulsating, hypoechoic to echo-free vessels. Their walls appear echogenic relative to accompanying veins. In large vessels the intima-media complex can be discerned on the luminal side with a favorable insonation angle. The brachial artery, radial artery, and ulnar artery are generally accompanied by paired veins and run an essentially straight course.
Waveforms
Normal Doppler waveforms sampled from the subclavian artery, axillary artery, and brachial artery by duplex scanning show a triphasic pattern typical of the extremity vessels. Spectral analysis normally indicates high systolic forward flow with a steep upstroke, rapid downstroke, and a reverse flow component in early diastole followed by brief forward flow.
The normal flow pattern seen on color Doppler is marked by a pulsatile color change. If the pulse repetition rate is not too high, the early diastolic reverse flow is coded in blue and the second diastolic peak along with the first is coded in red so that the triphasic flow pattern is visually detectable in color Doppler mode, as described in Chapter 3 on Hemodynamics.
Compared with waveforms in the pelvic and lower extremity arteries, the greater elasticity of the subclavian and brachial arteries may result in a second period of forward flow in mid-diastole, especially in younger patients. This happens because the primary pulse wave is reflected at the periphery, travels back through the arterial system, is reflected from the momentarily closed aortic valve, and is redirected distally producing the second forward peak (“ping pong” phenomenon). The wave amplitude diminishes as it moves distally because the flow velocity decays in a series of multiple in-line tubes.
Individual Variability
Because the autonomically regulated tone of the cutaneous vessels is subject to large individual variations (cold, warm, or moist hands), the variable frequency spectrum in the forearm arteries and especially the digital arteries also reflects these changes. Frequency spectra acquired from the distal forearm and digital arteries, even in healthy subjects, may show a continuous or even relatively high diastolic flow component when vascular resistance is physiologically low.
The effects of peripheral resistance are further amplified by even slight contraction of the finger muscles (increases the resistance). This produces a triphasic or multiphasic spectrum, which may revert to a monophasic waveform when the muscles are relaxed. Usually, the rapid systolic upstroke is initially preserved. This is different from a monophasic poststenotic or postocclusive waveform in which the systolic upstroke is markedly delayed.
Normally the digital arteries run an essentially straight course, display collateral flow to the opposite side when immersed in warm water (Video 7.1), and can be traced to the level of the fine fingertip vessels owing to the high resolution (< 0.5 mm) of modern scanners. It is not surprising, then, that water-bath ultrasound can define glomus tumors as small as 1 to 2 mm during the investigation of acral ischemia (Fig. 7.6).35
Fig. 7.6 Questionable blood flow disturbance in the thumb of a 46-year-old woman. (a) Skin ink marks a tender spot where pressure elicits a sharp pain radiating to the forearm. (b) Hypoechoic mass. (c) Color Doppler scan at low pulse repetition frequency (PRF) demonstrates a very vascular tumor, which was confirmed histologically as a glomus tumor.
It is not unusual to find caliber variations in the digital arteries, with larger calibers occurring on one side. Pronounced vascular tortuosity and individual occlusions may develop in the hand with ageing, especially in patients who do manual work. The systolic flow velocities in the digital arteries are normally > 20 m/s based on angle-corrected measurements.
Pathologic Findings
It is much rarer for atherosclerotic occlusive disease to be manifested in the upper extremities than in the pelvis and lower extremities. The involvement of upper extremity arteries is less common by a factor of approximately 1:20. Nonatherosclerotic arterial diseases are much more likely to be found in the arm than in the pelvis and legs. The complaints may relate, for example, to a thoracic outlet syndrome, inflammatory vascular disease, or embolism giving rise to an acral ischemia syndrome.12 We refer the reader to our detailed review of nonatherosclerotic vascular diseases in Chapter 3.
Diseases of Upper Extremity Arteries
The spectrum of complaints in upper extremity arterial occlusive disease ranges from claudication symptoms in the upper arm and forearm, functional complaints (Raynaud’s syndrome), and rest pain in the hand to finger necrosis in the setting of acral ischemia.
Hemodynamically significant stenosis or occlusion of the proximal upper limb arteries may lead to intermittent claudication with exercise-dependent pain in the upper arm or forearm. In a high-grade flow obstruction in the subclavian artery, the vertebral artery can usually provide such an effective collateral supply to the upper limb arteries that significant arm claudication will occur only with strenuous exertion (heavy carrying or overhead work). A prerequisite for this collateral pathway is that the vertebral artery arises from the proximal subclavian artery and not directly from the aortic arch (in 5% of cases the left vertebral artery arises directly from the arch). In this case the arms would be supplied by branches of the thyrocervical and costocervical trunks or by thyroid arteries on both sides. Typically, atherosclerotic obliteration is much more common in the left subclavian artery than the right. In one carotid artery study in almost 8,000 patients, 6.5% had BP difference of > 20 mmHg between the arms due to subclavian artery stenosis. While 19% of the patients had no steal from the vertebral artery, 23% had a partial steal and 61% a complete steal. Only 7.4% (38 of 514) of the patients with hemodynamically significant subclavian stenosis were symptomatic. In 32 of the 38 cases, symptoms were referable to the posterior cerebral circulation while only 6 of the 38 patients described complaints in their extremity.18
Stenosis and occlusion of the axillary and brachial artery are rare and most commonly result from embolism, vascular inflammation,21 or trauma such as arterial puncture, supracondylar humeral fracture in children, or axillary and brachial artery entrapment syndrome (see Table 8.11. Arterial catheterization may result in a dissection or pseudoaneurysm. Targeted ultrasound-guided procedures (probe compression, injection of fibrin glue) may be beneficial (Fig. 7.7).
Fig. 7.7 (a) Pseudoaneurysm in the elbow after cardiac catheterization. (b) Typical alternating flow in the pseudoaneurysm neck. (c) Cannula tip (white arrow) is advanced to the pseudoaneurysm. (d) A small amount of Tissucol (0.1 mL) is injected to obliterate the pseudoaneurysm.
The distal brachial artery is a site of predilection for traumatic entrapment or dissection due to a supracondylar humeral fracture in children. Literature reports 1.5% to 19% incidence of vascular injury in children.24 Among our own cases, 8 of 141 children with a supracondylar humeral fracture were found to have brachial artery injury. Given the special situation in children, especially at preschool age (reocclusion after vascular surgical intervention, vasospasm and keloid formation on one hand, excellent collateralization of occlusions without late sequelae, spontaneous recanalization on the other), a wait-and-see approach is justified in cases with a recordable peripheral Doppler spectrum and no clinical signs8 (Fig. 7.8, Fig. 7.9).
Fig. 7.8 Supracondylar humeral fracture in a 4-year-old girl who fell from a hopper ball. (a) Radiograph of the arm. (b) After fracture reduction, a high-grade stenosis of the brachial artery remains. (c) By 6 weeks, the stenosis has resolved completely in response to heparin therapy.
Fig. 7.9 Supracondylar humeral fracture in a 7-year-old boy who fell from a swing. (a) Image showing 3-cm-long occlusion of the brachial artery. (b) The artery recanalized after 3 months’ heparin and aspirin therapy, with moderate residual stenoses and palpable peripheral pulses.
Axillary artery compression syndrome is seen in professional basketball players (see “Axillary artery/posterior circumflex humeral artery (PCHA) injury” in Table 8.11 of Chapter 8). Finally, we will consider brachial artery entrapment syndrome at the elbow, a condition that is rarer than thoracic outlet syndrome. The patients diagnosed in our outpatient units had a history of strenuous physical exertion (farmer, lumberjack, warehouse worker, male newspaper carrier with complaints on Sundays due to bulkier editions) and presented with rest ischemia of the hand or individual fingers (Fig. 7.10). The pathogenesis was related to compression of the brachial artery by the bicipital aponeurosis, a long biceps tendon, a fibrous band, or the pronator teres muscle against the condyles.
Fig. 7.10 Rest ischemia of the right index finger in a 45-year-old warehouse worker. (a) Subungual splinter hemorrhages (black arrows). (b) Focal occlusion of the digital artery with a collateral vessel. (c) Provocative test: The patient flexes the arm against the resistance of the examiner’s hand. (d) Increasing compression of the brachial artery by the long biceps tendon as a function of muscular contraction (none to maximal).
As an adjunct to our discussions of nonatherosclerotic arterial diseases, we shall review the role of color duplex sonography in the investigation of acral ischemia. We have found it helpful in our outpatient units to distinguish between diseases of the vessel wall and of the vessel contents when investigating acral ischemia (Table 7.1).
Table 7.1 Pathogenetic aspects of acral ischemia syndrome of the upper extremity
Diseases of the vessel wall | Diseases of the vessel contents |
Organic, fixed | Local manifestation of systemic disease |
Degenerative | Hypercoagulability |
Inflammatory | Hyperviscosity |
Traumatic (exogenous, endogenous) | Embolism |
Toxic | Cardiogenic |
Functional | Arterioarterial |
Primary (Raynaud’s syndrome, acrocyanosis) | |
Secondary (Raynaud’s syndrome) |
While primary Raynaud’s syndrome is mainly a clinical diagnosis, color duplex sonography, with its ability to detect digital artery occlusions in a warm water bath, can provide information essential for differentiating between primary and secondary Raynaud’s syndromes (Fig. 7.11).14
Fig. 7.11 Long history of Raynaud’s syndrome in a 52-year-old lumberjack. (a) Acrocyanosis in both hands at room temperature. (b) Complete vasospastic occlusion of the digital arteries. (c) Gradual release of the spasm in a warm water bath.
In the presence of digital artery occlusions, sonography can greatly advance the diagnosis of arterioarterial embolism by detection of embologenic plaques, stenoses (inflammatory or degenerative), dissections, or traumatically acquired aneurysms. These conditions require differentiation from autochthonous digital artery occlusions due to severe atherosclerosis (diabetes, renal insufficiency), vasculitis, or trauma.
An exogenous traumatic cause is illustrated by hypothenar hammer syndrome,31 which can lead to painful ischemia of the fingers not only through occupational exposure (truck mechanics) but also in various sports such as baseball, volleyball, mountain biking, or hockey, and sometimes in patients who have to walk on crutches. Responsible for 2% of all hand ischemia, it is caused by acute or chronic recurring trauma to the ulnar artery due to impingement or compression of the vessel against the carpal bones of the hypothenar. It may lead to acute occlusion of the ulnar artery or, in chronic cases, to severe tortuosity or aneurysm formation with embolism to the digital arteries. Fig. 7.12, Fig. 7.13, Fig. 7.14, Fig. 7.15 each illustrates a hypothenar hammer syndrome causing direct vessel wall damage and precipitating arterioarterial embolism in four patients with embolic occlusions of the digital arteries.17 , 36
Fig. 7.12 Hypothenar hammer syndrome in a mechanic with an ulnar artery aneurysm. (a) Traumatic aneurysm of the ulnar artery on digital subtraction angiography (DSA). (b) Perfused aneurysm (arrow) in a longitudinal duplex scan.
Fig. 7.13 Hypothenar hammer syndrome in a furniture mover with a dissection and thrombotic occlusion of the ulnar artery. (a) Occlusion of the ulnar artery (arrow) on digital subtraction angiography (DSA). (b) Transverse scan of the occluded and enlarged ulnar artery (arrow).
Fig. 7.14 Hypothenar hammer syndrome in a farmer with partially thrombosed ulnar artery. (a) Ectasia of the ulnar artery on digital subtraction angiography (DSA). (b) Transverse scan of the partially thrombosed ulnar artery.
Fig. 7.15 Hypothenar hammer syndrome in a professional volleyball player with spastic narrowing of arteries in both hands and occlusion of the ulnar artery and individual digital arteries. (a) Digital subtraction angiography (DSA) demonstrates occlusion of the ulnar artery and digital arteries (arrows). (b) The same arteries in fingers 2 and 3 displaying the occlusion (arrow) in longitudinal section. (c) Same as b.
A similar condition is hand vibration syndrome caused by the prolonged use of vibrating handheld machinery. Depending on the frequency (100–200 Hz), amplitude, duration of exposure, and ambient conditions (cold and moisture), the vibrations may lead to occlusions in the arteries of the palmar arch and digital arteries and may also trigger vasospastic attacks by increasing the number and excitability of alpha α2 and mechanical receptors.1
Stenoses and Occlusions
Incidence
Atherosclerotic wall changes in the upper extremity arteries are rare.22 Proximal stenosis of the left subclavian artery is the most common. According to Vollmar,29 16% of supra-aortic occlusions occur at that location. In the study on subclavian steal syndrome,18 the left side was affected in more than two-thirds of cases.
Examination
Luminal narrowing in the subclavian artery can be detected with simple clinical tests and quantified by arterial BP measurements in the arm. If the readings are borderline with a BP differential of approximately 15 mmHg, an exercise stress test (20 fist clenches) can help to detect a hemodynamically significant obstruction.
When a suitable transducer has been selected and the patient positioned (p. 160), the intrastenotic or immediate poststenotic peak systolic velocity should be determined and related to the waveform and maximum frequency shift in the downstream vascular segment with laminar flow. Use of the color Doppler mode is essential for this purpose.
Stenosis Criteria
The velocity waveforms are analyzed using the standard criteria for extremity arteries. Subclavian stenosis of ≥ 70% can be diagnosed from a vmax syst of ≥ 350 cm/s with a sensitivity and specificity of 87% and 83%, respectively, a vmax diast of ≥ 60 cm/s with 76% and 93%, respectively, and an intra- to poststenotic vmax ratio of ≥ 2 or ≥ 4 with 91%/55% or 76%/91%, respectively, compared with digital subtraction angiography (DSA).9
In case of a less experienced operator or in an emergency (multiple injuries), indirect criteria in the poststenotic or postocclusive region will usually be applied since the site of the stenosis or occlusion itself cannot always be directly visualized. Even an experienced operator may encounter difficulties, especially when dealing with a proximal occlusion. In this case the absence of audible flow sounds combined with a monophasic waveform would create a very high index of suspicion for an occlusion, which could then be confirmed in a symptomatic patient by magnetic resonance angiography (MRA).
High-grade luminal narrowing of the brachiocephalic trunk always leads to a drastic flow reduction in the ipsilateral CCA relative to the opposite side. An exception is a normal variant of the CCA arising separately from the aorta. Fig. 7.16 shows a compilation of direct and indirect stenosis criteria found in a patient with bilateral proximal stenoses of the left subclavian artery and right brachiocephalic trunk.26
Fig. 7.16 Direct and indirect stenosis criteria in a patient with bilateral proximal stenoses in the arteries of the arm. A 73-year-old woman had a long history of vertigo, which she attributed to low blood pressure. Weak palpable radial pulses and loud bruits over both subclavian arteries were noted on physical examination. Both axillary arteries show monophasic waveforms with evidence of high-grade stenosis of the brachiocephalic trunk and left subclavian artery with a vmax of approximately 400 cm/s. (a) Left axillary artery. (b) Right axillary artery. (c) Brachiocephalic trunk. (d) Left subclavian artery. (e) Minimal pulsatility in the right internal carotid artery (ICA). (f) Left ICA for comparison. (g) Partial steal in the right vertebral artery. (h) Complete steal in the left vertebral artery. (i) Resultant alternating flow in the basilar artery. (j) Survey angiogram.
Embolic Occlusion
Acute cardiogenic embolism of the extremities affects the upper limb in 7% to 36% of cases. Thanks to good collateralization, upper limb amputation is necessary in just 0% to 4% of cases compared with 9% to 24% in the lower limb. The right side is affected three times more frequently than the left. The sites of predilection for an acute occlusion are the brachial artery bifurcation at the elbow (25%) followed by the radial artery (11%), axillary artery (6%), and ulnar artery (6%) (Fig. 7.17).6 Clinical criteria for distinguishing between embolic occlusion and acute thrombosis are shown in Table 7.14.
Fig. 7.17 Acute ischemia at rest in the right hand of a 70-year-old man. (a) Pale right hand compared with the left. (b) Nonocclusive embolus at the origin of the radial artery. (c) Embolic occlusion of the ulnar artery. (d) Stump waveform at the elbow (alternating flow with a reduced amplitude).
Dissections
Stanford type A dissections involve the subclavian artery in 5% to 17% of cases and, unlike acute dissections of the internal carotid artery, have a mobile intimal flap over a long vascular segment. The reentry point is usually marked by circumscribed flow acceleration at the end of the dissection.3 Acral ischemia may then result from a combination of luminal narrowing by the dissected flap, local thrombosis, or peripheral embolism (Fig. 7.18).
Fig. 7.18 Necrosis of the left hand in a 63-year-old man following an acute Stanford type A dissection and endovascular treatment. (a) Left hand compared with the right. (b) Mobile dissection flap, identified by the intima-media complex within the vessel lumen. (c) Infraclavicular reentry (white arrow) and retrograde flow in the false lumen. This explains the alternating flow in the frequency spectrum (yellow arrows).
Palmar Arches
The assessment of blood flow in the superficial and deep palmar arches may be important in patients with vascular injuries, after puncture-related vasospasm, dissections or occlusions, or before the creation of a hemodialysis fistula and should be done routinely prior to radial artery catheterization. The functional clinical Allen’s test (p. 158) has a 13% failure rate and cannot be used in the setting of posttraumatic shock.2 An abnormal Allen’s test should always be supplemented by duplex sonographic imaging of the brachial artery and both forearm arteries.
The most reliable criterion for a functionally competent palmar arch is flow reversal in the distal radial or ulnar artery in response to occlusive compression of the vessel proximal to the transducer. Another option is to check the flow direction in the princeps pollicis artery while the radial artery is compressed at the usual site for taking the pulse. Increased flow velocity in the ulnar or radial artery in response to occlusive compression of the opposite artery is too nonspecific.
Acral Circulation
In patients with decreased blood flow in the acral arteries, functional Raynaud’s syndromes should be distinguished from acral vascular occlusions, which can be diagnosed on a preliminary basis by taking pulses or pressure readings of the digital arteries. When duplex scanning is performed with a high-resolution linear array transducer, the following criteria can be used to distinguish chronic from acute occlusions and vasospastic from organic occlusions of the palmar digital arteries:
•Local segmental increase in flow velocity is > 50% compared with the proximal vascular segment.
•Aliasing on color Doppler with a properly adjusted pulse repetition frequency (PRF) and color gain indicates stenosis.
•In contrast to a chronic occlusion, the outlines of an acutely occluded vessel can be clearly visualized.
•Segmental vascular cutoffs in color Doppler mode and the detection of collateral vessels are suggestive of occlusion (note the flow direction).
•Increased tortuosity of vessels (in Winiwarter-Buerger’s disease, but also in patients who work with their hands).
•Peak systolic velocity reduced to less than 15 cm/s with a flat, monophasic spectrum suggests an occlusion proximal to the sample site.
•After immersing the fingers in ice water (5 °C), patients with primary Raynaud’s syndrome show a greatly delayed time to onset of perfusion compared with healthy subjects (3.6 minutes vs. 0.9 minute).28
Findings Associated with Digital Artery Occlusion
In one study of 450 digital arteries (45 hands), the sensitivity and specificity of color duplex sonography in diagnosing digital artery occlusions were 86.3% and 93.1%, respectively, compared with selective hand angiography.16 In a further3 study, the duplex findings for differentiating between primary and secondary Raynaud’s syndromes were subdivided into three groups:
•Group 1: Stenosis or isolated chronic occlusions
•Group 2: Stenoses and occlusions of all digital arteries
•Group 3: Acute occlusions (p. 164)
While 3 of 53 patients in the first group had a primary Raynaud’s syndrome, groups 2 and 3 consisted entirely of patients with a secondary Raynaud’s syndrome resulting from connective tissue disease, Buerger’s disease, antiphospholipid syndrome, or vibrational trauma.
The diagnostic algorithm in Fig. 7.19 was developed at our center and is recommended for the investigation of acral ischemia of the digital arteries.
Documentation
General Recommendations
Every finding should be documented by photo or video documentation, a precise description of the finding, and a final interpretation. The result report should include recommendations for further diagnostic procedures and may include therapeutic recommendations.
Note
The findings should be documented in such a way that the archived image could be shown in a presentation.
This may consist of a video recording of illustrative sequences. Dynamic documentation may include an acoustic recording of the time-varying frequency spectrum in addition to information from color Doppler, B-flow, power Doppler, and B-mode images.12 It is superior to single images for documenting complex findings such as aneurysms and steno-occlusive lesions and ideally requires the latest generation of ultrasound scanners with high storage capacity.
Normal Findings
Depending on the clinical question, relevant regions of interest such as the proximal subclavian artery, axillary artery, or forearm arteries should be documented in representative longitudinal scans that include a frequency spectrum. A color Doppler display can never replace pulsed Doppler flow-velocity measurements or Doppler spectral waveform analysis. Angle correction should be routinely applied for flow-velocity measurements.
Stenoses
Maximum flow acceleration should be documented at the pre-, intra-, and poststenotic levels. If caliber changes are present (hypoplasia, aneurysm, anastomoses), stenoses should be documented in two planes (longitudinal and transverse) with measurements of vascular diameters.
Occlusions
Occlusions should be documented by direct duplex ultrasound criteria (absence of color and Doppler signal in multiple planes with a low PRF, optimal insonation angle, and color gain) and indirect criteria (collateral vessels with distal reconstitution, low resistance index (RI) with a monophasic waveform).
Documentation Guidelines
Quality control standards for diagnostic ultrasound as adopted by the National Association of Statutory Health Insurance Physicians (NASHIP) and the American Institute of Ultrasound in Medicine (AIUM) in 201432 require that physicians document both the indication for the procedure and its conduct. Image documentation in color duplex sonography should include distance scale, transmission frequency, baseline, wall filters, and patient identity. Normal findings are documented by a sectional image in one plane with a Doppler spectrum, and pathologic findings by sectional images in two planes, preferably including a longitudinal scan with a Doppler spectrum. One of the vessels examined, such as the brachial artery, should have image documentation.
The AIUM Practice Parameter for the Performance of Peripheral Arterial Ultrasound Examinations (2019) using color and spectral Doppler imaging shows in detail the indications for peripheral arterial examinations, the qualifications and responsibilities of the physician, the written request for the examination, the specifications of the examination, the documentation required, the equipment specifications, quality control and improvement, safety, infection control, and patient education.
Note
The documentation of a vascular ultrasound examination includes image and waveform documentation of the finding in question, a description of the finding, and the interpretation of the finding with reference to the clinical question that prompted the examination.
Image and waveform documentations are an essential part of quality assurance in diagnostic ultrasound. They should convey information on the rationale for selecting the imaging techniques, proper conduct of the examination, proper image acquisition, and comparability of the findings. Specific recommendations for documenting the upper extremity arteries are as follows:
Standard Protocol
The following vessels are imaged successively in longitudinal section with simultaneous recording of angle-corrected velocity profiles or Doppler frequency profiles, and in transverse section if required:
•Supra- or infraclavicular subclavian artery
•Axillary artery
•Brachial artery
Depending on the indication and clinical question, bilateral examination of:
•Forearm arteries
•Digital arteries in a water bath
Basic Documentation (of Normal Findings)
Bilateral individual documentation of the longitudinal scans with angle-corrected Doppler spectra (peak systolic velocity) in the following:
•Supra- or infraclavicular subclavian artery
•Axillary artery
•Brachial artery
•Forearm arteries (if examined)
•Digital arteries (if examined)
Documentation of Pathologic Findings
Individual color duplex documentation of the longitudinal scan (and transverse scan, if applicable) at the site of maximum narrowing with (in longitudinal scan) associated Doppler spectrum documenting the angle-corrected velocity profile. Individual color duplex documentation of the longitudinal scan of the post- and prestenotic vessel, or at post- and prestenotic sites, with documentation of the angle-corrected velocity profile.
An occlusion is documented with longitudinal and optional transverse color Doppler scans of the occluded artery segment (may include occlusion endpoints in the longitudinal scan) with the associated color and/or Doppler spectrum (in the longitudinal scan) documenting acquisition of the angle-corrected velocity profile from the occluded segment, supplemented if necessary by the preocclusive and postocclusive segments, using low- or slow-flow settings for color Doppler and the velocity spectrum. Digital artery occlusion requires documentation of the preocclusive ulnar and radial arteries.
A hemodynamically significant obstructive lesion can almost always be recognized from the waveform of the distal Doppler spectrum and should not be missed due to a “rapid emergency examination.”
The description of findings provides a verbal description, aided by drawings if necessary, documenting the location, number, and sonomorphology of stenoses or occlusions, giving particular attention to echogenicity, media sclerosis, luminal width, and possible dilatations.
Sources of Error
Duplex sonography is subject to patient-specific and technical errors that may hinder the examination and distort the findings (Table 7.2). An awareness of these pitfalls will help the operator avoid technical problems and make key adjustments that can correct for patient-specific problems. Because these issues may arise in any duplex ultrasound examination of the upper and lower extremities and are comparable in both regions, they will be explored more fully in Chapter 7.2.1 (Lower Extremity: Arteries).
Table 7.2 Potential sources of error in duplex sonography of the upper extremity arteries
Patient-specific problems | Technical problems |
Obesity Motion artifacts Cardiac arrhythmias Inadequate rest period Positional anomalies and variants Venous overlay Prior trauma treatment (dressings, external fixator, plaster cast, surgical site) Restless patient (small children, tremor, etc.) | Improper transducer selection Faulty insonation angle Incorrect settings for color Doppler imaging (e.g., gain, high-pass filter for Doppler frequencies and color Doppler frequencies) Mirror-image artifacts |
▶ Mirror-image artifacts. During imaging of the subclavian artery, the pleura may produce a mirror-image artifact that creates a second, phantom image of the vessel projected onto the lung. This phenomenon can be positively identified as an artifact whenever a “duplicated” subclavian artery is found.
Utility of Color Duplex Sonography Compared with Other Methods
Today, color duplex sonography is considered the standard modality for diagnosing arterial steno-occlusive disease in the upper and lower extremities once preliminary clinical tests (pulse status, auscultation, Doppler pressure measurement, oscillography, finger pressure measurement or finger plethysmography) have helped to formulate a clear diagnostic question. The diagnostic validity of color duplex sonography has already been elucidated for specific vascular regions and indications.
▶ Utility. The strength of duplex sonography in the upper extremity, as in other vascular regions, is that it provides a tool for the noninvasive assessment of blood flow. When color duplex sonography was compared with digital angiography in 297 arterial segments of the upper extremity, it was found to have a mean sensitivity of 90% in the detection of vascular pathology and a specificity of 99%. When applied to the proximal supra-aortic vessels (21 vascular segments), it showed a sensitivity of 91% and specificity of 100%. These respective figures were 93.3% and 100% in the upper arm segments (n = 58), and 88.6% and 98.7% in the forearm segments (n = 201).30 Experienced examiners scanning the digital arteries can achieve a sensitivity and specificity of 86.9% (95% CI: 81%–91%) and 93.8% (95% CI: 91%–96%), respectively.15
In patients with peripheral arterial embolism, surgical outcome for patients with preoperative duplex sonography alone is equivalent to patients evaluated with contrast angiography or computed tomography angiography (CTA), particularly for upper extremity embolism.33
▶ Continuous-wave (CW) Doppler. Ideally, functional stenosis at the infraclavicular level can be detected with suitable provocative tests using a 4-MHz CW Doppler probe. This method is fast and simple compared with duplex sonography and yields reliable results on the hemodynamic significance of functional narrowing. If an arterial thoracic outlet syndrome (TOS) is suspected, the examination to exclude morphologic changes in the subclavian artery (plaque, stenosis, aneurysm, peripheral embolism) should be supplemented by color duplex sonography.
▶ Magnetic Resonance angiography. In symptomatic patients with equivocal color duplex findings, proximal steno-occlusive lesions of the subclavian artery and brachiocephalic trunk in particular should be documented by MRA. This study is unlikely to have advantages over color duplex sonography when applied to infraclavicular vascular segments down to the level of the digital arteries, which are freely accessible to ultrasound. In particular, the detection of functional stenoses in the setting of a thoracic outlet syndrome does not require confirmation by MRA because the degree and location of the varying stenoses can be accurately assessed sonographically. Both modalities require an experienced examiner. This is particularly true when the digital arteries are imaged for purposes of disability evaluation, which is significantly improved by MRA with subsystolic lower arm cuff compression to reduce venous overlay.20
Conclusion
In summary, color duplex sonography permits an accurate evaluation of arterial hemodynamics that can be repeated at any time. When combined with a clinical assessment of the effects of arterial steno-occlusive disease in the upper extremity, it provides a sound basis for definitive treatment planning. Conventional angiography of the large upper limb arteries is necessary only in exceptional cases. Because MRA and CTA provide excellent alternatives to DSA, and do not compete with color duplex sonography, they should be used within a diagnostic algorithm on an interdisciplinary basis.
References
[1]Abudakka M, Pillai A, Al-Khaffaf H. Hypothenar Hammer syndrome: rare or underdiagnosed? Eur J Vasc Endovasc Surg. 2006; 32(3):257–260
[2]Abu-Omar Y, Mussa S, Anastasiadis K, Steel S, Hands L, Taggart DP. Duplex ultrasonography predicts safety of radial artery harvest in the presence of an abnormal Allen test. Ann Thorac Surg. 2004; 77(1): 116–119
[3]Arning C, Hanke-Arning K, Eckert B. Clinical features of dissection in the brain-supplying cervical arteries. Dtsch Arztebl Int 2022; 119 (in press)
[4]Botte MJ. Vascular systems. In: Doyle J, Botte MJ, eds. Surgical Anatomy of the Hand and Upper Extremity. Lippincott Williams & Wilins; 2003:237–293
[5]Coleman SS, Anson BJ. Arterial patterns in the hand based upon a study of 650 specimens. Surg Gynecol Obstet. 1961; 113:409–424
[6]Davies MG, O’Malley K, Feeley M, Colgan MP, Moore DJ, Shanik G. Upper limb embolus: a timely diagnosis. Ann Vasc Surg. 1991; 5(1):85–87
[7]Edwards JM, Porter JM. Evaluation of upper extremity ischemia. In: Bernstein EF, ed. Vascular Diagnosis. St. Louis: Mosby; 1993:630–640
[8]Griffin KJ, Walsh SR, Markar S, Tang TY, Boyle JR, Hayes PD. The pink pulseless hand: a review of the literature regarding management of vascular complications of supracondylar humeral fractures in children. Eur J Vasc Endovasc Surg. 2008; 36(6):697–702
[9]Hafferl A. Lehrbuch der topografischen Anatomie. Berlin: Springer; 1969:784
[10]Hua Y, Jia L, Li L, Ling C, Miao Z, Jiao L. Evaluation of severe subclavian artery stenosis by color Doppler flow imaging. Ultrasound Med Biol. 2011; 37(3):358–363
[11]Karasch Th. Strömungsdarstellung im B-Bild – Die B-Flow- Technologie. Med Klin (Munich). 2001; 96:92
[12]Karasch Th, Ludwig M.. Doppler- und duplexsonografische Untersuchungsverfahren der extrakraniellen arteriellen Vertebralisund Subclavia- und Armstrombahn. Herz/Kreisl. 1999; 31:283–290
[13]Khairnar SV, Nath, RK. Et al Prevalence of abnormal upper limb arterial anatomy and ist correlation with access failure during transradial coronary angiography. Indian Heart Journal 73 (2021) 44–48
[14]Klein-Weigel P, Sander O, Reinhold S, Nielitz J, Steindl J, Richter JG: Raynaud‘s phenomenon—a vascular acrosyndrome that requires long-term care. Dtsch Arztebl Int 2021; 118: 273–80
[15]Ladleif M, LangholzHeidrich H, Blank B, et al. Wertigkeit der farbkodierten Duplexsonografie bei der Diagnostik von Fingerarterienverschlüssen. VASA. 1998; 52 Suppl.):44
[16]Langholz J, Ladleif M, Blank B, Heidrich H, Behrendt C. Colour coded duplex sonography in ischemic finger artery disease—a comparison with hand arteriography. Vasa. 1997; 26(2):85–90
[17]Moradi A, Hajian, A: Presentation of the hypothenar hammer syndrome as a low incidence aneurysmal disorder of the ulnar artery. International Journal of Surgery Case Reports 85 (2021)
[18]Osiro S, Zurada A, Gielecki J, Shoja MM, Tubbs RS, Loukas M. A review of subclavian steal syndrome with clinical correlation. Med Sci Monit. 2012; 18(5):RA57–RA63
[19]Phillips DJ, Beach KW, Primozich J, Strandness DE, Jr. Should results of ultrasound Doppler studies be reported in units of frequency or velocity? Ultrasound Med Biol. 1989; 15(3):205–212
[20]Reisinger C, Gluecker T, Jacob AL, Bongartz G, Bilecen D. Dynamic magnetic resonance angiography of the arteries of the hand. A comparison between an extracellular and an intravascular contrast agent. Eur Radiol. 2009; 19(2):495–502
[21]Tatò, F: Upper extremity peripheral arterial disease Dtsch Med Wochenschr. 2020 Oct;145(20):1437–1442.
[22]Schlichting J, Faßbender D, Ziemssen P, et al. A.-brachialis-Stenose als Ursache einer Claudicatzio-Symptomatik des Armes. Vasa. 1999; 55 Suppl:95
[23]Schmidt WA, Krause A, Schicke B et al: Color Doppler ultrasonography of hand and finger arteries to differentiate primary from secondary forms of Raynaud’s phenomenon . J Rheumatol 2008 35 (8): 1591–8
[24]Shah SR, Wearden PD, Gaines BA. Pediatric peripheral vascular injuries: a review of our experience. J Surg Res. 2009; 153(1):162–166
[25]Gefäßsonografie. Internist (Berl). 2012; 53(3):298–308
[26]Stiegler H, Brandl R. Periphere arterielle Verschlußkrankheit: Stellenwert der Sonografie. Ultraschall Med. 2009; 30(4):334–363
[27]Stiegler H, Mietaschk A, Bilderling P, Rauh G, Tato F, Marshall M. [Vascular occlusive disease without atherosclerosis]. MMW Fortschr Med. 2012; 154(21):55–58, 60
[28]Toprak U, Selvi NA, Ateş A, et al. Dynamic Doppler evaluation of the hand arteries of the patients with Raynaud’s disease. Clin Rheumatol. 2009; 28(6):679–683
[29]Vollmar J. Rekonstruktive Chirurgie der Arterien. Stuttgart: Thieme; 1996
[30]Wittenberg G, Schindler T, Tschammler A, Kenn W, Hahn D. Wertigkeit der farbkodierten Duplexsonographie bei der Beurteilung von Armgefässen–Arterien und Hämodialyseshunts. Ultraschall Med. 1998; 19(1):22–27
[32]AIUM Practice Parameter for the Performance of Peripheral Arterial Ultrasound Examinations Using Color and Spectral Doppler Imaging © 2014 by the American Institute of Ultrasound in Medicine. Parameter developed in collaboration with the American College of Radiology (ACR) and the Society of Radiologists in Ultrasound (SRU)
[33]Crawford JD, Perrone KH, Jung E, Mitchell EL, Landry GJ, Moneta GL. Arterial duplex for diagnosis of peripheral arterial emboli. J Vasc Surg. 2016; 64(5):1351–1356
[34]Mauri G, Fresa M, Ferraris M, et al. Radiological anatomy of upper limb arteries and their anatomical variability: implications for endovascular treatment in critical hand ischemia. Minerva Cardioangiol. 2016; 64(6): 613–624
[35]Lee W, Kwon SB, Cho SH, Eo SR, Kwon C. Glomus tumor of the hand. Arch Plast Surg. 2015; 42(3):295–301
[36]Wahl U, Kaden I, Köhler A, Hirsch T. Vascular trauma of the hand—a systematic review. Vasa. 2019; 48(3):205–215
7.1.2 Veins of the Neck and Upper Extremities
General Remarks
With the increase in intensive care therapy and catheter-based diagnosis in the last 30 years, the relative frequency of venous thrombosis in the shoulder and arm regions has risen from 1% to 2% of all venous thrombosis cases to approximately 10%.2 , 3 Central venous catheters (CVCs) and malignancies, with or without port implantation, are the most frequent causes of subclavian vein thrombosis, followed by shoulder trauma and neck infections. Together they account for approximately 80% of all upper extremity venous thrombosis (Table 7.3). The remaining 20% of cases result from anatomic constrictions in the shoulder and neck regions, whether due to compression by structures abutting the vessels (venous thoracic outlet syndrome) or microtrauma to the vein wall relating to strenuous activity (effort-related thrombosis or Paget–von Schroetter syndrome).1
Table 7.3 Causes of upper extremity venous thrombosis
Pathogenesis | Risk factors |
Primary (20%) | |
Venous thoracic outlet syndrome | Compression of the subclavian vein by the first rib, clavicle, costoclavicular ligaments, or muscles (scalene muscles, subclavius) |
Paget–von Schroetter syndrome (effort-related thrombosis) | Microtrauma to the subclavian/axillary vein from repetitive overhead use of the arms in certain sports (weightlifting, swimming, wrestling, etc.) or occupations (painter, window washer) |
Secondary (80%) | |
Catheter-associated thrombosis | CVC, pacemaker, defibrillator, chemotherapy port, drug-induced, multiple failed access attempts |
Cancer-associated thrombosis | Paraneoplastic hypercoagulability, tumor compression |
Shoulder trauma or surgery | – |
Spread of head and neck infection | Lemierre’s syndrome |
Hypercoagulability | Pregnancy, thrombophilia |
Abbreviations: CVC, central venous catheter. |
Complications of upper extremity venous thrombosis in the form of symptomatic pulmonary embolism or post-thrombotic syndrome (PTS) are much less common than in the lower extremity, with respective rates of 6%–14% versus 32%–51% for pulmonary embolism5 and approximately 5% versus 50% for PTS. Also, the recurrence rates of upper extremity venous thrombosis at 1 year are less than half the rates reported in the lower extremity (2%–5% vs. 10%).2 , 6
Anatomy and Variants
Deep Arm Veins
The deep veins of the forearm (radial and ulnar veins, anterior and posterior interosseous veins), which are usually paired, join at the elbow with the distal portions of the paired brachial veins, which continue proximally as the unpaired axillary vein and subclavian vein. The axillary vein extends from the inferior border of the pectoralis major muscle to the clavicle and usually contains a venous valve.
As they run proximally, the axillary and subclavian veins pass through three anatomic constrictions that may become hemodynamically significant with changes in arm position. These sites are located:
•Between the pectoralis minor muscle and coracoid process
•Between the clavicle and first rib
•Between the anterior scalene and subclavius muscles and the first rib
Each subclavian vein unites with the internal jugular vein behind the right or left sternoclavicular joint, next to the artery, to form the right or left brachiocephalic vein. A venous valve is consistently present at the jugulosubclavian venous junction, sometimes called the “venous angle.” As a normal variant, the axillary vein and subclavian vein are duplicated in approximately 1% of the population. Fig. 7.20 illustrates the anatomic relations of the deep shoulder and arm veins and the sites of physiologic constriction.
Fig. 7.20 Arrangement of the deep shoulder and arm veins and physiologic constrictions. (a) Supra- and infraclavicular level. (b) Costoclavicular space.
Superficial Arm Veins
The cephalic vein is an important superficial vein that ascends in the lateral bicipital groove, pierces the fascia in the upper arm, and joins with the subclavian vein at the infraclavicular level in Mohrenheim’s space. The basilic vein is generally a large vessel that runs to the mid-upper arm in the medial bicipital groove, pierces the brachial fascia, and usually opens into the medial brachial vein.
Neck Veins
The internal jugular vein conveys most of the blood from the cerebral veins and dural sinuses. It exits the skull through the jugular foramen. From there it runs lateral to the internal and common carotid arteries to its union with the subclavian vein.
Examination Technique and Normal Findings
Inspection and Palpation
Ultrasonography should always be preceded by a clinical examination of the patient in a sitting or standing position. With the patient disrobed to the waist, visual inspection is done for side-to-side comparison of the neck, shoulders, and arms for asymmetry, differences in circumference, prominent superficial veins, and skin discoloration. Palpation is used to assess skin temperature and texture and to check for local tenderness if phlebitis is suspected. Outflow obstruction may be suggested by unilateral prominent veins in the hand and forearm with the arms outstretched or by prominent superficial veins in the shoulder region (Fig. 7.21).
Fig. 7.21 Acute subclavian vein thrombosis with complete occlusion of the vessel. (a) Clinical manifestations of acute subclavian vein thrombosis with swelling of the arm and prominent superficial veins in the shoulder region. (b) Complete occlusion of the subclavian vein (SCV). The terminal portion of the cephalic vein (CV) is also occluded. CL, clavicle.
Equipment
Transducer
A linear-array transducer with a transmission frequency of 5 to 12 MHz is suitable for imaging the superficial venous system including the deep veins of the arm and neck. A 3- to 5-MHz sector transducer may be necessary for imaging the axillary and subclavian veins in muscular patients. The recommended velocity scale is 10 to 12 cm/s with a pulse repetition frequency (PRF) of approximately 1,000 Hz.
Patient Positioning
The shoulder and arm veins can be scanned with the patient in a sitting or supine position. The supine position can provide better spontaneous filling of the proximal veins (internal jugular vein, subclavian vein).
Examination of Internal Jugular Vein
Ultrasound examination of the internal jugular vein requires a side-to-side comparison. The operator images each vein in continuous transverse scans by sliding the transducer (with plenty of gel to reduce probe pressure) from the base of the neck to the skull base while assessing luminal changes in response to respirations and the cardiac cycle. If the vessel cannot be imaged or appears only as a narrow slit, especially with shallow respiration, the examination should be continued during a Valsalva maneuver. Usually this will make the jugular vein lumen twice as large as the medially adjacent common carotid artery (Fig. 7.22). Not infrequently, the fine cusps of the venous valve at the jugulosubclavian junction can be seen just cranial to the costoclavicular joint. The internal jugular vein can be compressed with light probe pressure to exclude thrombosis.
Fig. 7.22 Luminal changes of the internal jugular vein (IJV) as a function of respiratory state. CCA, common carotid artery. (a) Narrow lumen with the patient sitting and breathing normally. (b) Luminal expansion in response to a Valsalva maneuver.
Examination of Subclavian Vein and Brachiocephalic Vein
Examination of the subclavian vein is somewhat more difficult due to anatomical constraints but can be accomplished with a longitudinal scan without probe pressure at the supraclavicular and immediate infraclavicular levels. Venous valves can be identified in this area, similar to the jugulosubclavian junction. The subclavian vein can be traced to its union with the internal jugular vein, at which point the brachiocephalic vein can usually be imaged in a longitudinal scan using a 2- to 5-MHz curved array transducer. Imaging is facilitated by having the patient perform a Valsalva maneuver (Fig. 7.23). The transition into the axillary vein is obscured by acoustic shadowing from the clavicle.
Activation of color Doppler at this point will demonstrate the respiratory modulation of venous flow, which is different from the patterns in the femoral vein: during inspiration, the negative intrathoracic pressure exerts a suction effect on the large central veins. Besides brief flow acceleration toward the heart, this causes a transient reduction of luminal diameter. This also explains the initial centripetal flow that occurs during a Valsalva maneuver; the flow approaches zero while the patient is bearing down and then resumes antegrade flow toward the heart when the patient exhales.
Fig. 7.23 Imaging the supraclavicular portions of the upper extremity deep veins. (a) Longitudinal scan of the subclavian vein during a Valsalva maneuver (VALS) displays the union of the internal jugular vein (IJV) and subclavian vein (SCV) to form the brachiocephalic vein (BCV). (b) The subclavian vein has a narrow lumen during spontaneous respiration. PL, pleura. (c) Color Doppler activated immediately after the Valsalva maneuver. CL, clavicle.
Next, the veins are scanned longitudinally with color Doppler while the spectrum is displayed. Attention should be paid to the optimum beam–vessel angle in a side-to-side comparison. Because the brachiocephalic vein cannot be compressed, flow signals are acquired from the subclavian vein and internal jugular vein in side-to-side comparison.4
The brachiocephalic vein can be imaged using a sector transducer (3–5 MHz) with a simultaneous color and spectral display. Next, the axillary vein, located medial to the artery, is imaged in cross section just below the clavicle. Normally, the subclavian vein and axillary vein will disappear from the image when light compression is applied.
Examination of the Axillary Vein and Deep Arm Veins
The deep veins are imaged in contiguous transverse scans by moving the transducer distally over the axilla and medial side of the upper arm to the elbow—or to the level of the wrist in select cases—while light compression is applied to exclude deep vein thrombosis. An abrupt caliber increase is often noted at the junction of the brachial vein with the axillary vein. This results from the generally unpaired course of the axillary vein and its union with the basilic vein, which usually has a large caliber.
Venous flow signals are obtained by placing the transducer in a longitudinal orientation over the axillary or brachial vein and switching to color mode. If very weak spontaneous flow signals are acquired, A sounds (A for “augmented”) can be obtained by compressing the forearm. They are recorded on both sides and compared.
Examination of Superficial Arm Veins
Imaging of the superficial veins (basilic and cephalic veins) is important in patients with thrombophlebitis or a peripheral venous catheter and for planning the placement of a hemodialysis fistula. The patient may be examined in a sitting or supine position (with the upper body elevated) and the arm abducted. The superficial veins are scanned from the forearm to the upper arm, continuing to their junction with the deep venous system while light, intermittent compression is applied. Because of their superficial location, a high-resolution 7- to 15-MHz transducer should be used with plenty of coupling gel (Fig. 7.24). This transducer can also record collateral flow as S sounds (S for “spontaneous”) associated with deep vein occlusion.
Fig. 7.24 Thrombophlebitis in a 34-year-old heavy smoker. (a) Acute, painful swelling of the hand. Scans in a water bath display the superficial dorsal veins of the hand. (b) Phlebitis of subcutaneous veins with inflammatory wall thickening and an occlusive thrombus (transverse scan). (c) Longitudinal scan.
Examination Time
The total time required for a complete ultrasound examination of the superficial and deep veins of the shoulder and arm regions, including side-to-side comparisons and documentation, is approximately 15 to 20 minutes.
Pathologic Findings
Thrombosis of the Deep Shoulder and Arm Veins
Causes
Thrombosis of the deep shoulder and arm veins is most often a complication of indwelling catheters (Fig. 7.25). Its occurrence depends on the following factors7:
Fig. 7.25 Catheter-related thrombosis in a 4-year-old girl who had undergone liver transplantation, had polycystic kidneys and renal failure requiring dialysis, and had Burkitt’s lymphoma. A Shaldon catheter for cytostatic therapy had been in place for 1 year. (a) Floating end of a thrombus in the internal jugular vein. (b) Thrombus forms a cast of the catheter after withdrawal, with a central lumen (yellow arrow). (c) Residual peripheral lumen demonstrated by color Doppler. (d) Thrombosis extends to the infraclavicular level. The echogenic thrombus is documented in B-mode during compression of the upper arm. (e) Thrombosis extends to the infraclavicular level. The echogenic thrombus is documented in B-mode during compression of the upper arm.
•The access vein
•Number of access attempts
•Catheter material
•Catheter lumen
•Catheter length
•Location of the catheter tip
•Prior catheter-based therapy
•Duration of catheter-based therapy
A cardiac pacemaker may also lead to acute upper extremity venous thrombosis after a period of years (Fig. 7.26). Simultaneous involvement of the internal jugular vein occurs in 20% to 60% of cases, depending on the patient cohort.
Fig. 7.26 Acute upper extremity venous thrombosis in a 75-year-old woman who had worn a cardiac pacemaker for 6 years. Several days ago she developed erysipelas after a bee sting, and her whole arm was swollen. Ultrasound reveals a long-segment occlusion of the infra- and supraclavicular part of subclavian vein (SCV) and a patent cephalic vein (CV), which enters the brachiocephalic vein at a typical site and is drained via the brachiocephalic vein (BCV). Yellow arrow shows pacemaker lead.
Lemierre’s syndrome is a special condition that predominantly affects young patients in previously good health. It occurs when a bacterial pharyngotonsillitis is complicated by septic thrombosis of the jugular vein that spreads to central veins.8 Clinically, patients present with high fever, swollen neck, dyspnea, and severe pain. Severe septic organ complications may arise if diagnosis is delayed (Fig. 7.27).
Fig. 7.27 Lemierre’s syndrome in a 22-year-old woman with a 2-week history of neck pain and tenderness and dyspnea on mild exertion, later accompanied by joint and back pain, limited head rotation, and swallowing difficulties. She also had a 3-day history of fever and chills. (a) Composite images show inhomogeneous thrombosis of the internal jugular vein (IJV), subclavian vein (SCV), cephalic vein (CV), and brachiocephalic vein (BCV) with thrombotic fixation of a venous valve (white arrow). (b) In a cross-sectional view, the lumen of the thrombosed jugular vein is several times larger than that of the common carotid artery (CCA). (c) Image after contrast administration shows absence of enhancement in the thrombus (Th) with pronounced inflammatory uptake in surrounding tissues. CCA, common carotid artery.
In up to one-third of patients with deep vein thrombosis in the shoulder and arm regions, a specific cause cannot be identified.9 These primary forms include Paget–von Schroetter syndrome, an effort-related thrombosis preceded by microtrauma to the subclavian or axillary vein usually caused by strenuous activity in high-performance sports (Table 7.3). This syndrome is distinguished in the literature from venous thoracic outlet syndrome due to compression in the costoclavicular space. Clinical experience shows that a continuum exists between these two mechanisms of primary upper extremity venous thrombosis.11
Clinical Features and Diagnosis
In contrast to lower extremity venous thrombosis, no evidence-based data are available on clinical pretest probability in the shoulder and arm regions. Pain, unilateral edema, and lack of an alternative diagnosis in patients with an indwelling venous catheter provide a sensitivity of 78% and a specificity of 64%. The various diagnostic procedures for upper extremity venous thrombosis that are used in everyday clinical practice are listed and compared in Table 7.4. The highest diagnostic accuracy has been reported for compression ultrasound with 97% sensitivity and 96% specificity in detecting upper vein thrombosis in the distal veins.10 For anatomic reasons, Doppler and color-coded duplex are used instead of compression sonography for evaluation of the brachiocephalic vein.12
Table 7.4 Diagnostic methods in patients with clinically suspected upper extremity venous thrombosis (Based on Di Nisio et al10)
Method | Sensitivity | Specificity |
Clinical presentation (pain, swelling, lack of alternative diagnosis) | 78% (68%–88%) | 64% (57%–72%) |
Positive D-dimer | 100% (78%–100%) | 14% (4%–29%) |
Compression ultrasound (CU) | 97% (90%–100%) | 96% (72%–97%) |
CU + Doppler | 91% (85%–97%) | 93% (80%–100%) |
MRI (TOF) | 71% (29%–96%) | 89% (52%–100%) |
MRI + contrast medium | 50% (12%–88%) | 80% (44%–97%) |
Abbreviations: MRI, magnetic resonance imaging; TOF, time-of-flight. |
Duplex sonography not only permits an early diagnosis but can also determine the efficacy of therapeutic actions on the thrombus. Additionally, ultrasound-guided catheterization of the central neck and arm veins can significantly reduce inadvertent arterial punctures with hematoma formation or dissection, shorten the insertion time, and significantly increase the success rate of catheter insertion on the first attempt.13 This is particularly important in pediatric and neonatal intensive care units (ICUs).14
The various provocative maneuvers like those used to detect an arterial thoracic outlet syndrome (hyperabduction test, elevated arm stress test) are of no value in determining the cause of subclavian vein thrombosis. They almost always compress the veins against the adjacent bone, tendons, or ligaments and may be omitted.
Sonography
Noncompressibility of the affected vein is the most important direct criterion for thrombosis detection in the B-mode image. Other findings are luminal enlargement and intraluminal echoes. Depending on the age and texture of the thrombus, it may be hypoechoic (more recent) or echogenic (older). The affected vein is surveyed in continuous transverse scans with light compression, and the proximal and distal ends of the thrombosis are identified if possible. With a partially occlusive thrombus, color Doppler can detect residual flow while peripheral compression is applied.
If thrombosis cannot be detected directly in the proximal portions of the subclavian vein or brachiocephalic vein, it is necessary to rely on indirect signs in veins distal to the affected site:
•Loss of respiratory modulation of venous flow, with little or no spontaneous flow
•Congested distal veins show luminal enlargement but are still compressible
Another sign is spontaneous flow (S sound), usually unaffected by respiration, in the ipsilateral superficial veins of the upper arm, shoulder, and neck regions. This collateral flow is detectable with color flow and pulsed-wave (PW) Doppler using a water path or copious gel and a high-frequency, linear-array transducer. The duplex ultrasound criteria for acute deep thrombosis are summarized in Table 7.5.
Table 7.5 Duplex ultrasound criteria for acute deep vein thrombosis
Major criteria | Minor criteria |
a. Noncompressibility | a. No respiratory changes in lumen size |
b. Increased lumen size | b. Absence of Doppler signal with a complete occlusion, or residual flow with an incomplete occlusion |
c. Internal echogenicity (hypoechoic to echogenic) | c. High collateral flow, independent of respiration, in superficial veins |
Follow-up and Evaluating Treatment Response
Color duplex sonography is also useful for the surveillance of upper extremity venous thrombosis. This may be done while the patient is on anticoagulant medication, during and after thrombolytic therapy (Fig. 7.28), or after vascular surgery.
Fig. 7.28 Evaluating response to thrombolytic therapy. (a) Spontaneous thrombosis of the left subclavian vein and axillary vein in a thin, 25-year-old male. (b) Recanalization occurred within 12 hours after placement of a thrombolysis catheter with continuous thrombolysis. (c) Follow-up at 1 month shows a patent subclavian vein. Cl, clavicle. (d) Follow-up at 3 months shows a patent subclavian vein. Yellow arrow shows venous valve contours in a power Doppler view of the supraclavicular subclavian vein.
An older venous thrombosis in the arm may present any of the following color duplex signs:
•Partial compressibility of the vein
•Thickened, sometimes echogenic vein wall with decreased expansion during a Valsalva maneuver
•Decreased luminal diameter in a side-to-side comparison
•Doppler and color duplex detection of valvular incompetence
•Decreased, respiration-dependent axillary vein flow in a side-to-side comparison
•Increased collateral flow in superficial veins (S sound) in a side-to-side comparison
•A diminished A sound from the axillary vein in response to peripheral compression
Phlebitis (Superficial Venous Inflammation)
Etiology
Inflammations of the superficial arm veins usually have an iatrogenic cause. The injection of medications that damage the endothelium or catheter-based therapies can irritate the vein wall, promoting the secondary development of thrombosis. In rare cases, however, phlebitis may be the manifestation of a systemic disease and may occur in the setting of vasculitis such as in Behçet’s syndrome, in connective tissue diseases, in thrombangiitis obliterans (Fig. 7.24), giant cell arteritis, or in association with neoplasms.
Findings
Phlebitis can be diagnosed clinically with reasonable confidence and confirmed by compression ultrasound. Analogous to the deep veins, examination in acute cases reveals an enlarged, noncompressible vessel that is tender to pressure. Duplex sonography can additionally define the precise extent of thrombophlebitis and detect possible thrombus extension into the deep venous system.
Recommendations for documentation are based on the guidelines issued by the German Society for Ultrasound in Medicine (DEGUM) as well as on the AIUM Practice Parameter for the Performance of Peripheral Venous Ultrasound Examinations, published in 2020 by the American Institute of Ultrasound in Medicine.15
Normal Findings
The following documentation is recommended for normal findings:
•Bilateral transverse scans of the internal jugular vein in the lower third of the neck with functional residual capacity breath-hold and, if necessary, during a Valsalva maneuver.
•Longitudinal scan of the subclavian and axillary veins just below the clavicle with simultaneous acquisition of a respiratory-modulated Doppler spectrum; also scans of vessel cross sections with and without compression.
Acute Thrombosis
Direct Signs of Thrombosis
•Scans of vein cross sections with and without compression
•Document internal echoes at the thrombosis site (Fig. 7.28 , Fig. 7.29).
Indirect Signs of Thrombosis
•Absent or diminished respiratory modulation
•Absence of spontaneous flow in deep veins
•High collateral flow in subcutaneous veins
Describe the extent of thrombosis aided by a small drawing if necessary.
Fig. 7.29 Venous thrombosis in a 71-year-old woman with metastatic breast cancer and a chemotherapy port on the right side. Now she presented with acute, painful swelling of the left side of the neck and left arm. (a) Freely mobile venous valve (VV) is seen opposite the port tip (Po) with no evidence of thrombosis on the right side. (b) Acute, inhomogeneous thrombosis of the left brachiocephalic vein (BCV), internal jugular vein (IJV), and subclavian vein (SCV).
Older Thrombosis
These recommendations apply when documenting older venous thrombosis with signs of recanalization:
•Obtain comparative cross-sectional views with and without compression.
•State residual thrombus size in millimeters.
•If necessary, document high-level internal echoes in longitudinal section.
•Document venous flow with color and spectral Doppler in longitudinal section.
A side-to-side comparison is essential for evaluating venous drainage.
Phlebitis
In case of phlebitis, document the proximal end in cross section and, where applicable, document thrombus extension into the deep venous system in longitudinal section.
Comparison of Color Duplex Sonography with Other Modalities
While venous thrombosis in the shoulder and arm regions can be diagnosed from clinical criteria with a reported sensitivity of 50%, or 78% in patients with an indwelling venous catheter or pacemaker, compression ultrasound provides a sensitivity and specificity of 97% and 96%, respectively, based on a systematic review (Table 7.4)16 Interestingly, the additional use of Doppler ultrasound does not improve diagnostic quality.
Problems arise when dealing with nonocclusive thrombosis in proximal veins that are difficult to insonate. A definitive diagnosis in some cases may require the addition of contrast venography.17 It should be noted that catheter-induced thrombosis usually has a more central location than spontaneous thrombosis in upper extremity veins.7
Although not validated by study data, the use of a diagnostic algorithm is helpful in detecting or excluding upper extremity venous thrombosis in clinically suspicious cases (Fig. 7.30).
If ultrasound findings are inconclusive, a different imaging modality (magnetic resonance imaging [MRI], computed tomography [CT], or venography) should be used to permit confident detection or exclusion of disease. Moreover, MRI or CT is indispensable for investigating thoracic lesions in patients with suspected tumor-associated subclavian vein thrombosis. Using the Wells score for venous thrombosis, study by van Es et al tested an algorithm consisting of clinical pretest probability, D-dimer, and ultrasound.18 Age-adjusted D-dimer testing is likely to safely increase the efficiency of the diagnostic algorithm, but this approach needs prospective validation.
Fig. 7.30 Algorithm for the investigation of clinically suspected upper extremity venous thrombosis.
Advantages of Duplex Sonography
Duplex sonography has advantages over venography in the diagnosis of phlebitic vascular occlusions and in the differential diagnosis of unexplained arm edema, as it can reliably detect hematomas, joint effusions, arterial injuries after fractures, vascular malformations, compartment syndrome, and soft-tissue masses causing extrinsic vascular compression.
Conclusion
Given the varying accuracies of available diagnostic methods, we recommend the algorithm in Fig. 7.30 for the investigation of upper extremity venous thrombosis. It should be kept in mind that the accuracy of duplex sonography depends critically on operator’s experience, and that venography should also be performed by an experienced radiologist or vascular specialist and reserved for select indications. The methods shown in the algorithm are not competitive but should be viewed as complementary from a diagnostic, therapeutic, and economic standpoint.
References
[1]Chunilal S. Upper Extremity Deep Vein Thrombosis: Current Knowledge and Future Directions. Semin Thromb Hemost. 2021 Sep;47(6):677–691
[2]Kucher N. Clinical practice. Deep-vein thrombosis of the upper extremities. N Engl J Med. 2011; 364(9):861–869
[3]Kraaijpoel N, van Es N, Porreca E, Büller HR, Di Nisio M. The diagnostic management of upper extremity deep vein thrombosis: a review of the literature. Thromb Res. 2017; 156:54–59
[4]Khan O, Marmaro A, Cohen D. A review of upper extremity deep vein thrombosis Postgrad Med2021 Aug;133(sup1):3–10
[5]Yamashita Y, Morimoto T, Amano H, et al. COMMAND VTE Registry Investigators. Deep vein thrombosis in upper extremities: clinical characteristics, management strategies and long-term outcomes from the COMMAND VTE Registry. Thromb Res. 2019; 177:1–9
[6]Levy MM, Albuquerque F, Pfeifer JD. Low incidence of pulmonary embolism associated with upper-extremity deep venous thrombosis. Ann Vasc Surg. 2012; 26(7):964–972
[7]Linnemann B, Lindhoff-Last E. Risk factors, management and primary prevention of thrombotic complications related to the use of central venous catheters. Vasa. 2012; 41(5):319–332
[8]Astradsson T, Ekspong L, Norlander T. [Lemierre syndrome is a forgotten disease that primarily affects young people. Early antibiotic treatment can prevent fatal outcome]. Lakartidningen. 2013; 110(8): 413–415
[9]Heil J, Miesbach W, Vogl T, Bechstein WO, Reinisch A. Deep vein thrombosis of the upper extremity. Dtsch Arztebl Int. 2017; 114(14): 244–249
[10]Di Nisio M, Van Sluis GL, Bossuyt PM, Büller HR, Porreca E, Rutjes AW. Accuracy of diagnostic tests for clinically suspected upper extremity deep vein thrombosis: a systematic review. J Thromb Haemost. 2010; 8(4):684–692
[11]Modi BP, Chewning R, Kumar R: Venous thoracic outlet syndrome and Paget-Schroetter syndrome. Semin Pediatr Surg. 2021 Dec;30(6):151125
[12]Patel P, Braun C. et al Diagnosis of deep vein thrombosis of the upper extremity: a systematic review and meta-analysis of test accuracy. Blood Adv. 2020 Jun 9;4(11):2516–2522
[13]Rabindranath KS, Kumar E, Shail R, Vaux EC. Ultrasound use for the placement of haemodialysis catheters. Cochrane Database Syst Rev. 2011; 11(11):CD005279
[14]Guilbert AS, Xavier L, Ammouche C, et al. Supraclavicular ultrasoundguided catheterization of the subclavian vein in pediatric and neonatal ICUs: a feasibility study. Pediatr Crit Care Med. 2013; 14(4):351–355
[15]AIUM Practice Parameter for the Performance of a Peripheral Venous Ultrasound Examination. J Ultrasound Med . 2020 May;39(5):E49-E56
[16]Lim W, Le Gal G, Bates SM, et al. American Society of Hematology 2018 guidelines for management of venous thromboembolism: diagnosis of venous thromboembolism. Blood Adv. 2018; 2(22):3226–3256
[17]Hach W, Mumme A, Hach-Wunderle V. Venenchirurgie – operative, interventionell und konservative Aspekte. 3. Aufl. Stuttgart: Schattauer; 2013:276–283
[18]van Es N, Bleker SM, Di Nisio M, et al. ARMOUR study investigators. A clinical decision rule and D-dimer testing to rule out upper extremity deep vein thrombosis in high-risk patients. Thromb Res. 2016; 148:59–62
7.2.1 Arteries
General Remarks
Color duplex sonography can be used routinely for examinations of the pelvic and lower extremity arteries, where it permits fast and accurate localization of steno-occlusive lesions. Pulsed Doppler can be used to assess hemodynamic significance, and clinical and duplex findings can be correlated to determine the relevance of a stenosis and its therapeutic implications.
Owing to constant advances in sonographic techniques and operator training, color duplex sonography is achieving the accuracy level of magnetic resonance angiography (MRA) or intra-arterial digital subtraction angiography (DSA) in the lower extremity arteries.11 As the modality of first choice in the investigation of peripheral arterial occlusive disease (PAOD), it can reliably detect angiographically difficult lesions such as internal iliac artery stenosis with gluteal claudication, thigh pain due to proximal stenosis of the profunda femoris artery, or plantar claudication due to the occlusion of pedal arteries. However, this requires comprehensive operator training. Only a physician experienced in vascular disease can effectively utilize this method (an essential part of the PAOD algorithm), make a diagnosis, make appropriate therapeutic recommendations, and avoid a confusing, “colorful” description of findings, which could prompt costly and sometimes stressful testing and may even necessitate additional color duplex scans for clarification.
This section deals with basic anatomical and hardware aspects of lower extremity ultrasound, examination techniques, patterns of findings, and sources of error. The role of color duplex sonography in lower extremity arterial diseases will be explored and its capabilities compared with other imaging modalities.
Anatomy and Variants
Aorta and Iliac Arteries
The descending aorta enters the abdomen through the aortic aperture at the level of the T12 vertebra. Its diameter is approximately 16 to 25 mm on average and depends on several factors such as body height and gender. It descends in a straight course, passing to the left of the midsagittal plane and anterior to the vertebral bodies. At the level of the L4 vertebral body it divides into the two common iliac arteries, each measuring 10 to 12 mm in diameter (Fig. 7.31).
Approximately 4 to 8 cm distal to the aortic bifurcation, the internal iliac artery branches from the common iliac artery to supply the pelvic organs, pelvic wall, perineal region, and gluteal muscles. Distal to the iliac bifurcation, the common iliac artery continues as the external iliac artery. This vessel is approximately 8 to 10 mm in diameter and runs along the iliopsoas muscle in the pelvis. Proximal to the inguinal ligament it gives off the deep circumflex iliac artery; distal to that ligament it is gives off the inferior epigastric artery, which passes to the anterior abdominal wall, as well as the superficial circumflex iliac artery (Fig. 7.31). Very rarely, claudication symptoms will draw attention to atresia of the external iliac artery caused by a persistent fetal sciatic artery.
Fig. 7.31 Anatomy of the pelvic arteries. (a) General view of the abdominal aorta and pelvic arteries (with abdominal organs removed). (Reproduced with permission from: Schuenke M, Schulte E, Schumacher U. THIEME Atlas of Anatomy. General Anatomy and Musculoskeletal System. Illustrations by Voll M and Wesker K. © Thieme 2020.)
Fig. 7.31 (Continued) (b) Course and branching pattern of the femoral artery. (Reproduced with permission from: Schuenke M, Schulte E, Schumacher U. THIEME Atlas of Anatomy. General Anatomy and Musculoskeletal System. Illustrations by Voll M and Wesker K. © Thieme 2020.)
Femoral Arteries
Although anatomical nomenclature does not recognize the term, vascular specialists call the continuation of the external iliac artery distal to the inguinal ligament the common femoral artery, which runs lateral to the common femoral vein and medial to the femoral nerve. The joint line of the hip marks its junction with the external iliac artery on angiograms. Approximately 3 to 5 cm below the inguinal ligament, the artery divides into the superficial femoral artery and profunda femoris artery (deep femoral artery).
The profunda femoris artery runs posterolaterally in 48% of cases, posteriorly in 40%, and medially in 10% to supply the muscles of the thigh. Just past its origin it gives off two large branches: the medial and lateral circumflex femoral arteries, each named for its course. Both vessels may branch directly from the common femoral artery, a pattern found in 15% to 18% of cases (Fig. 7.31). The terminal branches of the profunda femoris artery, especially the lateral circumflex femoral artery, connect with smaller side branches of the articular rete about the knee, the genicular anastomosis. The lateral circumflex femoral artery provides an important source of collateral blood flow in patients with femoral artery occlusive disease. As an anatomic variant, branches of the profunda femoris artery may arise directly from the superficial femoral artery.51
The superficial femoral artery first runs anteromedially and then medially down the thigh, approaching the femoral shaft in its middle and distal thirds. At the junction of the middle and distal thirds of the femur, it enters the adductor canal (of Hunter), accompanied by the superficial femoral vein and saphenous nerve, and turns posteriorly. The adductor canal is formed by an anterior aponeurosis composed of adductor longus and magnus fibers (vasoadductor membrane) and the posterior portions of those two muscles (Fig. 7.32).
Fig. 7.32 Anatomy of the lower extremity arteries. (a) Posterior view. (b) Anterior view. (Reproduced with permission from: Schuenke M, Schulte E, Schumacher U. THIEME Atlas of Anatomy. General Anatomy and Musculoskeletal System. Illustrations by Voll M and Wesker K. © Thieme 2020.)
Popliteal and Infrapopliteal Arteries
At the level where the femoral artery intersects the medial femoral shaft on radiographs, the superficial femoral artery becomes the popliteal artery, which extends from the inferior border of the adductor canal (tendinous adductor hiatus) to the inferior border of the popliteus muscle. It measures 4 to 6 mm in diameter. It has a total length of 12 to 18 cm and is divided into three segments (P I–III):
•The proximal segment (P I) extends from the adductor hiatus to the gastrocnemius tunnel.
•The middle segment (P II) occupies the gastrocnemius tunnel just posterior to the joint space of the knee.
•The distal segment (P III) extends from the end of the gastrocnemius tunnel to the tendinous arch of the soleus muscle. There the artery gives off three smaller side branches (middle, inferior medial, and inferior lateral genicular arteries), which form the genicular anastomosis (Fig. 7.32).
The first crural branch of the popliteal artery is the anterior tibial artery. After passing through the interosseous membrane with a proximal diameter of 3 to 5 mm, it runs sharply downward between the extensor muscles of the lower leg and crosses the front of the ankle joint to become the dorsalis pedis artery. Distal to the origin of the anterior tibial artery, the vessel becomes the tibioperoneal trunk, which descends for 1 to 2 cm before branching into the posterior tibial artery and peroneal (fibular) artery. Trifurcation of the popliteal artery into all three infrapopliteal arteries at one level may be encountered as a rare variant (Table 7.6).
Table 7.6 Variations of the popliteal and infrapopliteal arteries (Based on Kil and Jung34)
Variations | Extremities (%) |
No deviations from normal | 1,543 (88.2) |
ATA origin above the knee joint | 60 (3.5) |
Trifurcation at one level | 53 (3.0) |
Hypoplastic or aplastic PTA collateralized distally by the PA | 32 (1.8) |
Hypoplastic ATA collateralized distally by the PA | 25 (1.4) |
PTA origin above the knee joint | 17 (1.0) |
High PTA origin with common anterior fibular trunk | 14 (0.8) |
Hypoplastic ATA and PTA collateralized distally by the PAa | 3 (0.2) |
Abbreviations: ATA, anterior tibial artery; PA, peroneal artery; PTA, posterior tibial artery. a“Dominant peroneal artery.” Given the special anatomic location of the peroneal artery, the collateral supply to a hypoplastic PTA leads to falsely elevated Doppler pressure readings that can mimic medial calcific sclerosis. |
The posterior tibial artery is generally the largest of the infrapopliteal vessels. It runs downward between the soleus and deep flexor muscles. The peroneal artery ends at the ankle in the lateral malleolar network (Fig. 7.32).
Anatomic variants of both the popliteal and infrapopliteal arteries are not uncommon and are found in approximately 12% of the population.34 The most common variants are described in Table 7.6.
Arteries of the Foot
The dorsalis pedis artery gives off the small arcuate artery from which the dorsal metatarsal arteries arise, while its main portion continues distally between the first and second metatarsals as the deep plantar artery and communicates with the deep plantar arch. The posterior tibial artery divides into the medial and lateral plantar arteries. The latter forms the deep plantar arch from which the plantar metatarsal arteries and common plantar digital arteries arise.
Similar to the hand, numerous variations may be found in the arterial supply to the foot. The deep plantar arch, and thus the toes, are supplied primarily by the dorsalis pedis artery in 27% of cases and primarily by the posterior tibial artery in 26%. In 19% of cases the posterior tibial artery and anterior tibial artery supply the fourth and fifth toes and the first through third toes, respectively (Fig. 7.33).51
Fig. 7.33 Arteries of the foot. (a) Dorsal view. (Reproduced with permission from: Schuenke M, Schulte E, Schumacher U. THIEME Atlas of Anatomy. General Anatomy and Musculoskeletal System. Illustrations by Voll M and Wesker K. © Thieme 2020.)
Fig. 7.33 (Continued) (b) Plantar view. (Reproduced with permission from: Schuenke M, Schulte E, Schumacher U. THIEME Atlas of Anatomy. General Anatomy and Musculoskeletal System. Illustrations by Voll M and Wesker K. © Thieme 2020.)
Examination Technique
Preliminary Workup
Color duplex sonography is included in the diagnostic algorithm for diseases of the pelvic and lower extremity arteries (Fig. 7.34). The algorithm begins with the history, visual inspection, palpation, and auscultation, supplemented by the Ratschow’s test and, if necessary, auscultation after exercise to aid further differentiation. These tests plus the measurement of ankle artery Doppler pressures with and without exercise will detect clinically significant PAOD in > 95% of cases. This enables the examiner to classify the PAOD clinically as:
•Pelvic type
•Thigh type
•Calf type
•Foot type
Color duplex sonography can be used effectively in this setting as the imaging modality of first choice for the accurate evaluation of PAOD and directing the use of possible additional diagnostic and therapeutic modalities.
Fig. 7.34 Diagnostic algorithm for peripheral arterial occlusive disease (PAOD) (based on the S3 guideline69).
Necessary Equipment
Transducers
To ensure useful morphologic and Doppler data acquisition from vessels at depths ranging from a few millimeters to 15 to 20 cm, the duplex system should be equipped with linear and curved-array transducers with transmission frequencies of 3 to 17 MHz so that the frequency can be tailored to the structure under investigation. Modern multifrequency transducers can also provide variable frequencies in each mode so that the highest possible transmission frequency can be used for a given scanning depth. The frequencies used for B-mode imaging are higher than those used for Doppler scans.44 A color Doppler and pulsed Doppler mode is essential in the detection of steno-occlusive disease.
Pure B-mode imaging is as important for the detection of early atherosclerotic vessel wall changes, uniformly hypoechoic vasculitic wall structures, wall changes in medial calcific sclerosis, and rare cystic wall degeneration as it is for the evaluation of perivascular tissues and neighboring structures (nerves, veins, lymph nodes, muscles). Because the lower extremity vessels are not placed very deeply in the leg, despite a highly variable leg circumference, linear-array transducers operating at 5 to 10 MHz are generally used. Often a 2- to 4-MHz curved-array transducer will have to be used in very obese patients or patients with heavy vascular calcification.
Positions of the Patient and Operator
Comfortable Environment
Both the patient and operator should be able to assume a comfortable position for the examination. Otherwise, both the performance and quality of sectional imaging would be compromised, especially in lengthy examinations. This requirement includes a room temperature that is comfortable for the patient, since a cold room may lead to shivering artifacts and increased peripheral vascular resistance, which could distort the findings.
Patient Preparation
The bladder should be empty for imaging the pelvic arteries, since transducer pressure on a distended bladder would cause discomfort to the patient. The patient should rest for approximately 10 minutes before the examination, as any muscular effort during that time would distort the Doppler spectrum due to hyperemia.
Positioning and Operator Position
The patient is positioned supine for examination of the iliac and femoral arteries as well as the anterior and posterior tibial arteries. The operator sits on the patient’s right side and maneuvers the transducer with the right hand. The left hand controls the ultrasound machine, which is next to the examination table. Both the stool and examination table should have an adjustable height so that the operator can handle the transducer in a relaxed position. This is particularly important in lengthy examinations to avoid excessive tension in the arm and shoulder muscles, which could affect the quality of the examination.
The patient should be placed in a prone position for examination of the popliteal artery, tibioperoneal trunk, and peroneal artery, as these vessels are more easily identified when the transducer is on the back of the leg. The same applies to patients with claudication symptoms in the gluteal muscles. In this case the superior and inferior gluteal arteries, terminal branches of the internal iliac artery, are identified by scanning with a 3- to 5-MHz curved-array transducer at the level of the greater sciatic foramen (see Fig. 7.49). The patient lies on his or her stomach. If the patient is unable to turn to a prone position, lateral position is a possible alternative. A fallback option is to scan the popliteal artery in the supine position with the knees flexed and the legs drawn up. If necessary, an assistant can elevate the legs of the supine patient to facilitate transducer access to the popliteal fossa and calf. Any difficulties encountered during the examination should be noted in the ultrasound report.
Examination Protocol
Duplex sonography used within the diagnostic algorithm for PAOD can supply information on the morphology and function of arterial vascular segments.
▶ B-mode. It is prudent to begin the examination with a longitudinal B-mode scan. A normal artery should present an echo-free lumen.
Note
For longitudinal scans, orient the transducer so that the cranial end of the artery is always displayed on the left side of the image.
For transverse (cross-sectional) scans, the patient’s right side should be displayed on the left side of the image.
Analogous to documentation in CT, the image is displayed as if the examiner were “looking into the patient from below.”
The procedure and scope of the examination depend on the clinical question:
•For a suspected flow obstruction proximal to the inguinal ligament: The abdominal aorta, pelvic arteries, and common femoral artery should be examined over their full length in continuous longitudinal scans.
•For a presumed obstruction distal to the inguinal ligament: The common femoral artery, superficial femoral artery, origin of the profunda femoris artery, and femoropopliteal vessels should be scanned over their full length. The infrapopliteal arteries should also be scanned if required.
Because renal artery stenosis (up to 40%) and abdominal aortic aneurysms (up to 15%) are common findings, depending on the severity of PAOD, the vessels of the abdomen and lower extremities should be examined together when vascular status is determined. Usually this will not significantly prolong the examination time. Aortic aneurysm and renal artery stenosis are both independent predictors of increased cardiovascular mortality.42 , 57
▶ Color Doppler. The color-coded imaging of blood flow has greatly facilitated examination of the extremity arteries. Arterial flow should be coded in red, venous flow in blue. In color Doppler sonography, attention should be given to brightly colored areas, aliasing, turbulence, and changes in flow direction (color reversal) that signal an increased mean flow velocity or mark the site of a draining or feeding vessel (Fig. 7.35). With correct machine settings (pulse repetition frequency [PRF], gain, and color filter adjusted to give a homogeneous red color in normal arterial segments), the color-coded image will shorten the examination time since the operator can quickly detect areas of stenosis and evaluate them on the basis of direct and indirect stenosis criteria (Fig. 7.35). Occlusion is marked by an abrupt cutoff of color flow signals. Often this is associated with inflow/outflow signals from small collateral vessels that are not coaxial with the main vessel. This provides a differentiating feature from the color dropout caused by calcified plaque.
Fig. 7.35 Incidental findings during the investigation of peripheral arterial occlusive disease (PAOD). (a) High-grade stenosis of the superior mesenteric artery is indicated by color artifacts (yellow arrow) with a “confetti sign” (see Chapter 3). (b) Left renal artery stenosis (white arrow shows aliasing).
▶ Length of examination. The examination time necessary depends in large part on operator’s experience and patient-specific factors. It also depends on whether the findings are normal or abnormal. While clinical vascular status itself is a strong indicator of vascular health (Fig. 7.34) and can be confirmed in minutes by duplex scanning, complex vascular findings may require examination time of 30 to 45 minutes or more. This is especially true in patients with poor renal function (generalized vessel wall calcification, contraindication to contrast media) and patients with nonatherosclerotic vascular disease (compression syndromes, vasculitis, vascular malformations, acral ischemia syndrome), and there is ever-present need to think “outside the box” (glomus tumor, carpal or tarsal tunnel syndrome, spinal claudication). Regardless of the region under investigation, color duplex sonography should always attempt to answer the question posed to the vascular specialist, aid in selecting any further tests, and direct the decision for invasive or conservative vascular therapy.
Pelvic Arteries
With the patient lying in a relaxed supine position, the examination begins with B-mode imaging of the aorta in longitudinal and transverse sections. Color Doppler is then superimposed over the longitudinal gray scale image to check for flow irregularities. In this way the proximal renal arteries can be evaluated quickly and confidently even in nonfasted patients (Fig. 7.35).
B-mode imaging of the iliac arteries begins in the groin. Orientation is established from the double contours of the external iliac artery and vein (Fig. 7.36), which can be traced up to the aortic bifurcation in longitudinal section. Like the aorta, the artery can be traced continuously in the proximal direction using the color and Doppler modes while documenting the Doppler frequency spectrum, which normally has a triphasic waveform. When stenosis is detected, flow parameters are determined from the pre-, intra-, and poststenotic systolic Doppler spectra, and significant stenoses are confirmed by indirect flow parameters in the common femoral artery.
Fig. 7.36 Normal findings in the external iliac artery and vein and their internal branches, which show “opposite” color encoding. EIA, external iliac artery; EIV, external iliac vein; IIA, internal iliac artery; IIV, internal iliac vein.
As Fig. 7.31 illustrates, the internal iliac artery often arises from the “deepest” (most posterior) part of the common iliac artery. Imaging the origin of the internal iliac artery is important in that it may supply information on vascular impotence in males or on the rare finding of vascular claudication of the gluteal musculature in a patient with palpable femoral artery pulses. To evaluate the gluteal arteries, the examination is performed with the patient in prone position. This type of stenosis or occlusion is also accessible to endovascular therapy.
Lower Extremity Arteries
As in the pelvis, examination of the femoral circulation begins in the groin. With the patient in a relaxed supine position with the leg in slight external rotation, scanning is continued to the distal segments of the superficial femoral artery in the adductor canal. The transducer is initially positioned for a longitudinal view of the common femoral artery and its bifurcation with the origins of the superficial femoral and profunda femoris arteries. The bifurcation is a site of predilection for early angiopathic vessel-wall changes and should be routinely assessed for complications after a catheterization procedure (pseudoaneurysm, occasional dissection, or arteriovenous [AV] fistula), even in patients with mild complaints. Note that the level of catheter insertion is highly variable and can range from the distal external iliac artery to the proximal superficial femoral artery.
The femoral bifurcation is first imaged in longitudinal section. The origins of the profunda femoris artery and superficial femoral artery are separately identified, giving particular attention to possible variations in their origin. The profunda femoris artery should be traced for several centimeters, continuing past the origins of the first muscular branches in the thigh. This can be done only in color mode and should be combined with the acquisition of Doppler spectra. This method can easily detect sites of proximal stenosis (Fig. 7.37), which are difficult to detect by indirect methods and may even be missed on anteroposterior (AP) angiograms due to overlying structures.
Fig. 7.37 Angiographic and color duplex findings in a 78-year-old woman scheduled for a femoropopliteal bypass. (a) Angiography confirms occlusion of the superficial femoral artery. (b) Duplex sonography reveals a high-grade proximal stenosis of the profunda femoris artery (black arrow in a) with a “seagull cry” in the frequency spectrum (white arrows). As a result of this lesion, which had been missed by angiography, the proposed bypass was changed to a profundaplasty.
Next, the femoral artery is traced distally in one plane as it courses from an anteromedial to a posterolateral position in the thigh. Special attention is given to the femoropopliteal junction, which is a site of predilection for stenosis. With the thigh in slight external rotation, this area is traced into the P1 segment of the popliteal artery.
For scanning of the popliteal artery, the patient can be placed in a prone position with the knee in slight flexion, aided by a foot rest, or in a sitting position with the lower legs hanging down. The femoropopliteal vascular segment is deeply embedded in the muscles and is a frequent site of stenosis or collateral reconstitution distal to a femoral artery occlusion. In some cases, a 3- to 5-MHz curved-array transducer is useful for better penetration. Besides noting congenital variants, it may be necessary to image the popliteal artery to its junction with the individual crural arteries, depending on the clinical question.
If the patient’s mobility is limited, the anterior and posterior tibial arteries can be traced continuously while the patient is supine, moving the transducer laterally to scan the peroneal artery, after the femoral circulation has been examined. For the investigation of plantar claudication or acral ischemia, the dorsalis pedis artery and its terminal branches should be scanned with a 5- to 17-MHz linear-array transducer. Additionally, the distal portions of the posterior tibial artery should be scanned from the plantar surface of the foot. Doppler frequency spectra should be sampled for the detection of proximal or distal occlusions.55
Normal Findings
Pelvic Arteries
B-mode
The normal pelvic arteries appear as pulsating structures with smooth borders and no intraluminal echoes. The sonographically determined diameter of the external iliac artery is approximately 8 to 10 mm. The radiographic diameters reported for the common iliac artery average 8.3 mm (left side) and 8.9 mm (right side).36 The normal intima-media thickness in the common femoral artery is not greater than 0.7 mm. Besides caliber measurements, B-mode imaging is recommended for the exclusion of true aneurysms in the aorta and iliac arteries.
Doppler Measurements
For orientation purposes, typical triphasic Doppler spectra in normal cases are recorded from both common femoral arteries and the sides are compared. As a general rule, normal findings in the extremity arteries should meet the criteria listed below.
The extremity arteries normally have a triphasic spectral waveform with:
•A narrow frequency band
•A steep systolic upstroke (acceleration)
•A narrow peak
•A rapid downstroke (deceleration)
•A reverse flow component in early diastole, approximately one-fifth the amplitude of systolic forward flow (DIP = diastolic inverse peak)
•Brief forward flow in late diastole
This latter characteristic occurs when the primary pulse wave is reflected in the periphery, travels back through the arterial system, is reflected from the momentarily closed aortic valve, and is redirected peripherally, depending on aortic compliance, to produce a second forward peak (“ping pong” phenomenon).7 This may not occur as a normal phenomenon in elderly patients.
The waveform pattern of extremity arteries results mainly from the high peripheral resistance that prevails in the terminal vascular bed (skin and muscles) of the extremities at rest. The wave amplitudes diminish distally due to the decay of flow velocity that occurs in multiple in-line tubes (Fig. 7.38). A triphasic Doppler spectrum in the common femoral artery, with no evidence of flow disturbances, excludes a high-grade stenosis at the pelvic level with reasonable confidence (Fig. 7.38, see Fig. 7.48).
Fig. 7.38 Triphasic Doppler velocity spectra with diminishing amplitudes. (a) Common femoral artery. (b) Superficial femoral artery. (c) Popliteal artery. (d) Pedal arteries.
While it is pointless to report peak systolic or diastolic flow velocities of the extremity arteries in patients with normal findings, velocity ratios should always be correlated with clinical findings when evaluating stenoses. The “normal values” for peak velocities in different vascular regions are listed in Table 7.7 to provide a rough guide. These values are strictly for orientation purposes and are subject to large intra- and interindividual variations.
Lower Extremity Arteries
B-mode
Under physiologic conditions, B-mode is used to determine vascular course and diameter. This involves the exclusion of aneurysmal dilatation, atherosclerotic plaques, uniformly hypoechoic vasculitic wall deposits, possible medial calcific sclerosis in long-term diabetics, or detection of stents. Sonographically determined vascular diameters are approximately 8 to 10 mm for the common femoral artery, 6 to 8 mm for the proximal superficial femoral artery, and 5 to 7 mm for the distal part of the artery, values that show good agreement with anatomically measured calibers.28
Doppler Measurements
While the above flow velocities provide a guideline for normal findings in the femoral and popliteal arteries, normal findings in the infrapopliteal arteries are comparable only with regard to spectral waveforms. A triphasic or biphasic waveform in the pedal arteries at the malleolar level virtually excludes a hemodynamically significant flow obstruction in the more proximal vessels. On the other hand, vasospasms (e.g., in response to a low room temperature) may reduce systolic flow amplitudes to such a degree that a “stump waveform” is produced despite arterial patency.
Flow resistance in the cutaneous arteries affects the infrapopliteal arteries more than it does the larger limb arteries. This flow resistance is highly variable, particularly in children, and is affected by emotional factors as well as room temperature. Holodiastolic forward flow may be a normal finding caused by increased cutaneous blood flow in a warm room and should not be falsely attributed to decreased poststenotic resistance. Similarly, systolic–diastolic flow may be increased due to an inflammation of the forefoot, decreased flow resistance due to peripheral neuropathy, or iatrogenic injury to the sympathetic nerve (Fig. 7.39).
Fig. 7.39 A patient after resection of a tumor near the sympathetic trunk. (a) Hyperemia of the left foot with local warmth and redness. (b) Normal triphasic frequency spectrum from the right posterior tibial artery. (c) Monophasic waveform with an increased flow volume (2 vs. 26 mL/minute) after iatrogenic sympathectomy. The patient had been referred for investigation of a possible arteriovenous (AV) fistula in the left foot.
Pathologic Findings
Peripheral Arterial Occlusive Disease
PAOD is a hitherto underdiagnosed disease whose prognostic significance for life expectancy is usually underestimated. As a marker disease, it not only affects the diseased extremity but is also distinguished by a high cardiovascular mortality, even more than the history of coronary heart disease.
▶ Frequency. According to an ankle-brachial pressure index (ABI) study of patients seen by private practitioners, the prevalence of PAOD is 19.8% and 16.8%, respectively, in men and women ≥ 65 years, increasing to 27.9% and 29.2% by 85 years of age.17 When the infrapopliteal arteries are included, the prevalence in a study of 6,880 patients is approximately doubled to 34% and 34.8%, respectively. These figures do not include patients with asymptomatic, hemodynamically insignificant PAOD not detectable by changes in the ABI.22 These cases are detectable only by color duplex sonography.71
As Fig. 7.40 indicates, the frequency distribution of PAOD is influenced not only by age but also particularly by risk factors and is a generalized vascular disease that is rarely confined to a circumscribed region.18
Fig. 7.40 Effect of risk factors on the distribution pattern of peripheral arterial occlusive disease (PAOD) in 2,659 symptomatic patients managed by interventional therapy. (Adapted with permission from Diehm et al.18)
▶ Sites of occurrence. The classification of lower extremity PAOD by anatomic regions is based on the subtypes proposed by Ratschow. Three different affected regions are distinguished:
•Pelvic level (pelvic type)
•Thigh level (thigh type)
•Crural and pedal arteries (peripheral type)
As a general rule of thumb, the complaints associated with PAOD always occur distal to the vascular obstruction. Thus, a patient with an occlusion at the pelvic level may experience significant claudication in the calf in addition to exercise-dependent pain in the gluteal or thigh muscles. The nature, extent, and location of the typically exercise-dependent complaints depend on the collateral blood supply and thus on the patient’s level of conditioning.
In our experience, approximately one-fourth of patients have multilevel involvement, or the presence of obliterative lesions at multiple pelvic levels, at the time of initial diagnosis. It is common to find bilateral changes which, interestingly, will often show a symmetrical distribution. This underscores the importance of a complete, proximal-to-distal vascular examination that includes scanning of the opposite leg, as the reported complaints may be limited to one side.
▶ Staging. According to the Kirchhoff’s laws, the resistances produced by steno-occlusive lesions in multilevel disease are additive and explain the severity of the disease, which is staged by the Fontaine and Rutherford criteria shown in Table 7.8.
Duplex Sonographic Criteria
Hemodynamic Principles of Stenosis Detection
As described above, the triphasic waveform with a narrow frequency band remains intact out to the peripheral level. Only the amplitudes diminish distally because flow velocities decrease along multiple in-line tubes (Fig. 7.38). Meanwhile the frequency spectrum is subject to a number of influences that can compound the difficulty of spectral analysis:
•Cardiac pumping function (delayed systolic peak and amplitude in heart failure)
•Aortic valve function (delayed systolic peak due to aortic stenosis, or increased systolic and diastolic amplitudes with continuous negative diastolic reverse flow due to aortic insufficiency)
•Functional competence of the aortic windkessel (expansion chamber)
•Course of the vessel (spectral broadening and amplitude changes due to tortuosity)
•Vascular branching
•Degree of collateralization
•Peripheral vascular resistance
∘Decreased due to peripheral inflammation, polyneuropathy, previous exercise, warm extremity, AV shunts
∘Increased due to a cold extremity, elevated tissue pressure as in a compartment syndrome, vasospasm
Direct and Indirect Stenosis Criteria
The accuracy of many duplex ultrasound studies in determining the extent and location of vascular obstructions, including comparisons with other imaging modalities, does not always correspond to the experience in angiologic practice because the study methodology is often reduced to an analysis of single flow parameters. The hemodynamic significance and clinical relevance of a vascular obstruction can be accurately assessed based on a correlation of clinical findings, vascular status, and sonographically acquired direct and indirect stenosis criteria.
▶ Intrastenotic flow changes. These are the direct stenosis criteria listed in Table 7.9.
Table 7.9 Intrastenotic flow changes as direct stenosis criteria (Based on Jäger et al27)
Degree of stenosis (% diameter reduction) | Flow changes |
Normal | •Triphasic Doppler waveform with a narrow frequency band •Diastolic forward flow decreases with ageing until only diastolic reverse flow remains |
< 25 | •Preservation of triphasic waveform •Possible slight spectral broadening •No change in pre-, intra-, or poststenotic flow velocities |
25–50 | •Increased flow velocity •Bi- or triphasic waveform •Further spectral broadening •No change in pre-, intra-, or poststenotic flow velocities |
50–75 | •Peak systolic velocity in the stenosis increases by more than 100% and thus by a factor > 2, usually much more than 2. •While the prestenotic spectrum may show little, if any, change, the distal spectrum will usually show a diminished, monophasic waveform. |
75–99 | •Peak systolic velocity is increased manyfold. •In a very high-grade stenosis, flow is decreased due to friction. •Variable intrastenotic diastolic flow (from flat forward flow to flat reverse flow, Fig. 7.41) •Prestenotic reduction of peak systolic velocity with a normal slope and decreased diastolic reverse flow •Poststenotic reduction of peak systolic velocity and slope (= flattened waveform) with absence of a diastolic component (= monophasic flow) |
Fig. 7.41 Holodiastolic reverse flow (arrow) in a high-grade stenosis of the superficial femoral artery. (a) Pulsed-wave (PW) Doppler. (b) Color Doppler.
Based on a duplex study of arterial segments from the iliac to popliteal level, < 50% stenosis can be distinguished from > 50% stenosis with a sensitivity and specificity of 77% and 98%, respectively, which matched the angiographic grading of stenosis by two independent radiologists.28 If we consider only a peak systolic velocity (PSV) of > 180 cm/s for > 50% stenosis, the sensitivity and specificity are reduced to 66% and 80%, respectively.45 This is explained by a reduction of flow velocities across multiple stenoses. But when we take the ratio of intrastenotic PSV to prestenotic PSV (the peak velocity ratio, PVR), we obtain a quantitative measure for evaluating the degree of stenosis.45 This increases the sensitivity and specificity for the detection of > 50% stenosis to 87%–91% and 91%–98%, respectively (Fig. 7.42, Table 7.10).45 , 46 , 70
Fig. 7.42 Incidental finding of an asymptomatic stenosis with approximate grading of the stenosis. (a) Incidental angiographic finding. (b) Velocity profile across the stenosis with a peak velocity ratio (PVR) of approximately 2. (c) Grading of the stenosis in the Ranke nomogram (approximately 40%).
If the prestenotic segment cannot be clearly visualized, the PVRdist (ratio of intra- to poststenotic PSV) can be used as a measure of stenosis, although this method has slightly lower sensitivity and specificity.45 In an angiographically controlled study of 177 lower extremities, a PVR of > 2 could detect > 50% stenosis with a sensitivity and specificity of 92% and 99% in vascular segments distal to the origin of the renal arteries.1 A poor correlation was found between the peak end-diastolic velocity and the detection of > 50% stenosis (sensitivity: 47%).45 Diastolic flow may be antegrade or retrograde, depending on peripheral runoff conditions and the resistance across the stenosis, which explains the poor accuracy of degree-of-stenosis determination based on the peak end-diastolic flow velocity alone (Fig. 7.43).
Fig. 7.43 Examples of three clinically significant > 70% stenoses of the superficial femoral artery. (a) With biphasic reverse flow. (b) With holodiastolic reverse flow. (c) With predominantly diastolic forward flow.
High-grade stenosis may also be manifested by artifacts, which appear as “musical murmurs” in pulsed-wave (PW) Doppler and form a high-frequency “seagull cry” superimposed over the Doppler spectrum (Fig. 7.37).
▶ Prestenotic flow changes. Spectral changes proximal to an obstructive lesion are indirect stenosis criteria that are seen only in association with high-grade stenosis or occlusion. The poorer the collateral circulation and the shorter the distance from the sampling site to the lesion, the more pronounced the changes. This particularly applies to an acute embolic occlusion with inadequate peripheral compensation. The decrease in volume flow leads to a reduced systolic amplitude, while the slope of the systolic upstroke usually remains unchanged. The diastolic reverse flow component is reduced and shows a biphasic or monophasic pattern. In extreme cases it may produce a “stump signal” with short, steep forward and reverse flow amplitudes (Fig. 7.17, Fig. 7.44).
Fig. 7.44 Asymptomatic occlusion of the popliteal artery in a 65-year-old athletic male. (a) Spectrum from the profunda femoris artery at its origin shows holodiastolic forward flow as evidence of increased collateral flow through the vessel. (b) Reduced systolic and diastolic flow amplitudes with a normal slope of the systolic upstroke in the middle third of the superficial femoral artery. (c) Further amplitude reduction and absent diastolic inverse peak (DIP) at the femoropopliteal junction approximately 2 cm proximal to the occlusion.
▶ Poststenotic flow changes. These changes can provide important indirect stenosis criteria. A hemodynamically significant stenosis with an angiographic diameter reduction of 50% according to intra-arterial pressure measurements should not be equated with a clinically significant stenosis.53 Besides causing no clinical symptoms in most cases, a stenosis of approximately 50% is usually not manifested by indirect pre- and poststenotic Doppler frequency parameters. On the other hand, a clinically significant stenosis of > 70% will cause a poststenotic decrease in the diastolic reverse flow component with transition from a triphasic to a bi- or monophasic waveform, a delayed systolic peak, a reduced PSV, and therefore a reduced pulsatility index (PI). The more stenoses are arranged in series, the greater the reduction in PI since the resistances add up according to the Kirchhoff’s laws. While the normal PI values range from 4.5 to 13 at different sites in the peripheral arteries (mean value = 6.7), they may be less than 1 due to the presence of multilevel occlusions. It is also true that as the peak diastolic flow more closely approaches the peak systolic flow, peripheral compensation for the upstream occlusions becomes less effective. Severe cases show a flat frequency spectrum with minimal systolic-to-diastolic velocity fluctuations (Fig. 7.45). In our experience, the presence of this waveform in patients with medial calcific sclerosis equals the prognostic value of transcutaneous oxygen measurement in the assessment of critical ischemia.57
Fig. 7.45 Flat waveform from the posterior tibial artery with an extreme flow reduction and a falsely elevated ankle-brachial index (ABI) of 0.9 in a patient with medial calcific sclerosis.
Color Doppler
Besides improving the spatial resolution of vascular imaging compared with gray scale duplex sonography, superimposing color Doppler information provides rapid, semiquantitative information on the presence of flow inhomogeneities such as local flow separation (turbulence) or stenosis-induced flow acceleration with aliasing or a color mosaic over perivascular tissue (“confetti sign,” Fig. 7.46) that may indicate high-grade luminal narrowing. The investigation of suspicious vascular segments by PW Doppler spectral analysis using the pre-, intra-, and poststenotic flow changes described above is a reliable method for evaluating the degree of stenosis. Similar to the “seagull cry” in PW Doppler, a high-grade stenosis may produce color artifacts due to perivascular tissue vibrations with a pulsatile confetti sign (Fig. 7.46).
Fig. 7.46 Series of multiple stenoses in the superficial femoral artery. (a) Angiography. (b) With duplex sonography, the stenoses are quickly revealed by the presence of aliasing (yellow arrows) and eddy currents (white arrow, confetti sign).
Occlusion
Color Doppler is essential for detecting vascular occlusion and determining its length. The criteria for this purpose are listed in Table 7.11. Errors may arise due to pronounced vessel wall calcifications or improper machine settings (e.g., PRF set too high for detecting slow postocclusive flow). Also, if the color box angle is too flat, it may lead to misinterpretation in a deeply situated vessel. The flat incidence angle causes color signals to be absent or diminished due to increased tissue reflections and a prolonged transit time, mimicking an occlusion. It is helpful in such cases to change from a linear transducer to a curved array.
Table 7.11 Color duplex criteria for vascular occlusion (Based on Karasch et al32)
Direct criteria | Indirect criteria |
•Imaging in multiple planes shows no intraluminal color signals with a low PRF, optimal insonation angle, and optimal color gain •A Doppler frequency spectrum cannot be acquired from the affected vascular segment | •Collateral vessels arising proximal to the occlusion •Postocclusive reconstitution of color flow by collateral vessels •Reduced postocclusive flow velocity with a steep upslope, monophasic or biphasic •Low postocclusive flow velocity with a flat upslope, usually monophasic |
Abbreviation: PRF, pulse repetition frequency. |
B-mode sonographic criteria may be an occlusive internal echo pattern or narrow tissue bands with irregular echoes accompanying the deep vein. The latter indicates an occlusive event that occurred many years earlier. Ultimately, a comprehensive assessment of the duplex sonographic criteria will determine the reliability of the findings.
The occlusion length may be underestimated if proximal or distal collaterals in the course of the vessel mimic a patent channel. It may be overestimated if:
•Shadowing plaques create a color void in the proximal or distal part of the occlusion or
•The severely reduced postocclusive flow velocity is no longer color-coded.
Steno-occlusive Disease of the Pelvic Arteries
Clinical Examination
Steno-occlusive arterial disease at the pelvic level may be suspected from the history (claudication of the gluteal, thigh, or calf muscles), absent or diminished femoral pulses, and auscultation at rest and after exercise. Audible bruits provide the only clinical means for differentiating high-grade stenosis from occlusion. At the same time, high-grade stenosis may not produce an audible bruit, or a stenosed collateral may mimic an iliac stenosis when an occlusion is present. In rare cases vasogenic impotence or claudication complaints in the gluteal muscles may be caused by an internal iliac occlusion that is obscured clinically due to equal femoral pulses.
Although the specific arteries that collateralize a flow obstruction can vary considerably in different individuals, the most commonly recruited pathways are shown in Fig. 7.47. With a unilateral occlusion of the common iliac artery, cross-connections will often develop between branches of the right and left internal iliac arteries. This explains why occlusive lesions of the iliac bifurcation and the origin of the inferior mesenteric artery, which block the connection between the two principal collateral pathways, cause much greater ischemic complaints than occlusions located below those levels.
Fig. 7.47 Potential collateral pathways associated with aortic, aortoiliac, and femoral occlusions (diagrammatic representation): mesenteric epigastric, lumbar, iliofemoral, and ilioprofundal. IMA, inferior mesenteric artery.
Duplex Sonography
▶ Qualitative measurements. Because indirect stenosis criteria in the lower extremities are easy to determine, even for less experienced operators, and supply reliable information on stenosis at the aortoiliac level, we start the examination by sampling a Doppler spectrum from the common femoral artery and proceed upward. While < 50% stenosis does not alter the spectral waveform, proximal stenosis of 50% to 70% can cause a reduction of the reverse flow component (DIP) in the common femoral artery. This waveform is not qualitatively different from a condition of aortoiliac patency with distal femoral artery occlusion. Stenosis must exceed 70% in order to produce diminished amplitudes with a delayed systolic peak and a decreased PI. The normal PI is approximately 5 (8.5 ± 3.5) and may fall to 1 in patients with a poorly compensated occlusion. According to an older study, a femoral PI cutoff value of 4 had 95% sensitivity and 82% specificity for detecting isolated aortoiliac obstructions with corresponding values of 99% and 45% for two-level occlusions.62
▶ Common femoral artery velocity waveforms. In another study, duplex ultrasound velocity waveforms recorded from the common femoral artery were classified into four patterns: triphasic, biphasic, sharp monophasic, and poor (flat) monophasic. The findings were then compared with MRA to determine their accuracy for detecting aortoiliac obstructive disease. The presence of a poor monophasic waveform had 56% sensitivity and 97% specificity for detecting high-grade iliac stenosis. A full one-fourth of cases with significant aortoiliac stenosis presented a triphasic waveform.52
But when the duplex ultrasound findings (monophasic and biphasic) in the common femoral artery were compared with the results of pelvic angiography, they were found to have a sensitivity and specificity of 95% and 89%, respectively, for detecting significant aortoiliac occlusive disease.47 When a common femoral artery PSV of 45 cm/s or less is included in the analysis, the sensitivity and specificity of the monophasic waveform are, respectively, increased from 89% and 75% to 97% and 92% (Fig. 7.48).47
Fig. 7.48 Duplex findings in a 58-year-old heavy smoker with claudication symptoms in both calves, spreading later to the thighs and buttocks. (a) Color Doppler demonstrates an infrarenal occlusion of the aorta (3.7 cm long). Collateral flow is noted through a stenosed inferior mesenteric artery (IMA) with associated vortices and through posterior lumbar arteries. (b) Monophasic waveform from the right common femoral artery (CFA) with a vmax of 40 cm/s. (c) Monophasic waveform from the left CFA with a vmax of 40 cm/s.
The hemodynamic effects of an obstructive lesion of the internal iliac artery with gluteal claudication can also be qualitatively assessed based on velocity waveforms from the superior and inferior gluteal arteries. As Fig. 7.49 shows, a monophasic waveform is recorded from the terminal gluteal branches of the internal iliac artery. A biphasic or triphasic waveform excludes claudication due to circulatory insufficiency and would warrant interdisciplinary tests.
Fig. 7.49 Stenosis of the internal iliac artery in a 75-year-old man who complained for years of pain in the left buttock when walking uphill. (a) Sonographic examination in the prone position with ultrasound probe in the middle of the buttock. (b) Filiform stenosis of the internal iliac artery (vmax > 4 m/s). (c) Waveform from the inferior gluteal artery is monophasic with greatly reduced velocities. (d) Angiographic view of the stenosis before interventional therapy.
▶ Quantitative measurements. Usually, the iliac artery can be visualized as far as the aortic bifurcation, even in obese patients. Because the transducer pressure may cause discomfort, the examination should be performed with the bladder empty. This is essential for a qualitative evaluation of the pelvic arteries, which involves determining the PSV and the ratio of peak velocities within the stenosis and 2 cm proximal (or distal) to the stenosis (PVR). Cutoff values for > 50% stenosis have been determined with the aid of receiver operating characteristic (ROC) curves (Table 7.12).48 These ROC curves are constructed by plotting the sensitivity of the test against its specificity for various cutoff values.
Table 7.12 Quantification of stenosis based on ROC curves
Degree of stenosis (% diameter reduction) | vmax (cm/s) | Ratio |
> 50 | 120 | 1.4 |
> 70 | 160 | 2.0 |
> 90 | 180 | 2,9 |
Abbreviation: ROC, receiver operating characteristic. |
As a general rule, the value for the optimal relationship between sensitivity and specificity is called the best test value.61 Nevertheless, the values reported by Sacks et al48 are lower than in most other studies, which determined a cutoff value of 180 cm/s for PSV and a ratio of 2.5 for > 50% stenosis. These values are in good agreement with other studies,64 which found an in vivo PVR value of 2.67 for > 50% stenosis.
In another study of 112 aortoiliac segments,16 various Doppler waveform parameters (PSV, PSV ratio, PSV difference, and end-diastolic flow velocity) were compared with invasive pressure measurements and stenosis cross-sectional area reduction based on angiograms. A PSV cutoff ratio of 2.8 achieved the best result for the detection of > 50% aortoiliac stenosis with 86% sensitivity and 84% specificity. A PSV ratio of 5 achieved lower sensitivity (65%) but higher specificity (91%) for the detection of angiographically determined stenosis of > 75%.39
In cases of borderline iliac artery stenosis, the degree of stenosis can be further quantified by exercise testing.9 A velocity increase of > 140 cm/s (intrastenotic/prestenotic) provoked by exercise had a sensitivity and specificity of 93% and 87%, respectively, for detecting hemodynamically significant stenosis. This underscores the importance of all available flow parameters in evaluating stenosis, especially in patients who are difficult to examine or have equivocal findings. The examination result should never stand alone and clinical correlation must be performed to be meaningful. Due to instances of imprecise patient claims at our outpatient unit, it is our practice to take the patient on a “test walk” at an individualized pace.
Occlusion
Duplex sonography has become an increasingly important tool for evaluating collateral circulation in response to iliac artery occlusions. For example, it may demonstrate retrograde flow through the internal iliac artery to the external iliac artery in patients with an occluded common iliac artery (Fig. 7.50a). Similarly, retrograde flow (i.e., toward the transducer) may be detected in the profunda femoris artery due to an occlusion at the junction of the external iliac artery and common femoral artery. This occlusion is collateralized from branches of the internal iliac artery that gain attachment to the lateral or medial circumflex femoral artery, producing retrograde flow in the profunda femoris artery (Fig. 7.50b).
Fig. 7.50 Profunda femoris: A 57-year-old man with an occlusion of the distal aorta and right common iliac artery (CIA). (a) Retrograde flow in the internal iliac artery (IIA) and monophasic flow in the external iliac artery (EIA). (b) Left-sided occlusion of the iliac and common femoral artery (CFA) with retrograde collateral flow in the profunda femoris artery (PFA) and collateralization of the superficial femoral artery (SFA).
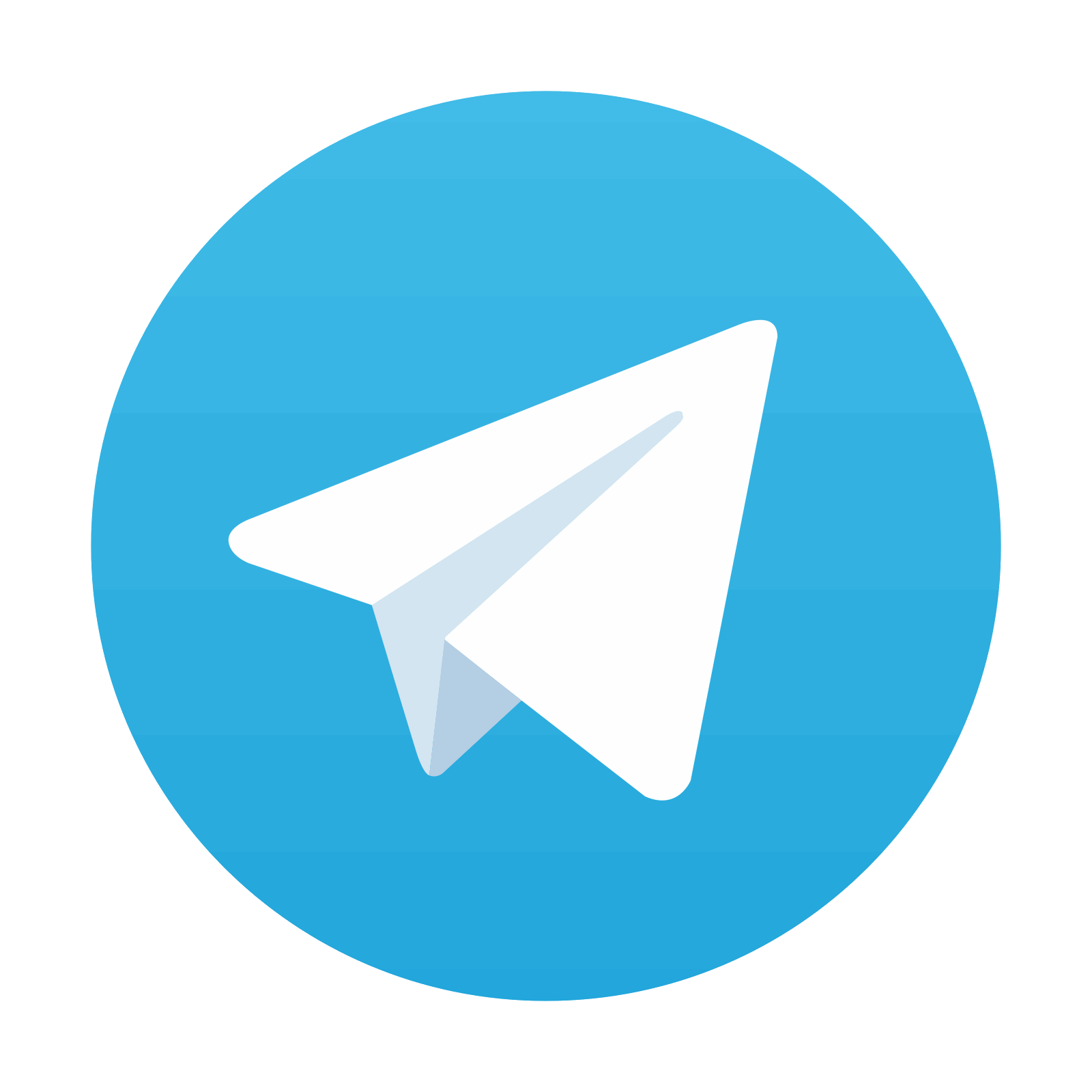
Stay updated, free articles. Join our Telegram channel

Full access? Get Clinical Tree
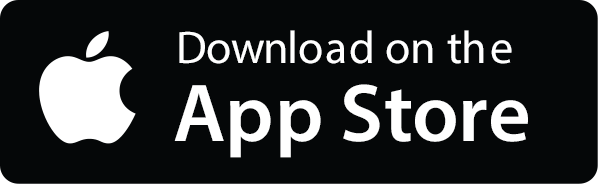
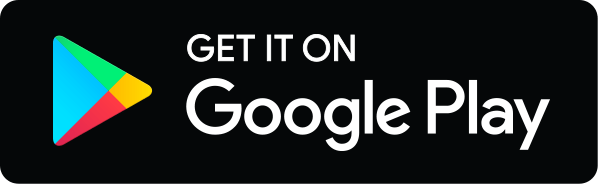